1 Introduction
Hydroxychromene and its derivatives are widespread in nature [1,2]. They have been widely employed as valuable intermediates in the synthesis of several complex synthetic and natural products [3]. Synthesis of butenolide derivatives from the chromenes via a three–component reaction was reported by Balalai et al. [4]. These compounds have attracted considerable attention due to their unique biological and pharmacological activities [5–7]. Many biological properties [8–11] and antifungal activities [12] were reported for the chromenes. Different synthetic strategies have been reported for the synthesis of hydroxychromenes via the reactions of ortho-hydroxy aromatic aldehydes and dialkyl acetylenedicarboxylates [13,14]. Ramazani et al. reported this reaction in the presence of silica gel under solvent-free conditions at 90 °C. They isolated and identified two products with an approximately equal ratio and identified the desired product as 4-hydroxy-4H-chromenes albeit as an unstable compound [15]. 4-Hydroxychromene derivatives were also prepared via the reactions of diethyl acetylenedicarboxylate with salicyl N-tosylimines or salicylaldehydes in the presence of DABCO or dimethylphenylphosphine under mild conditions in excellent yields [16]. Recently, Yoshioka et al. reported the synthesis of 2-hydroxychromenes via the reaction of salicylaldehydes with dienophiles in the presence of CsF [17].
Using conventional organic solvents especially chlorinated hydrocarbons poses a serious threat to the environment because of their toxicity and frequent volatile nature [18]. Nowadays, scientists and especially synthetic organic chemists are obliged to themselves to follow and develop green chemistry disciplines in practical organic chemistry in order the principles of sustainable chemistry in order the criteria and principles of sustainable, environmentally benign chemistry [19]. Consequently, reactions which have a chance of being conducted under solvent-free conditions can be categorized as green [20]. These reactions have attracted tremendous attention both in academia and industry [21–24].
Heterogeneous catalysis is considered as attractive and superior in many areas of synthetic organic chemistry due to exhibiting unprecedented qualities such as ease of separation and reusability [25,26]. Heterogeneous catalysis is also important in many areas of the chemical and energy consuming industries [27]. In heterogeneous catalysis, the quality and extent of the surface area of the catalyst are vital. One of the best choices for maximizing the surface area is using silica [28]. Due to our interest in application of heterogeneous catalysts and use of MWI in organic reactions [26, 29–34], we wish to reveal here our achievements to develop the synthesis of hydroxychromenes via the reaction of salicylaldehydes and dialkyl acetylenedicarboxylates mediated by imidazole-functionalized silica nanoparticles [35] as an efficient and recyclable solid base catalyst under MWI (Scheme 1).
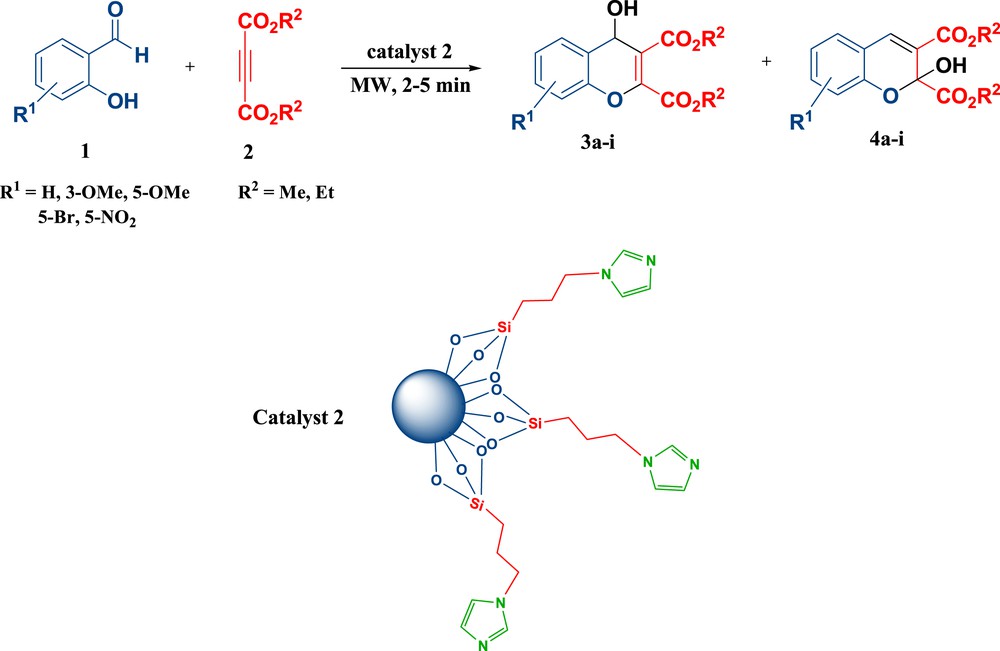
Microwave-assisted synthesis of chromenes using an imidazole based solid catalyst.
2 Results and discussion
The reaction between salicylaldehyde and DMAD was chosen as a model reaction. The reaction conditions were deliberately chosen being very mild initially just by refluxing the reactants in CH2Cl2 and purposely no catalyst was employed in order to achieve a non-catalyzed reaction. The progress of the reaction was monitored by TLC (using EtOAc/n-hexane 1:4 as an eluent). The reaction was preceded sluggishly and the reaction was completed in 3 h. In other trials, the reaction was examined using imidazole-functionalized silica nanoparticles [35] as a catalyst under MWI for possible synthesis of the corresponding hydroxychromenes. After a conventional work up, the purity of crude was tested by TLC (using EtOAc/n-hexane 1:4 as an eluent) and no trace of starting materials was detected. However, two separable spots were observed. They were separated and identified as dimethyl 4-hydroxy-4H-chromene-2,3-dicarboxylate (3a) and dimethyl 2-hydroxy-2H-chromene-2,3-dicarboxylate (4a) in 16% and 23% yields, respectively (entry 1, Table 1). Other solvents were examined in this procedure and no noticeable improvement was observed in the time of the reaction and yields of the products (entries 2–4, Table 1).
Microwave-assisted reaction of salicylaldehyde (1 mmol) with DMAD (1 mmol) under different conditions.
Entry | Solvent | Temperature (°C) | Catalyst (mol%) | Heating | Time (min) | Product 3a Yielda (%) | Product 4a Yielda (%) |
1 | CH2Cl2 | Reflux | – | Conventional | 180 | 16 | 23 |
2 | DMF | 70 | – | Conventional | 180 | 18 | 26 |
3 | EtOH | 70 | – | Conventional | 180 | 14 | 20 |
4 | CH3CN | 70 | – | Conventional | 180 | 17 | 22 |
5 | – | 60 | – | MW | 5 | 19 | 30 |
6 | – | 60 | Catalyst 2 (0.27) | MW | 2 | 39 | 45 |
6 | – | 60 | Catalyst 2 (0.45) | MW | 2 | 40 | 48 |
7 | – | 60 | Catalyst 2 (0.72) | MW | 2 | 42 | 52 |
8 | – | 60 | Catalyst 2 (0.9) | MW | 2 | 42 | 52 |
9 | – | 60 | Catalyst 1 (8) | MW | 2 | 27 | 38 |
10 | – | 60 | Catalyst 1 (13) | MW | 2 | 31 | 42 |
11 | – | 60 | Catalyst 1 (17) | MW | 2 | 35 | 47 |
12 | – | 60 | Catalyst 1 (22) | MW | 2 | 35 | 47 |
13 | – | 80 | Catalyst 1 (17) | MW | 2 | 30 | 45 |
14 | – | 60 | Nano-SiO2 (5)b | MW | 10 | 18 | 28 |
15 | – | 80 | Nano-SiO2 (10)b | MW | 10 | 19 | 30 |
16 | – | 60 | CPS-nanoSiO2 (5)b | MW | 10 | 17 | 18 |
17 | – | 80 | CPS-nanoSiO2 (10)b | MW | 10 | 15 | 25 |
18 | – | 60 | ZnO (20) | MW | 100 | 37 | 30 |
19 | – | 60 | MgO (20) | MW | 100 | 40 | 28 |
20 | – | 60 | K2CO3 (20) | MW | 180 | 30 | 25 |
21 | – | 60 | TiCl4 (20) | MW | 120 | 35 | 37 |
a Isolated yields.
b The values in mg.
We have been engaged with the development of various organic conversions, by performing them under MWI in order to accelerate the reactions and improving their yields. Armed with these experiences, the typical reaction was examined under MWI and solvent-free conditions and found actually promising to pursue. Interestingly, the reaction was completed in very short time (2 min) in higher yields (entry 5, Table 1). To go further, we decided to perform the reaction catalytically. We decided to examine the catalytic potential of imidazole (catalyst 1) [36,37] and freshly prepared a heterogeneous imidazole-functionalized nano-silica catalyst (catalyst 2) [35]. Excellent yields of products were obtained in the presence of catalysts 1 and 2 under MWI solvent-free conditions (entries 7, 11, Table 1). Furthermore, it was found that catalyst 2 is more effective than catalyst 1 (Table 1). It was also found that the amount of catalyst has an appreciable effect on the yields of the product. On increasing the amount of catalyst from 0.27 to 0.72 mol% the yield increased. However, more increase in the amount of the catalyst did not show any appreciable increase in the yield of the product. Additionally, the catalytic activity of silica nanoparticles and 3-chloropropyltrimethoxysilane functionalized silica nanoparticles (CPS-nanoSiO2) was investigated for the pilot reaction (entries 14–17, Table 1). Also, we screened the catalytic activity of various catalysts such as ZnO, MgO, K2CO3 and TiCl4 for the pilot experiment and the results are gathered in Table 1. These results obviously showed that the catalyst 2 is the best choice for this reaction. Eventually, we concluded that the best yield of the products can be obtained using 1/1 M ratios of salicylaldehyde/DMAD in the presence of catalyst 2 (0.72 mol%) (Table 1). The recyclability and stability of catalyst 2 were investigated in the reaction of salicylaldehyde and dimethyl acetylenedicarboxylate under the optimized reaction conditions (Table 2). Upon completion of the reaction, catalyst 2 was recovered virtually quantitatively from the reaction mixture by simple filtration and washing three times with ethyl acetate. This recovered catalyst was used at least for five consecutive runs under optimal conditions without appreciable loss of catalytic activity. In addition, the result of thermogravimetric analysis (TGA) showed that imidazole-functionalized silica nanoparticles (Im-nanoSiO2) could endure about 244 °C with little loss of their weight. The decomposition of Im-nanoSiO2 started from about 533 °C confirming the high thermal stability of the catalyst 2 as an advantage (Fig. 1).
The recyclability of catalyst 2 in the synthesis of 3a and 4a.
Run | Product 3a Yield (%)a | Product 4a Yield (%)a |
1 | 42 | 52 |
2 | 42 | 52 |
3 | 41 | 50 |
4 | 40 | 49 |
5 | 38 | 49 |
a Isolated yield.

Thermogravimetric curve of Im-nanoSiO2.
Furthermore, in order to establish the generality of this method the scope of this protocol was extended by employing a wide variety of salicylaldehydes which are subjected to the same reaction under optimal conditions. The obtained experimental results are listed in Table 3. To go further, diethyl acetylenedicarboxylate instead of DMAD was also reacted with some selected salicylaldehyde derivatives under concluded optimal conditions. Predictably, no significant difference in the reaction times and yields of products was observed. Salicylaldehydes bearing electron-withdrawing substituents afforded to the corresponding adducts in higher yields in comparison with those bearing electron-donating groups (Table 3).
Synthesis of hydroxychromenes via the reaction of salicylaldehyde and di-(methyl or ethyl) acethylenedicarboxylate using imidazole-functionalized silica nanoparticles.
Entry | R1 | R2 | Reaction time (min) | Product | |
3 (Yielda %) | 4 Yielda (%) | ||||
1 | H | Me | 2 | 3a (42) | 4a (52) |
2 | 3-OMe | Me | 3.5 | 3b (34) | 4b (46) |
3 | 5-OMe | Me | 3.5 | 3c (32) | – |
4 | 5-NO2 | Me | 1.5 | 3d (44) | – |
5 | 5-Br | Me | 2 | 3e (44) | 4e (53) |
6 | 3-OMe | Et | 3.5 | 3f (30) | – |
7 | 5-NO2 | Et | 2 | 3g (39) | – |
8 | 5-Br | Et | 2 | 3i (37) | 4i (47) |
a Isolated yields.
A possible mechanism for the formation of products 4 and 3 in the presence of Im-nanoSiO2 is shown in Scheme 2. At first, Im-nanoSiO2 activates the OH group of salicylaldehyde to increase its nucleophilic attack on dialkyl acetylenedicarboxylates. Subsequently, intramolecular cyclization results in the intermediate I. Reactive intermediate II is formed by dehydration of intermediate I. Then water can attack II by two pathways (A and B). The products 4 and 3 result from paths A and B, respectively.

The possible mechanism.
3 Conclusion
In summary, we have developed a catalyzed MW-assisted reaction leading to a wide range of hydroxychromenes, starting from simple and readily available starting materials. This approach shows merits and advantages such as using the recyclable heterogeneous catalyst and the MWI as a green source of energy combined with a solvent-free system. It places the method among the environmentally benign reactions for the facile and efficient operational approach for the synthesis of hydroxychromene derivatives.
4 Experimental
4.1 General information
All reagents were purchased from Merck and used without further purification. Infrared spectra and FT-IR spectra were recorded on a PerkinElmer using KBr discs. 1H NMR spectra were recorded on a Bruker Avance AC-300 MHz (or 250 MHz) Spectrometer and 13C NMR spectra at 75.4 MHz (or 62.5 MHz) on the aforementioned instrument using CDCl3 as the deuterated solvent and TMS as an internal standard. MW experiments were conducted in a Milestone MicroSynth apparatus. All melting points are measured in open glass-capillaries using Stuart melting point apparatus. FE-SEM images were recorded by using a Hitachi, model S- 4160. Mass spectra, using electron ionization (EI)-mass spectrometry (MS), were recorded on a Shimadzu GCMS-QP-2000A mass spectrometer. Elemental analyses were conducted using a Perkin–Elmer 240C elemental analyzer; their results were found to be in good agreement with the calculated values. Thermogravimetric analysis (TGA) of the synthesized catalyst was performed on a TGA/SDTA 815e (METTLER/TOLEDO) over a 50–950 °C range at a heating rate of 10 °C/min, under a nitrogen flow.
4.2 Synthesis of catalyst 2
The freshly prepared silica nanoparticle suspension [30] was precipitated with n-hexane and extracted through centrifugation (twice at 6000 rpm). Then, silica (1.02 g) was again suspended in CH2Cl2 (5 ml) and the solution of 3-chloropropyltrimethoxysilane (198 mg, 1 mmol) in CH2Cl2 was added. The mixture was stirred for 3 days at 50 °C. In the following step, the solvent and the methanol created in the grafting step were distilled off and the remaining solid was dried under reduced pressure and the excess of 3-chloropropyltrimethoxysilane was removed by extraction with dichloromethane. The crude solid was poured into a flask containing toluene, triethylamine (0.14 ml, 1 mmol), and imidazole (68 mg, 1 mmol). The mixture was stirred at reflux temperature for 48 h [35]. After completion of the reaction, the solvent was distilled off under vacuum pressure and washed three times with ethyl acetate. The residue was dried under high vacuum to afford the catalyst 2 (Scheme 3).

Synthesis of imidazole-functionalized silica nanoparticles (Catalyst 2).
The SEM image of the freshly prepared catalyst was obtained and compared with the SEM image of silica nanoparticles. Comparing the SEM image of silica nanoparticles with that of Im-nanoSiO2 showed that the mean size of Im-nanoSiO2 is larger than that of silica nanoparticles (Figs. 2 and 3).
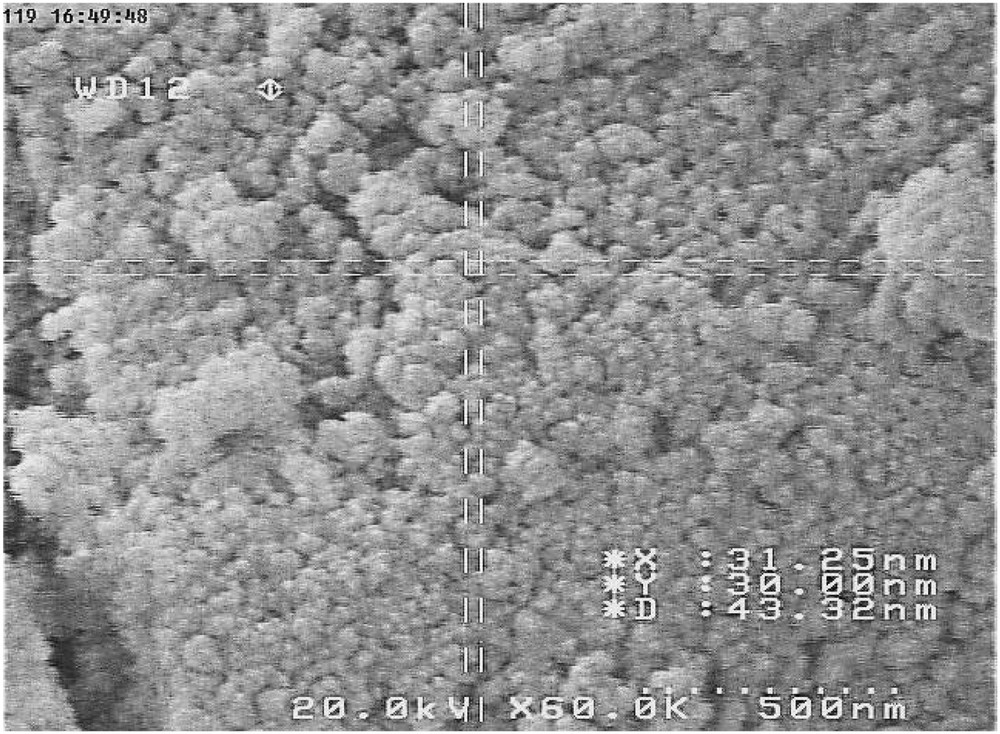
The SEM image of silica nanoparticles.

The SEM image of imidazole-functionalized silica nanoparticles.
4.2.1 FT-IR analysis of catalyst 2
Fig. 4 shows the FT-IR spectrum of silica nanoparticles, CPS-nanoSiO2 and Im-nanoSiO2. The Si–O–Si vibration appeared at 1079.74 cm−1 for silica nanoparticles. This vibration was observed to have shifted to higher frequencies in CPS-nanoSiO2 and Im-nanoSiO2 since the chemical changes take place adjacent to the Si–O–Si framework by immobilization of 3-chloropropyltrimethoxysilane and imidazole. The broad band observed around 3439.56 cm−1 is related to OH vibration of Si–OH and absorbed water. The appearance of bands due to imine, CN stretching vibration (1640.00 cm−1) and C–N (1515.42 cm−1) confirms that the incorporation of the imidazole molecule with the silica nanoparticles exists [35].
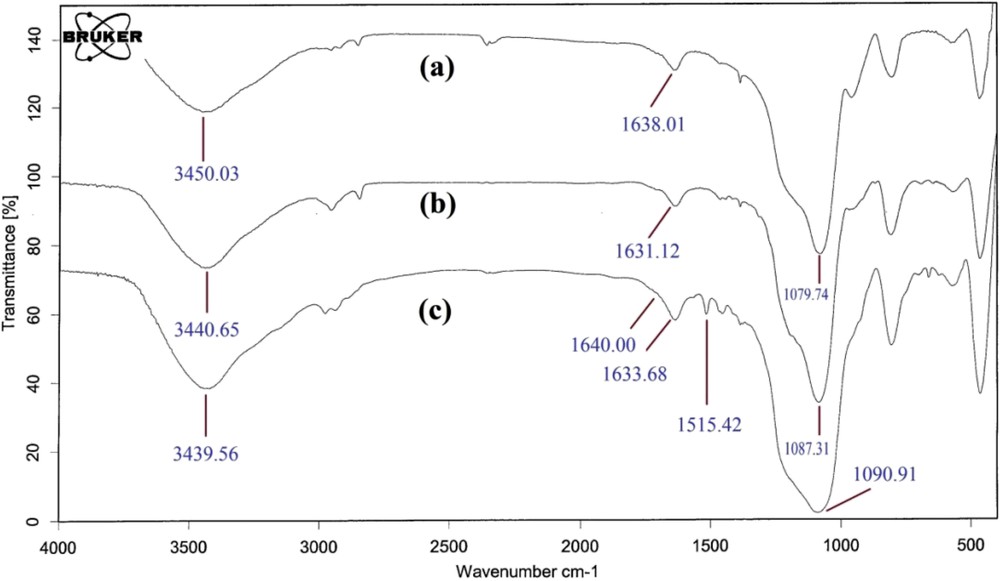
The FT-IR spectra of a) silica nanoparticles, b) 3-chloropropyltrimethoxysilane functionalized silica nanoparticles (CPS-nanoSiO2), and c) imidazole-functionalized silica nanoparticles (Im-nanoSiO2).
4.2.2 Interpretation of thermogravimetric analysis (TGA) of catalyst 2
Thermogravimetric analysis (TGA) of catalyst 2 showed 9.8% weight loss of Im-nanoSiO2 with the decomposition of all organic structures at 533 °C. 3-(1-Imidazole)propyl group loading was calculated to be 0.9 mmol/g using TGA data.
4.3 Synthesis of hydroxychromenes: general procedure
An appropriate salicylaldehyde (1 mmol), dialkyl acetylenedicarboxylate (1 mmol) and catalyst 2 (0.72 mol%) were mixed thoroughly with stirring. The mixture was subjected to MWI at 250 W and at 60 °C for a few minutes (depending on the reactants, see Table 3). The completion of the reaction was confirmed by TLC using EtOAc/n-hexane 1:4 as an eluent. After completion of the reaction, the mixture was cooled to room temperature and extracted with ethyl acetate. The extracts were concentrated under reduced pressure. The crude was directly subjected to silica gel (Merck 230–240 mesh) column chromatography using a 1:5 ethyl acetate/n-hexane mixture as an eluent to give pure products which were confirmed by their spectroscopic data (1H NMR, 13C NMR, IR, MS and elemental analysis).
4.4 Selected spectroscopic data
4.4.1 Dimethyl 4-hydroxy-4H-chromene-2,3-dicarboxylate (3a, C13H12O6)
White solid; mp 109.6–110.6 °C; IR (KBr) (νmax/cm−1): 3464.5 (OH stretching), 3019.0 (aromatic CH stretching), 1729.0, 1705.0 (CO stretching), 1671.0 (CC stretching); 1H NMR (300 MHz, CDCl3): δ 7.53 (d, 1H, J = 6.0 Hz, ArH), 7.36 (m, 1H, ArH), 7.25 (m, 1H, ArH), 7.14 (d, 1H, J = 6.0 Hz, ArH), 5.73(d, 1H, J = 3.0 Hz, CHOH), 3.94 (s, 3H, OCH3), 3.85 (s, 3H, OCH3), 3.05 (d, 1H, J = 3.0 Hz, OH) ppm; 13C NMR (75.4 MHz, CDCl3): δ 166.1, 162.7, 150.1, 149.0, 129.9, 129.8, 125.7, 120.8, 116.7, 107.6, 60.3, 53.2, 52.5 ppm; MS: m/z 264 (M+). Anal. Calcd for C13H12O6: C, 59.09; H, 4.58; O, 36.33. Found: C, 59.10; H, 4.38; O.
4.4.2 Dimethyl 4-hydroxy-8-methoxy-4H-chromene-2,3-dicarboxylate (3b, C14H14O7)
White solid; mp 128–129.6 °C; IR (KBr) (νmax/cm−1): 3494.8 (OH stretching), 3003.7 (aromatic CH stretching), 1742.5, 1702.8 (CO stretching), 1650.6 (CC stretching); 1H NMR (300 MHz, CDC13): δ 7.19 (m, 2H, ArH), 6.92(dd, 1H, J = 9.0, 1.8 Hz, ArH), 5.75 (1H, d, J = 6.0 Hz, CHOH), 3.96 (s, 3H, OCH3), 3.89 (s, 3H, OCH3), 3.86 (s, 3H, OCH3), 2.94 (d, 1H, J = 6.0 Hz, OH) ppm; 13C NMR (100 MHz, CDCl3): δ 165.1, 161.5, 148.7, 146.7, 138.0, 124.4, 121.2, 120.8, 110.6, 106.9, 59.4, 55.2, 52.2, 51.5 ppm; MS: m/z = 294 (M+). Anal. Calcd for C14H14O7: C, 57.14; H, 4.80; O, 38.06. Found: C, 57.04; H, 4.74; O, 38.00.
4.4.3 Dimethyl 4-hydroxy-6-methoxy-4H-chromene-2,3-dicarboxylate (3c, C14H14O7)
White solid; mp 129–129.6 °C; IR (KBr) (νmax/cm−1): 3484.7 (OH stretching), 3007.8 (aromatic CH stretching), 1731.0, 1698.3 (CO stretching), 1661.0 (CC stretching); 1H NMR (300 MHz, CDC13): δ 7.07 (d, 1H, J = 9.0 Hz, ArH), 6.99 (d, 1H, J = 3.0 Hz, ArH), 6.90 (dd, 1H, J = 9.0, 3.0 Hz, ArH), 5.72 (d, 1H, J = 6.0 Hz, CHOH), 3.94 (s, 3H, OCH3), 3.85 (s, 3H, OCH3), 3.82 (s, 3H, OCH3), 3.12 (d, 1H, J = 6.0 Hz, OH) ppm; 13C NMR (75.4 MHz, CDCl3): δ 166.4, 162.9, 157.1, 150.5, 143.2, 121.4, 117.9, 117.1, 112.5, 106.5, 60.9, 55.8, 53.3, 52.6 ppm; MS: m/z = 294 (M+). Anal. Calcd for C14H14O7: C, 57.14;H, 4.80; O, 38.06. Found: C, 57.20; H, 4.68; O, 38.06.
4.4.4 Dimethyl 4-hydroxy-6-nitro-4H-chromene-2,3-dicarboxylate (3d, C13H11NO8)
White solid; mp 170.8–172.5 °C; IR (KBr) (νmax/cm−1): 3431.6 (OH stretching), 3080 (aromatic CH stretching), 1738.0, 1700.9 (CO stretching), 1665.0 (CC stretching); 1H NMR (300 MHz, CDC13): δ 8.51 (d, 1H, J = 3.0 Hz, ArH), 8.25 (dd, 1H, J = 9.0, 3.0 Hz, ArH), 7.28 (d, 1H, J = 9.0 Hz, ArH), 5.80 (d, 1H, J = 6.0 Hz, CHOH), 3.98 (s, 3H, OCH3), 3.90 (s, 3H, OCH3), 3.35 (d, 1H, J = 6.0 Hz, OH) ppm; 13C NMR (100 MHz, CDCl3): δ 164.4, 160.9, 151.9, 148.5, 143.9, 125.5, 124.2120.8, 117.0, 107.6, 58.9, 52.4, 51.9 ppm; MS: m/z = 309 (M+). Anal. Calcd for C13H11NO8: C, 50.49; H, 3.39; O, 41.30; N, 4.53. Found: C, 50.44; H, 3.52; O, 41.15; N, 4.48.
4.4.5 Dimethyl 6-bromo-4-hydroxy-4H-chromene-2,3-dicarboxylate (3e, C13H11BrO6)
White solid; mp 93.5–94.6 °C; IR: 3460.8 (OH stretching), 3015.8 (aromatic CH stretching), 1738.0, 1700.99 (CO stretching), 1665.0 (CC stretching); 1H NMR (300 MHz, CDC13): δ 7.69 (d, 1H, J = 3.0 Hz, ArH), 7.47 (dd, 1H, J = 9.0, 3.0 Hz, ArH), 7.04 (d, 1H, J = 9.0 Hz, ArH), 5.71 (d, 1H, J = 6.0 Hz, CHOH), 3.90 (s, 3H, OCH3), 3.80 (s, 3H, OCH3), 3.12 (s, 1H, J = 6.0 Hz, OH) ppm; 13C NMR (75.4 MHz, CDCl3): δ 165.9, 162.5, 149.9, 148.1, 132.9, 132.7, 122.7, 118.7, 118.1, 107.7, 60.08, 53.4, 52.7 ppm; MS: m/z = 370 (M+). Anal. Calcd for C13H11BrO6: C, 45.50; H, 3.32; O, 27.9. Found: C, 45.42; H, 3.30; O, 27.9.
4.4.6 Diethyl 4-hydroxy-8-methoxy-4H-chromene-2,3-dicarboxylate (3f, C16H18O7)
White solid; mp 100.3–101.9 °C; IR (KBr) (νmax/cm−1): 3418.3 (OH stretching), 3020.8 (aromatic CH stretching), 1745.1, 1713.1 (CO stretching), 1654.6 (CC stretching); 1H NMR (300 MHz, CDC13): δ 7.14 (m, 2H, ArH), 6.90 (dd, 1H, J = 6.0, 3.0 Hz, ArH), 5.73 (d, 1H, CHOH, J = 3.0 Hz), 4.39 (q, 2H, J = 6.0 Hz, CH2), 4.31 (q, 2H, J = 6.0 Hz, CH2), 3.88 (s, 3H, OCH3), 3.08 (d, 1H, J = 3.0 Hz, OH), 1.38 (t, 3H, J = 6.0 Hz, CH3), 1.33 (t, 3H, J = 6.0 Hz, CH3) ppm; 13C NMR (75.4 MHz, CDCl3): δ 165.7, 162.2, 150.1, 147.7, 139.1, 125.3, 121.8, 120.8, 111.6, 107.4, 62.5, 61.5, 60.4, 56.1, 14.0, 13.8 ppm; MS: m/z = 322 (M+). Anal. Calcd for C16H18O7: C, 59.68; H, 5.63; O, 34.75. Found: C, 59.65; H, 5.60; O, 34.73.
4.4.7 Diethyl 4-hydroxy-6-nitro-4H-chromene-2,3-dicarboxylate (3g, C15H15NO8)
White solid; mp 113.5–115.2 °C; IR (KBr) (νmax/cm−1): 3441.8 (OH stretching), 3050.0 (aromatic CH stretching), 1741.7, 1704.7 (CO stretching), 1662.8 (CC stretching); 1H NMR (300 MHz, CDC13): δ 8.49 (d, 1H, J = 3.0 Hz, ArH), 8.23 (dd, 1H, J = 9.0, 3.0 Hz, ArH), 7.28 (m, 1H, ArH), 5.79 (d, 1H, J = 3.0 Hz, CHOH), 4.37 (m, 2H, CH2), 3.52 (d, 1H, J = 3.0 Hz, OH), 1.377 (m, 3H, CH3) ppm; 13C NMR (75.4 MHz, CDCl3): δ 165.1, 161.5, 152.9, 149.16, 144.9, 126.5, 125.2, 121.9, 117.9, 108.2, 62.9, 62.0, 59.9, 14.0, 13.8 ppm; MS: m/z = 337 (M+). Anal. Calcd for C15H15NO8: C, 53.42; H, 4.48; O, 37.95. Found: C, 53.41; H, 4.46; O, 37.93.
4.4.8 Diethyl 6-bromo-4-hydroxy-4H-chromene-2,3-dicarboxylate (3i, C15H15BrO6)
White solid; mp 83.2–85 °C; IR (KBr) (νmax/cm−1): 3466.51(OH stretching), 3074.8 (aromatic CH stretching), 1718.1 (CO stretching), 1656.1 (CC stretching); 1H NMR (300 MHz, CDC13): δ 7.67 (d, 1H, J = 3.0 Hz, ArH), 7.44 (dd, 1H, J = 9.0, 3.0 Hz, ArH), 7.03 (d, 1H, J = 6.0 Hz, ArH), 5.69 (d, 1H, J = 3.0 Hz, CHOH), 4.38 (q, 2H, J = 6.0 Hz, CH2), 4.30 (q, 2H, J = 6.0 Hz, CH2), 3.27 (d, 1H, J = 3.0, OH), 1.38 (t, 3H, J = 6.0 Hz, CH3), 1.32 (t, 3H, J = 6.0 Hz, CH3) ppm; 13C NMR (75.4 MHz, CDCl3): δ 165.4, 162.0, 149.9, 148.0, 132.8, 132.6, 122.6, 118.5, 117.9, 107.3, 62.6, 61.7, 60.0, 14.0, 13.8 ppm; MS: m/z = 370 (M+). Anal. Calcd for C15H15BrO6: C, 48.54; H, 4.07; O, 25.86. Found: C, 48.53; H, 4.02; O, 25.80.
4.4.9 Dimethyl 2-hydroxy-2H-chromene-2,3-dicarboxylate (4a, C13H12O6)
White solid; mp 141.6–142.2 °C; IR (KBr) (νmax/cm−1): 3459.3 (OH stretching), CH 3018.7 (aromatic CH stretching), 1737.6, 1703.6 (CO stretching), 1637.5 (CC stretching); 1H NMR (300 MHz, CDC13): δ 7.83 (s, 1H, olefinic H), 7.37 (m, 2H, ArH), 7.05 (m, 2H, ArH), 5.12 (s, 1H, 1H, OH), 3.92 (3H, s, OCH3), 3.84 (3H, s, OCH3) ppm; MS: m/z = 264 (M+). Anal. Calcd for C13H12O6: C, 59.09; H, 4.58; O, 36.33. Found: C, 59.02; H, 4.50; O, 36.30.
4.4.10 Dimethyl 2-hydroxy-8-methoxy-2H-chromene-2,3-dicarboxylate (4b, C14H14O7)
White solid; mp 179–181 °C; IR (KBr) (νmax/cm−1): 3405.2 (OH stretching), 3007.7 (aromatic CH stretching), 1758.9, 1709.9 (CO stretching), 1645.2 (CC stretching); 1H NMR (300 MHz, CDC13): δ 7.82 (s, 1H, olefinic H), 6.99 (m, 3H, ArH), 5.18 (s, 1H, OH), 3.92 (s, 3H, OCH3), 3.90 (s, 3H, OCH3), 3.84 (s, 3H, OCH3) ppm; 13C NMR (100 MHz, CDCl3): δ 163.0, 161.3, 151.0, 148.5, 147.0, 123.4, 118.7, 117.4, 107.5, 97.9, 55.5, 52.2, 51.9, 50.7 ppm; ms: m/z = 294 (M+). Anal. Calcd for C14H14O7: C, 59.62; H, 5.63; O, 34.74. Found: C, 59.60; H, 5.61; O 34.72.
4.4.11 Dimethyl 6-bromo-2-hydroxy-2H-chromene-2,3-dicarboxylate (4e, C13H11BrO6)
White solid; mp 73.5–75 °C; IR (KBr) (νmax/cm−1): 3461.3 (OH stretching), 3010.9 (aromatic CH stretching), 1713.7 (CO stretching), 1664.8 (CC stretching); 1H NMR (250 MHz, CDCl3): δ 7.70 (s, olefinic H), 7.67 (d, 1H, J = 5.0 Hz, ArH), 7.40–7.45 (dd, 1H, J = 7.5, 5.0 Hz, ArH), 7.03–7.06 (d, 1H, J = 7.5 Hz, ArH), 5.75 (s, OH), 3.87 (s, 3H, OCH3), 3.79 (s, 3H, OCH3); 13C NMR (62.5 MHz, CDCl3): δ 166.5, 163.6, 148.7, 143.5, 132.1, 131.6, 121.3, 118.3, 117.1, 106.4, 61.8, 52.6, 51.3; MS: m/z = 342.1(M+). Anal. Calcd for C13H11BrO6: C, 45.50; H, 3.23; O, 27.98. Found: C, 45.54; H, 3.20; O, 27.97.
4.4.12 Diethyl 6-bromo-2-hydroxy-2H-chromene-2,3-dicarboxylate (4i, C15H15BrO6)
White solidified oil; IR (KBr) (νmax/cm−1): 3468.11 (OH stretching), 3069.1 (aromatic CH stretching), 1715.5 (CO stretching), 1658.2 (CC stretching); 1H NMR (250 MHz, CDCl3): δ 7.71 (s, 1H, olefinic H), 7.43 (m, 3H, ArH), 5.53 (s, OH), 4.45 (q, 2H, J = 6.01 Hz, CH2), 4.32 (q, 2H, J = 6.01 Hz, CH2), 1.37 (t, 3H, J = 6.01 Hz,CH3), 1.30 (t, 3H, J = 6.01 Hz, CH3); 13C NMR (62.5 MHz, CDCl3): δ 165.1, 1631.3, 149.7, 146.6, 133.9, 125.3, 119.2, 116.1, 107.2, 61.8, 61.0, 60.1, 57.5, 15.8, 14.1; MS: m/z = 370.7 (M+). Anal. Calcd for C15H15BrO6: C, 48.54; H, 4.07; O, 25.86. Found: C, 48.50; H, 4.12; O, 25.85.
Acknowledgment
The partial financial assistance from the Research Vice Chancellor of Azarbaijan Shahid Madani University is gratefully acknowledged.