1 Introduction
Oxidation reactions are among the most important transformations in synthetic chemistry and offer an important methodology for the introduction and modification of functional groups. Therefore, the oxidation of alcohols by metal complexes to the corresponding carbonyl compounds is a worthwhile goal [1,2]. Many different oxidants were used for the oxidation of alcohols such as pyridinium chlorochromate (PCC), pyridinium dichromate (PDC), KMnO4, MnO2, CrO3, H2O2 and so on. Most of these oxidizing reagents which can be used in a research laboratory in stoichiometric amounts are expensive or toxic [1,3]. Thus, offering innovative, “clean” methods which use environmentally friendly oxidants such as peroxides, molecular oxygen and tetra-n-butyl-ammonium-peroxy-monosulfate and non-toxic solvents in particular aqueous media could be a new challenge in this regard. Moreover, it has been found that reactions in water can facilitate access to different reactivity and selectivity patterns compared with those observed in common organic solvents due to its unique physical and chemical properties. However, the manifestation of a new catalytic oxidation system which is cost effective, non-toxic and environmentally friendly is receiving a good deal of attention.
Hydrogen peroxide oxidation is found to be less relatively economical because of its cost and poor efficiency [4,5]. Oxone (2KHSO5·KHSO4·K2SO4) has drawn a lot of attention as a mild and operative reagent for various organic transformations in recent years [6–12]. However, oxone is practically soluble in water. Then, perhaps the most significant drawback in the use of oxone is its application in aqueous or pH-controlled reactions [13]. Several salts of oxone have been reported, such as tetra-n-butyl-ammonium-peroxy-monosulfate (n-Bu4NHSO5, TBA-OX), tetra-n-pentyl-ammonium-peroxy-monosulfate, tetra-n-hexyl-ammonium-peroxy-monosulfate and benzyl-triphenyl-phosphonium-peroxy-monosulfate. Recently, Lie et al. [14] have demonstrated TBA-OX as an effective reagent for the oxidation of alcohols in CH2Cl2 or H2O.
These tremendous advantages of TBA-OX have encouraged us to choose it as an oxidant in our study. There are several reagents available for the oxidation of sulfides and alcohols, but most of them are not satisfactory for medium to large scale synthesis due to the low content of effective oxygen, formation of environmentally unfavorably co-products, and high cost [15]. An aqueous solution of TBA-OX is an excellent oxidant in terms of being environmentally friendly, safe in storage and operation [14,15].
In this work, copper (II) phthalocyanine nanoparticles were used as a catalytic system. Phthalocyanines (Pcs) constitute a remarkably versatile and robust class of compounds with diverse technological applications [16–18]. Phthalocyanine transition metal complexes are important industrial pigments which have been considered as potential oxidation catalysts due to their facile preparation on a large scale, chemical and thermal stabilities. Metallophthalocyanines have been used as efficient biomimetic catalysts for the oxidation, reduction, and other reactions of different organic compounds. However, the low solubility of metallophthalocyanine catalysts is the most important problem that is often encountered by the catalyst in different reactions [8].
The extensive use of toxic and volatile organic solvents in these oxidation systems has reduced considerably the practical importance of biologically inspired oxidation catalysis. The discarding of harmful organic solvents is a problem in chemical industries which accounts for about 80% of their wastes [13]. Therefore, using non-toxic solvents in aqueous media can play a significant role in these reactions and a new challenge could be done to make such a process in aqueous solutions or under solvent free conditions [19–23].
Nanoinorganic materials are an interesting subject of research because of their unique physical and chemical properties [24]. Over hundred papers describing inorganic nanoparticle reactions with different materials have appeared in chemical literature studies in recent years. Nanoparticles have recently emerged as efficient alternatives for the immobilization of homogeneous catalysts. Nevertheless, difficulties in recovering the nanoparticles from the reaction mixture result from effective dispersion in solution by forming stable suspensions, which limit their wide applications [25,26].
In continuation of our interest in exploring the oxidation of organic compounds [27–30], this study aims to report a mild and effective heterogeneous strategy for the aqueous oxidation of alcohols by n-Bu4NHSO5 and copper (II) phthalocyanine as catalysts (Scheme 1). However, to the best of our knowledge, the use of copper (II) phthalocyanine nanoparticle catalysts for the oxidation of alcohols has not been previously reported.

Oxidation of alcohols with n-Bu4NHSO5 catalyzed by copper (II) phthalocyanine nanoparticles.
2 Experimental
1H and 13C spectra were determined on a Varian Mercury 300 MHz or a Bruker AM-400. Gas chromatographic analyses were performed on a Hewlett Packard 6890 instrument equipped with a FID detector and an HP-1 (either a 10 m × 0.2 mm i.d. or a 25 m × 0.2 mm i.d.) capillary column.
2.1 Preparation of the nano-CuPc
Copper (II) phthalocyanine was synthesized according to literature procedures [27–30]. Then, copper phthalocyanine nanoparticles up to 42 nm were prepared pursuant to the following procedures. Briefly, 0.15 g CuPc was first dissolved in 5 ml 98% concentrated sulfuric acid, and then the solution was added drop-by-drop into a 300 ml aqueous solution containing 0.45 g hexadecyltrimethyl ammonium-bromide in an ultrasonic ice-water bath. Isolation of phthalocyanine nanoparticles from a solution phase was done by using a simple centrifuge.
The obtained nanoparticles were washed immediately with hydrochloric acid twice, to remove bromide and then washed with deionized water three times. The final product was dried at 40 °C under vacuum.
Characterization of copper (II) phthalocyanine nanoparticles was performed with a SEM as shown in Fig. 1[31,32]:

SEM images of copper (II) phthalocyanine nanoparticles with average diameters of 42 nm.
Confirmation of the catalyst structure was done by elucidating UV (Fig. 2). Fig. 2 shows the absorption spectrum of CuPc and its related nanoparticles. Other metal phthalocyanines have very similar absorption spectra in the visible spectral region; these bands were assigned to π→π transitions of the macrocyclic π-system [33].
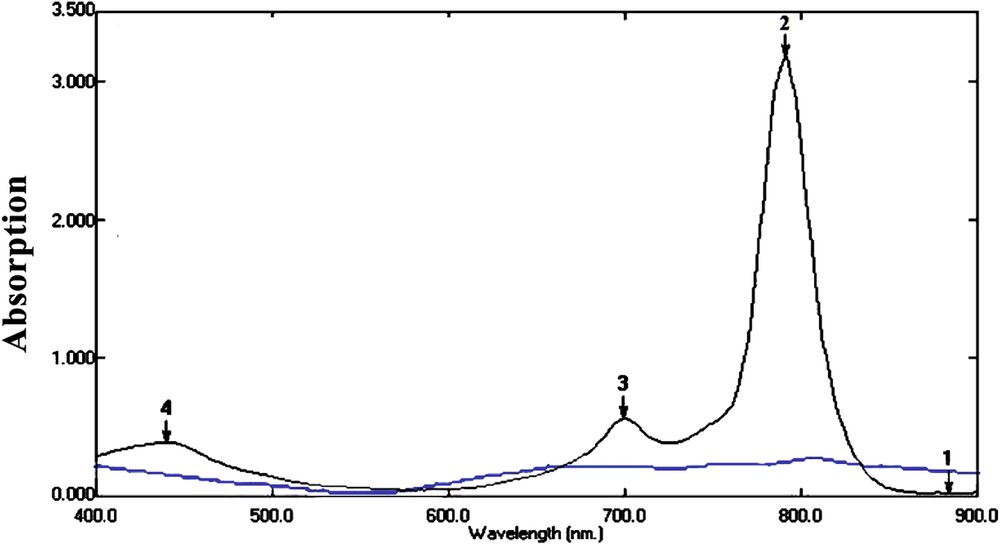
Absorption spectra of CuPc (black) and its related nanosized particles (red) in the ultra-violet and visible (UV–Vis) wavelength ranges.
The oxidant (TBA-OX) was prepared from commercially available oxone and n-Bu4NHSO4 by a procedure described in the literature [34].
3 Results and discussion
Copper (II) phthalocyanine nanoparticles have provided a valuable tool for the synthesis of both aliphatic and aromatic carbonyl compounds. In a typical reaction, n-Bu4NHSO5 as an oxidant (two equivalents) was added to the magnetically stirred aqueous solution of a mixture of alcohol (1 mmol) and copper (II) phthalocyanine. The oxidation of cyclohexanol with TBA-OX as an oxidant in aqueous media did not proceed in the absence of catalyst. Introductory catalytic experiments were addressed to the oxidation of cyclohexanol (1 mmol) with n-Bu4NHSO5 (0.7 g) in the presence of desired copper (II) phthalocyanine nanoparticles (0.5 mol%) in aqueous solution at 25 °C under air which led to the formation of cyclohexanone in 55% yield after 8 h according to GC analysis. Then, the temperature effect on the aqueous oxidation of cyclohexanol using n-Bu4NHSO5 catalyzed by nano-CuPc was examined. It can be concluded from Fig. 3 that an increase in the temperature remarkably increased the effectiveness of the catalytic system. The full conversion of cyclohexanol was achieved at 85 °C within 72 min (Fig. 3).

The effect of temperature on the aqueous oxidation of cyclohexanol using tetra-n-butyl-ammonium-peroxo-monosulfate catalyzed by nano-CuPc.
Under these conditions, the oxidation reaction proceeded smoothly and cyclohexanone was secured in 95% yield after a simple extraction from the aqueous phase. It is worth mentioning that blank experiments for the oxidation of cyclohexanol using TBA-OX as an oxidant at 70 °C gave just 10% of cyclohexanone after 1 h. The conversion of cyclohexanol as a function of temperature is shown in Fig. 3.
The yield of oxidation of cyclohexanol to the cyclohexanone as a function of time is indicated in Fig. 4.

The reaction profile for aqueous oxidation of cyclohexanol using n-Bu4NHSO5 catalyzed by nano-CuPc at 85 °C.
It is important to note that using organic co-solvents such as dichloromethane and n-hexane mitigated the reaction yields; 55, 47 and 6% yields have been obtained for water, dichloromethane/water and n-hexane/water, respectively.
The effect of increasing the amount of catalyst from 0.1 to 1 mol% on cyclohexanol oxidation and product selectivity was examined. When the catalyst amount was increased, the reaction yield also increased. For instance, 34, 71, 100 and 100 have been obtained for the 0.1, 0.2, 0.5 and 1 mol% of catalyst, respectively. It is worth mentioning that the increasing mol percent of catalyst up to 5 does not have any seminal effect on the reaction yields. However, the full conversion of cyclohexanol was achieved with a low catalyst loading of 0.5 mol %.
Recycling of the catalyst has also been studied. The catalyst was separated from the product by simple extraction with ether. n-Bu4NHSO4 can be recycled by removing the aqueous phase. Tetrabutylammonium hydrogen sulfate (TBAHS) is a phase transfer catalyst and can be reused in the preparation of TBA-OX. The procedure was repeated 4 times in the oxidation of cyclohexanol (Fig. 5) [35].

Recycling of the catalytic system for the oxidation of cyclohexanol.
In order to exploit the general applicability of the present catalytic system, several primary and secondary aliphatic and aromatic alcohols were selected for oxidation under optimized conditions in the aqueous solution. Secondary alcohols gave good yields and these are generally excellent substrates for this oxidation system (Table 1, entries1–8). Oxidation of primary alcohols such as benzylic and aliphatic occurred with a slow reaction rate and gave low to moderate yields of the related carbonyl compounds (entries 9–13). Total aromatic alcohols gave better yields than aliphatic alcohols in the oxidation reaction by this catalyst.
The oxidation of various alcohols.
Entry | Alcohol | Product | Yield% | M.P./B.P. of product (°C) |
1 | 64 | 56 | ||
2 | 95 | 153 | ||
3 | 89 | 161 | ||
4 | 87 | 113–115 | ||
5 | 86 | 205–208 | ||
6 | 90 | 49 | ||
7 | 94 | 115 | ||
8 | 92 | 202 | ||
9 | 79 | 179 | ||
10 | 85 | 249 | ||
11 | 83 | 204 | ||
12 | 88 | 48 | ||
13 | 92 | 105 |
Based on literature studies [36–38], the proposed mechanism for this catalytic oxidation reaction is depicted in Scheme 2. In the first step, copper phthalocyanine (CuPc) reversibly combines with TBA-OX. Apparently, due to the less steric hindrance around the terminal oxygen atom of the peroxo residue (HO–OSO3), the attachment of this species to the metal centre through the terminal peroxo oxygen atom is more facile relative to the other oxygen atoms of TBA-OX [39]. Cu(III)–OO species induces hydrogen abstraction from the studied alcohols resulting in the formation of an alcoholic radical. In a rebound process and subsequent cleavage, the corresponding carbonyl compound is produced [40,41].

Proposed mechanism for the oxidation reaction.
Table 2 shows a comparison between the catalytic efficiency of CuPc nanoparticles and other catalysts found in the literature. As seen in Table 2, the reaction did not proceed without any catalyst at room temperature. The use of 12-tungstocobaltate (II), NaCl, and TEMPO-IL/[bmim][PF6] as catalysts at room temperature gave 85, 65, and 90% yields at different times. Our catalyst was more effective in terms of yield and reaction time compared to the other reported catalysts.
A comparison between the catalytic efficiency of CuPc nanoparticles and other catalysts found in the literature for the oxidation of alcohol.
Entry | Oxidant | catalyst | Tempreture | Time/min | Yield/% [Ref] |
1 | TBOX | – | 60 | 180 | 10< |
2 | TBOX | 12-tungstocobaltate (II) | 50 | 180 | 85 [42] |
3 | TBOX | NaCl | 25 | 300 | 65 [43] |
4 | TBOX | TEMPO-IL/[bmim][PF6] | 60 | 30 | 90 [35] |
5 | TBOX | CuPC nanoparticles | 85 | 70 | 95 |
4 Conclusion
In conclusion, the catalytic performance of nanocopper (II) Phthalocyanine in the oxidation of alcohols using water as a solvent and n-Bu4NHSO5 as an oxidant was studied. The excellent yield was achieved for oxidation of alcohols at 85 °C with the absence of surfactants and nitrogenous bases. The employment of neat water as a ‘green’ solvent in this oxidation method gave high yield products as well as easy and safe work-up which are strong points of this novel methodology making it more attractive for practical goals. Organic co-solvents, surfactants, and co-catalysts were not used in this catalytic strategy. This system was green and time effective.
Acknowledgement
We gratefully acknowledge the financial support from the Research Council of Sharif University of Technology. We also thank Vivekanand V. Gobre at Fritz Haber institute der Max Planck for helpful discussions.