1 Introduction
Tetrazoles are an important class of nitrogen containing heterocycles with a wide range of medicinal and commercial applications [1,2]. The moiety has given a platform for the synthesis of many drugs such as sartan family drugs (Candesartan and Losartan) and antihistaminic drugs (Pemiroplast and Pranlukast) (Fig. 1) [3,4]. Tetrazoles are widely used in information recording systems [5], high-density energy materials [6], antifoggants in photography [7], propellants and explosives [8]. These are also studied as catalysts in asymmetric synthesis [9], ligands in coordination chemistry [10], cis-peptide bond mimics [11], lipophilic spacers [12], and ion and peptide chelating agents [13]. In addition, they have been used as resistants to metabolic degradation as well as chemical oxidants [14].
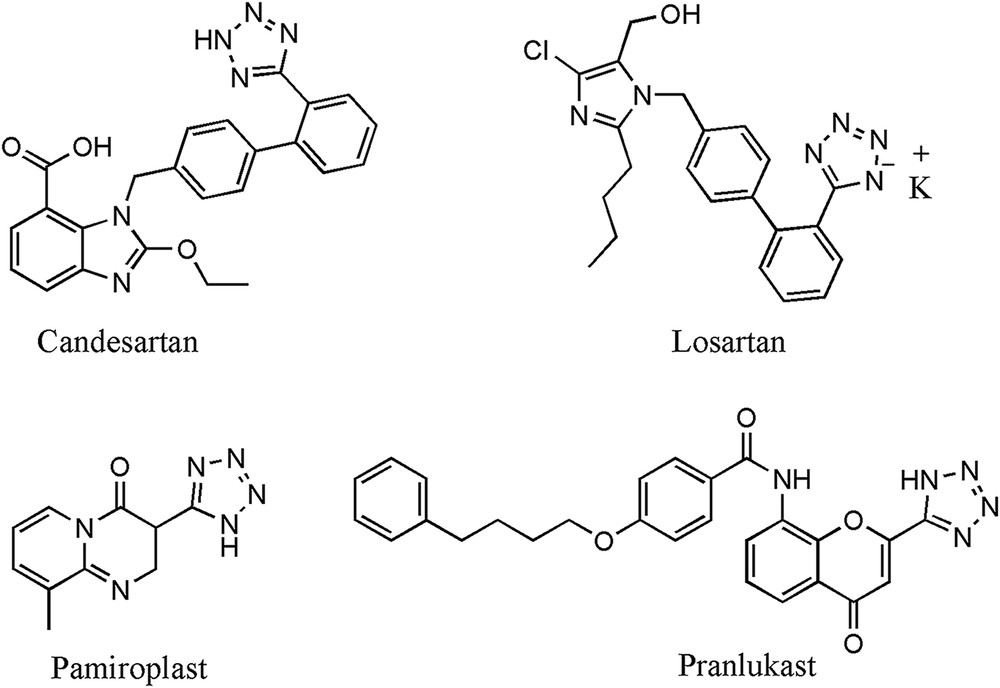
Chemical structure of pharmacologically important tetrazole drugs.
Such diversified applications of tetrazoles have prompted us to make a significant effort towards their synthesis. The synthesis of 5-substituted 1H-tetrazoles was first reported by Hantzsch and Vagt in 1901, which is based on [3+2] cycloaddition of azide with organic nitriles [15]. Since then, many reports have appeared in the literature for the synthesis of 5-substituted 1H-tetrazoles under the influence of several catalysts and different solvent conditions. The numerous catalysts and their methodology have also been reported till date such as copper triflates [16a], Fe(OAc)2 [16b], CdCl2 [16c], ZnBr2 [16d], triethyl amine hydrochloride [16e], (CH3)2SnO [16f], COY zeolites [17a], mesoporous ZnS nanospheres [17b], Zn hydroxyapatite [17c], Zn/Al hydrotalcite [17d], Cu2O [17e], Pd(PPh3)4 [17f], and nanocrystalline ZnO [17g] and CuFe2O4 nanoparticles [17h]. More recently, some newer catalysts such as Yb(OTf)3·×H2O [18a], B(C6F5)3 [18b], gold nanoparticles and auric chloride [18c] have also been reported for tetrazole synthesis. Although sodium azide has been used as the azide source in most of the above mentioned protocols, silicon [6], tin [19a] and organoaluminum azides [19b] have also been reported. Some of the above mentioned catalysts suffer from usual drawbacks such as long reaction time, expensive reagents, stoichiometric amounts of catalysts, strong Lewis acids, low yield and water sensitivity. Moreover, hydrazoic acid formed in situ is toxic, volatile and explosive [16f]. For example, Jamison et al. [20] and Kappe et al. [21] reported an innovative method for the synthesis of 5-substituted 1H-tetrazoles by using a continuous-flow microreactor, in this method, hydrazoic acid is used safely that formed in situ but it required higher temperature (190–260 °C) for synthesis. Moreover, Sharpless et al. [22] have also reported an efficient method for tetrazole synthesis with the use of a stoichiometric amount of Zn salt. However, this method also requires high temperature for sterically hindered aromatic and alkyl inactivated nitriles. Thus, there is still some scope to improve and develop new, simple and efficient synthetic methodologies to minimize these drawbacks.
Our research is mainly based on the design and synthesis of biologically active compounds [23]. To diversify our research, we focused our aim to find new catalysts for tetrazole synthesis. A literature survey reveals that lead is widely used in organic synthesis as organolead compounds. They are prepared by using lead(II) chloride with Grignard reagents in most of the cases [24]. Pb(II) reagents have been used as catalysts in one pot synthesis of quinoxalines [25a], substituted pyrroles [25b], aldol reactions [25c], oligo(C) formation from the 5-monophosphorimidazolide of cytidine [25d], oxidation of guanine [25e] and direct growth of single-walled carbon nanotubes [25f]. To the best of our knowledge, no report is available for the synthesis of tetrazoles catalyzed by Pb(II) salts. This led us to investigate whether Pb(II) salts could be useful for the synthesis of 5-substituted 1H-tetrazoles. In this study, we report an efficient method for the synthesis of 5-substituted 1H-tetrazoles from nitriles and sodium azide catalyzed by Pb(II) salts under mild conditions.
2 Experimental
2.1 Materials and methods
All reagents and solvents were obtained commercially and used without further purification. Nuclear magnetic resonance (1H and 13C) spectra were recorded on a JEOL GS-400 model FT-NMR (400 MHz for 1H and 100 MHz for 13C) spectrometer and processed with Delta software. The chemical shifts were measured in parts per million (ppm) on the delta (δ) scale relative to the resonance of tetramethylsilane (TMS) as the internal reference. FT-IR spectra were recorded in KBr pellets in the range of 4000–400 cm−1 at room temperature using a Perkin Elmer 400 FT-IR spectrometer. A single crystal of tetrazole (15b) for X-ray diffraction was mounted on glass fibers. The X-ray diffraction intensity data were measured at 293 K by using the X-ray scan technique on a Bruker AXS SMART APEX Single Crystal X-ray Diffractometer with a CCD-detector using graphite mono-chromatized Mo-Kα radiation (λ = 0.71073 Å). The data were corrected for Lorentz-polarization as well as for absorption effects. The structure of the compound was solved by the direct method using the program SHELXS-97, and was refined by using the full-matrix least-squares technique on F2 by SHELXL97. Scattering factors incorporated in SHELXL97 were used. All calculations were carried out using the WinGX package of the crystallographic program and PLATON. ORTEP-3 and Mercury were used to generate molecular graphics.
2.2 General procedure for the synthesis of tetrazoles
Benzonitrile (103 mg, 1 mmol) and sodium azide (97.5 mg, 1.5 mmol) were dissolved in 2 ml of dry DMF in a 25 ml round bottom flask. PbCl2 (27.8 mg, 0.1 mmol, 10 mol %) was added to the reaction mixture and stirred at 120 °C for 8 h under nitrogen. After completion of the reaction (as monitored by TLC), the reaction mixture was cooled to room temperature and 10 ml of ice water was added followed by addition of 3 N HCl until the reaction mixture became strongly acidic (pH 2–3). The reaction mixture was extracted three times with 20 ml ethyl acetate. The organic layer was washed with brine solution and dried over anhydrous sodium sulfate, and was evaporated under reduced pressure to give a white solid product of 5-phenyl 1H-tetrazole with 81% yield. The obtained products were sufficiently pure and characterized by IR, 1H NMR, and 13C NMR and compared with reported spectral data. The compound 15b was also characterized by X-ray crystallographic analysis.
3 Results and discussion
We aim to develop a better catalytic system by screening three Pb(II) salt catalysts [PbCl2, Pb(NO3)2, and Pb(CH3COO)2] for their ability to catalyze the [3+2] cycloaddition between benzonitrile and sodium azide in dimethylformamide (Table 1). Out of these, Pb(CH3COO)2 afforded 5-phenyl 1H-tetrazole in poor yield (55%) while Pb(NO3)2 afforded 5-phenyl 1H-tetrazole in moderate yield (69%). Moreover, these catalysts took a longer time (18h and 12h respectively) for the completion of the reaction. PbCl2 gave better yield (81%) in a shorter reaction time (8h). Therefore, Pb(NO3)2 and Pb(CH3COO)2 catalysts were eliminated from the scope of the present study. Further, PbCl2 is a stable salt, water tolerant and easy to handle. Overall, PbCl2 is an excellent option and was thus selected for further studies for the synthesis of tetrazoles (Scheme 1).
Optimization of the reaction conditions for the synthesis of tetrazole (1b)a.
Entry | Catalyst [mol %] | Solvent | Temp (°C) | Time (h) | Yieldb (%) |
1. | Nil | DMF | 120 | 12 | NRc |
2. | Pb(NO3)2 [10] | DMF | 120 | 12 | 69 |
3. | Pb(CH3COO)2 [10] | DMF | 120 | 18 | 55 |
4. | PbCl2 [10] | DMF | 120 | 8 | 81 |
5. | PbCl2 [5] | DMF | 120 | 14 | 40 |
6. | PbCl2 [15] | DMF | 120 | 8 | 83 |
7. | PbCl2 [20] | DMF | 120 | 8 | 82 |
8. | PbCl2 [10] | DMSO | 120 | 16 | 56 |
9. | PbCl2 [10] | EtOH | 78 | 24 | 15 |
10. | PbCl2 [10] | Toluene | 110 | 24 | 28 |
11. | PbCl2 [10] | THF | 65 | 24 | 11 |
12. | PbCl2 [10] | CH3CN | 80 | 24 | 0 |
13. | PbCl2 [10] | Acetone | 60 | 24 | 0 |
14. | PbCl2 [10] | 1,4-Dioxane | 100 | 24 | 0 |
15. | PbCl2 [10] | H2O | 100 | 24 | 0 |
a Reaction conditions: Benzonitrile (1 mmol), NaN3 (1.5 mmol) are used at given temp.
b Experimental yield.
c In the absence of catalysts, no reaction occurred after 12 h.
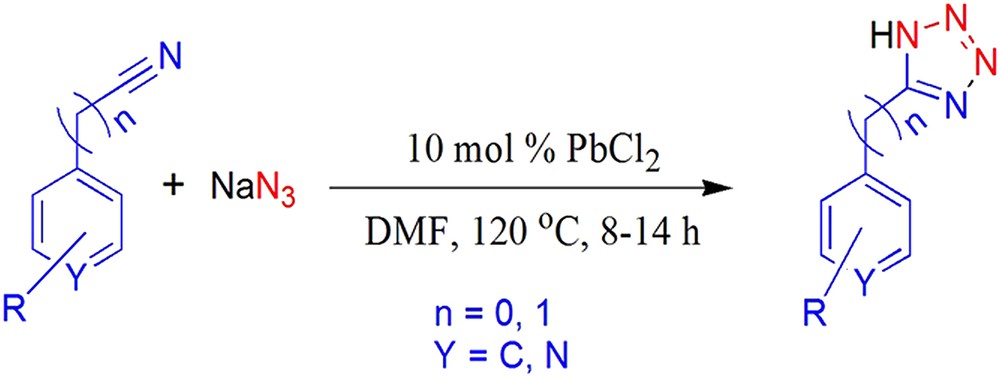
Reaction of nitriles with sodium azide catalyzed by PbCl2.
With the catalytic species chosen, studies were carried out to find the mildest reaction conditions for the synthesis of tetrazoles. Thus, the reaction conditions were standardized by monitoring the effect of catalyst loading, solvents (Table 1) and temperature (Table 2). The effect of catalyst loading was investigated in terms of product yield. In the absence of catalysts at 120 °C, no reaction occurred even after 12 h stirring (Table 1, entry 1). When the reaction was performed in the presence of 5 mol% PbCl2, the product was isolated in a lower yield (40%) in a longer reaction time (Table 1, entry 5). This yield was improved to 81% in a short reaction time when the reaction was carried out in the presence of 10 mol % of PbCl2 (Table 1, entry 4). Further, on increasing the concentration of the catalyst up to 15–20 mol%, there was no significant increase in yield (Table 1, entries 6 and 7). Hence 10 mol % of lead chloride was considered as an optimum catalyst concentration. The nature of solvents also played an essential role for the investigation of reaction time and product yield in experiments. It was observed that the reaction in polar protic solvents such as ethanol (Table 1, entry 9) resulted in only 15% product formation while in non-protic solvents such as toluene and THF (Table 1, entries 10 and 11) only 28% and 11% products were formed. Other solvents such as acetonitrile, acetone and 1,4-dioxane (Table 1, entries 12–14) also gave unsatisfactory results with no product formation even at elevated temperatures and 24 h stirring. We also tried a reaction in water towards the use of green solvents in reaction media but the desired product did not form (Table 1, entry 15). The best yields were obtained in DMF (Table 1, entries 4–7) while moderate yield was obtained in DMSO (Table 1, entry 8). On the basis of the above studies, DMF was found to be a better solvent for tetrazole synthesis than DMSO and other solvents.
Effect of temperature on the synthesis of 5-phenyl 1H-tetrazole(1b) in DMF.
Entry | Catalyst | Temp (°C) | Time (h) | Yield (%) |
1. | PbCl2(10 mol %) | 35(RT) | 17 | 0 |
2. | PbCl2(10 mol %) | 60 | 14 | 22 |
3. | PbCl2(10 mol %) | 80 | 14 | 45 |
4. | PbCl2(10 mol %) | 100 | 14 | 68 |
5. | PbCl2(10 mol %) | 120 | 8 | 81 |
6. | PbCl2(10 mol %) | 140 | 8 | 75 |
The reaction was also carried out using 10 mol % of PbCl2 at different temperatures (Table 2). Among the various temperature ranges tested, it was found that there was an increase in the yield with increase in temperature up to 120 °C (Table 2, entries 1–5). Further, there was no increase in the yield with increase in temperature (Table 2, entry 6). Hence, 10 mol % of catalyst at 120 °C in DMF was considered as an optimal condition for the synthesis of 5-substituted 1H-tetrazoles in the present study.
We next examined the scope of the PbCl2 promoted [3+2] cycloaddition of structurally diverse organic nitriles and sodium azide to form 5-substituted 1H-tetrazoles and the results are summarized in Table 3. In the case of aromatic nitriles, electron withdrawing and donating groups have a significant influence on the yield of products and reaction time. The aromatic nitriles containing an electron withdrawing group at the para-position gave better yields (Table 3, entries 2 and 3), while an electron donating group at the para-position gave lower yield comparatively in a longer reaction time (Table 3, entry 5). The nitriles containing both electron withdrawing as well as electron donating groups gave better yield (Table 3, entries 6–9), when compared to the nitrile containing an electron donating group (Table 3, entry 5). The nitriles containing protected amine, N-(4-cyanophenyl)benzamide, at the para-position gave the corresponding 1H-tetrazole in excellent yield (83%, Table 3, entry 12) while N-(4-cyanophenyl)acetamide gave lower yield (74%, Table 3, entry 10). Introduction of the nitro group at the meta position in N-(4-cyanophenyl)acetamide resulted in an improved yield (78%, Table 3, entry 11). When, the nitrile containing N-alkylated amine with nitrogen containing functionality, 4-({[1-(4-nitrophenyl)-1H-1,2,3-triazol-4-yl]methyl}amino)benzonitrile gave lower yield (73%, Table 3, entry 13) as compared to the protected amino nitriles. In addition to this, heterocyclic nitriles can also be used to prepare tetrazoles efficiently using PbCl2 as the catalyst (Table 3, entry 4). This methodology was also applied for the synthesis of benzyl tetrazoles in excellent yields, however, it took a longer time for the completion of reactions (Table 3, entries 14–17). From the above results, it is concluded that PbCl2 is an excellent catalyst for the synthesis of 5-substituted 1H-tetrzoles which tolerate a wide range of substituents irrespective of the electronic nature and position of the substituents on an aromatic ring involved in conversion. Moreover, the authenticity of this approach was also confirmed by X-ray crystallographic analysis of tetrazole compound 15b. For the X-ray study, good quality crystals of compound 15b were grown in a mixture of solvents (hexane:ethyl acetate, 2:8) by allowing slow evaporation at room temperature. The X-ray structure of the compound contains one molecule in the asymmetric unit (Fig. 2) and crystallized in the monoclinic crystal system with the P21/c space group, having the unit cell parameters: a = 7.8256(6) Å, b = 11.919(10) Å, c = 9.8796(8) Å, β = 96.769(4)°, V = 915.08(13) Å3, Z = 4 and Dx = 1.265 g cm−1. The crystal structure of tetrazole is stabilized by N–H⋯N (a) and C–H⋯N (b) intermolecular hydrogen bonding that exhibit a two-dimensional (2D) network. This network is decoded systematically and consistently into recognized terminological notations (Fig. 3).
PbCl2 catalyzed synthesis of 5-substituted 1H-tetrazolesa.
Entry | Substrate [a] | Product [b] | Time (h) | Yieldb (%) |
1 | 8 | 81, 74c | ||
2 | 8 | 79 | ||
3 | 8 | 77 | ||
4 | 8 | 80 | ||
5 | 10 | 75 | ||
6 | 10 | 78 | ||
7 | 10 | 80 | ||
8 | 10 | 80 | ||
9 | 12 | 78 | ||
10 | 10 | 74 | ||
11 | 10 | 78 | ||
12 | 10 | 83 | ||
13 | 10 | 73 | ||
14 | 12 | 80 | ||
15 | 14 | 74 | ||
16 | 14 | 76 | ||
17 | 12 | 81 |
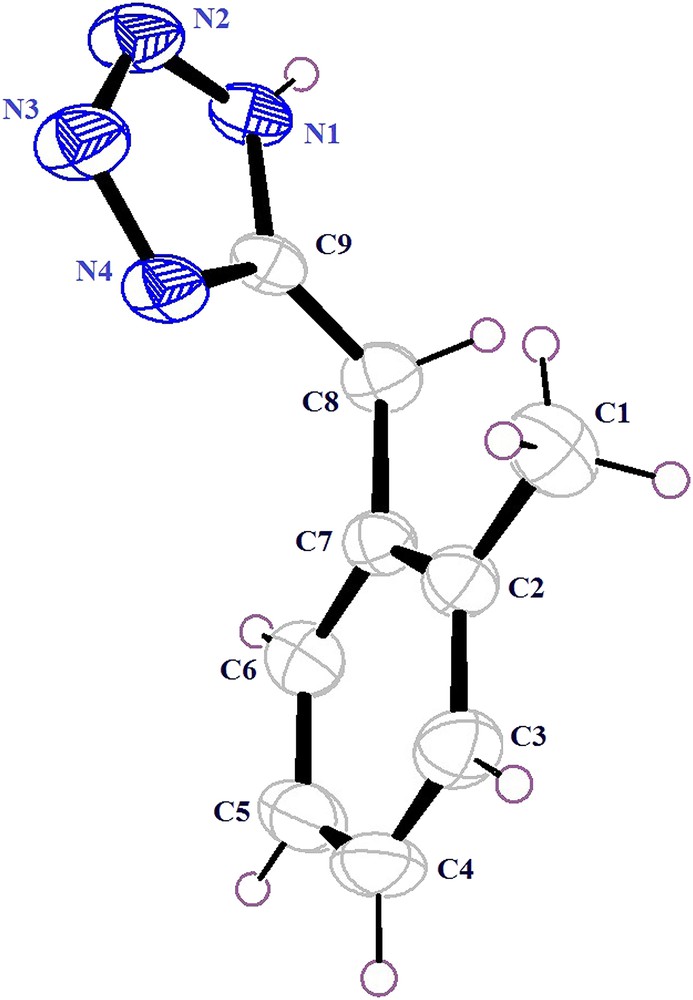
Ortep diagram of tetrazole 15b.

2D network of hydrogen bonding in tetrazole 15b.
The role of catalysts in the synthesis of tetrazoles is shown in Scheme 2. Presumably, the mechanism of the reaction proceeds through formation of Pb(N3)2 in situ by the action of NaN3 on Pb(II) salts [26], which facilitates the [3+2] cycloaddition reaction with nitriles to form 5-substituted 1H-tetrazole products.
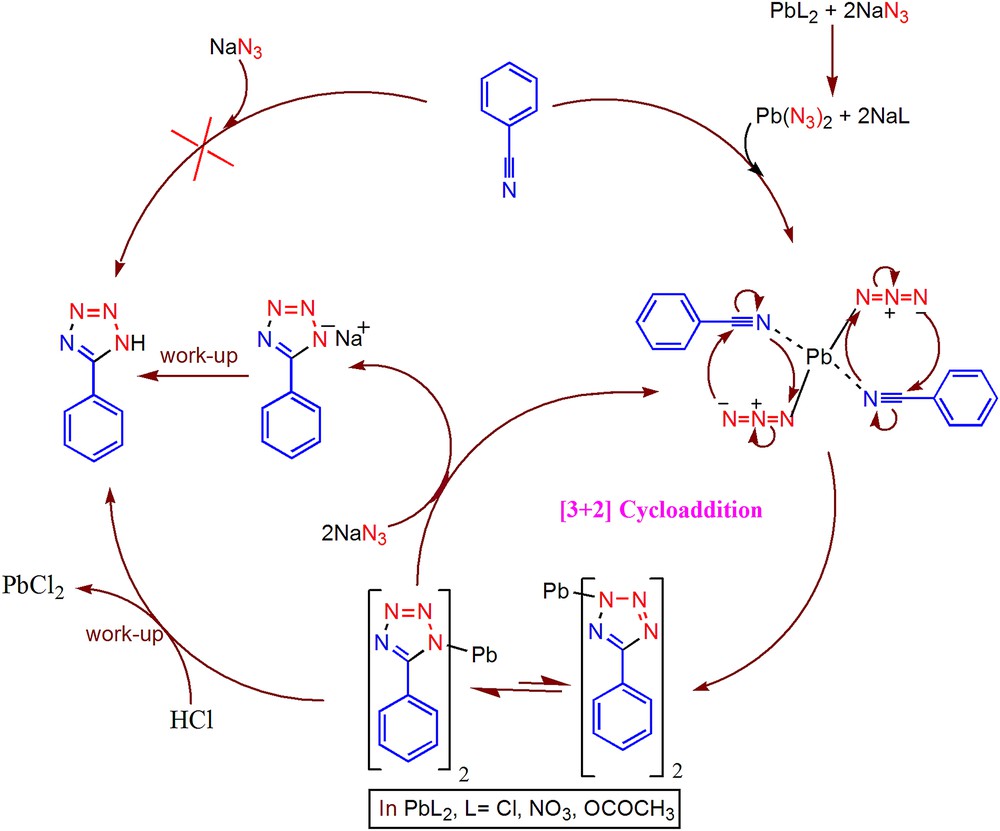
Plausible mechanism for the synthesis of tetrazoles catalyzed by Pb(II) salts.
The recovery or recyclability of catalysts is an important issue for chemical industries. Therefore, the recovery and reusability of lead chloride were investigated (Fig. 4). The catalyst was recovered easily at the end of the reaction by simple filtration due to its negligible solubility in water [27]. The recovered catalyst was washed with ethyl acetate & ethyl alcohol and dried under vacuum for further use. Our experiments showed that the recycled catalyst was reused over four catalytic cycles with only negligible loss of activity (Table 3, entry 1). The 1H NMR spectra of 5-phenyl 1H-tetrazole synthesized by using the recovered catalyst after the fourth cycle confirmed the purity of the products (Fig. S1).
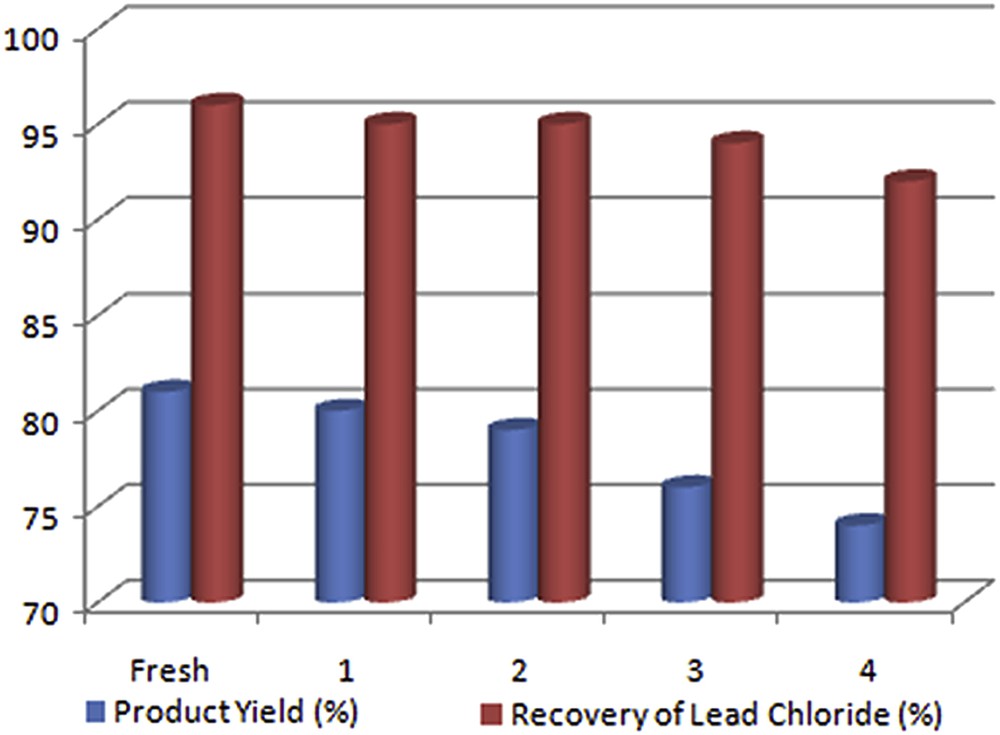
Recyclability of the PbCl2 catalyst.
4 Conclusions
In the present study, three Pb(II) salts have been used to catalyze the [3+2] cycloaddition between benzonitrile and sodium azide in dimethylformamide at 120 °C. PbCl2 was found to be the best catalyst with respect to yields and reaction times for the synthesis of tetrazoles. Substrates with electron withdrawing groups are found to be more reactive than those with electron donating groups whereas aromatic nitriles are more reactive than benzyl nitriles. Additionally, heterocyclic nitriles can also be used to prepare tetrazoles using this catalyst. Thus, this methodology should provide a facile route for the synthesis of other 5-substituted 1H-tetrazoles too.
Acknowledgments
RK is thankful to CSIR HRDG New Delhi, India (Grant no. 09/013(0541)/2014-EMR-I) for a Junior Research Fellowship and AA is thankful to Banaras Hindu University, Varanasi, India for financial assistance. We are also grateful to Dr. M. Nethaji, Department of Inorganic & Physical Chemistry, Indian Institute of Science, Bangalore for X-ray analysis.
Appendix A Supplementary data
The following is the supplementary data (The spectral data of the synthesized tetrazoles are given in supplementary information for this article. CCDC-1011441 for compound 15b contains the supplementary crystallographic data that can be obtained free of charge at http://www.ccdccam.ac.uk/const/retrieving.html or from the Cambridge Crystallographic Data Centre (CCDC), 12 Union Road, Cambridge CB2 1EZ, UK; fax: + 44(0)1223-336033 or email:deposit@ccdc.cam.ac.uk.) related to this article: