1 Introduction
Organisms produce material solutions using minerals in conjunction with organic polymers in order to provide not only protection but also for other purposes such as magnetic sensors, gravity sensing devices and iron storage and mobilization [1–4]. Among the biominerals identified (Fig. 1) let's quote silicates which are present in algae and diatoms [5], carbonates in in vertebrates [6], and calcium phosphates in vertebrates [7]. Regarding the organic part, collagen and chitin have been identified to give structural support to bones and shells [8,9].
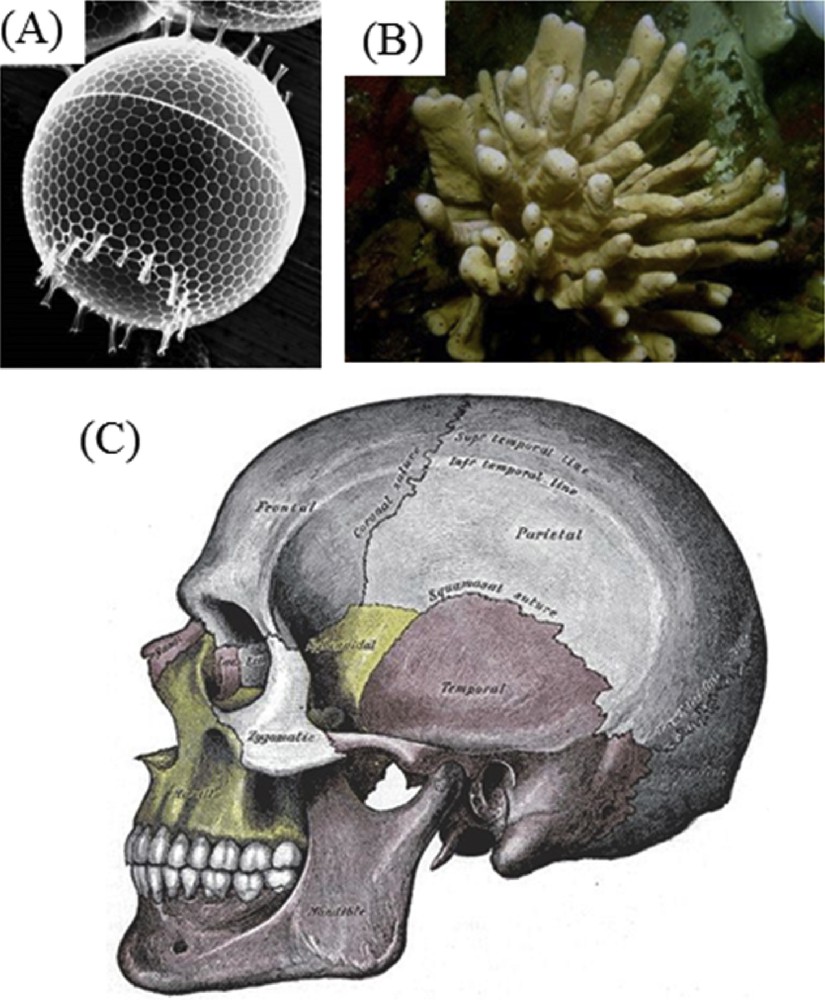
Biominerals present in different organisms: silicates (algae and diatoms), carbonates (invertebrates) and calcium phosphates and carbonates (vertebrates).
Organisms also produce materials when they dysfunction. This time, such a new family of biomaterials may contain information regarding the pathology which responsible of their pathogenesis. At this point it is worth to underline that pathological calcifications (PCs) [10–13] can be considered as a biomineralization depending on the definition. For some authors biomineralization constitutes a process by which organisms produce material solutions for their own functional requirements and thus PCs are excluded by such definition. For others, the definition is more general and in that case, biomineralization is the process by which living organisms produce minerals. We also have to underline that the number of chemical compounds related to synthesized biominerals is low compared to the one hundred chemical compounds identified in kidney stones.
From a chemical point of view, it is amazing to see that Mother Nature can elaborate a great structural complexity even with a limited number of chemical constituents through a precise control of the nucleation and the growth processes[14]. An illustration is given by calcium carbonate (Fig. 2).
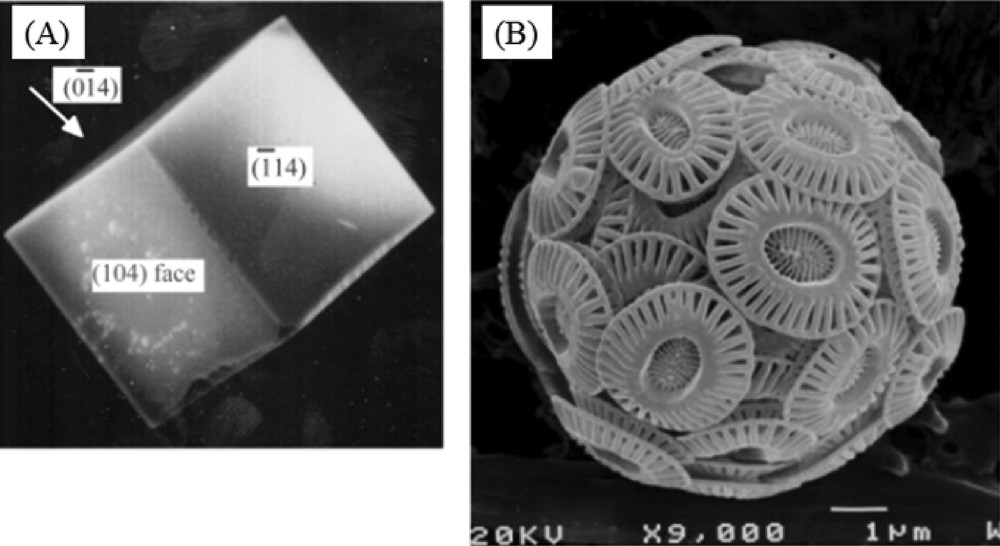
Scanning electron micrographs of (A) synthetic calcite, (B) Coccolithophorid, Emiliania huxleyi (from the website of the International Nannoplankton Association).
As we can see through scanning electron microscopy, the hierarchical structure present in Coccolithophorid cannot by explained by the simple morphology of the calcium carbonate crystal [15]. As underlined by J.J. De Yoreo and P.G. Vekilov [16], a complete physical picture of biomineral growth requires at least a description of the geometry and stereochemistry of the interaction between the crystal lattice and the organic modifiers.
Such complexity exists also for pathological calcifications. To exemplify such a parallel, we have to move from calcium carbonate to calcium oxalate crystallites present in kidney stones [17–19]. In Fig. 3A, we observe a radial and compact structure from the core of the stone as well as a more or less visible concentric organization. This structure present at the micrometer scale and the dark color of the stone observed through stereomicroscopy is related to a relatively slow growth, reflecting intermittent hyperoxaluria (excess of oxalate in urine) as a result of dietary conditions such as low beverage intake or high oxalate-rich vegetable consumption [20,21]. In Fig. 3B, SEM observations show a very peculiar aspect of crystallite agglomeration. This specific aspect has been consistently observed in all Ic kidney stones, which appear to be pathognomonic for primary hyperoxaluria [22]. This genetic disorder is associated with an overproduction of oxalate by the liver due to an enzymatic defect leading to a high risk of renal failure as a result of intratubular whewellite crystallization [23,24]. Thus, an early diagnosis is very important for initiating medical treatment as soon as possible. Our findings which are of clinical importance suggest that the SEM examination of the stone could be a valuable approach for detecting the stones related to this inherited disease instead of genetic investigations which are of high cost [25,26]. While the characterization of the mineral part of pathological calcification can be performed accurately at the subcellular scale through conventional techniques as well as through techniques specific to large scale instruments, little is known regarding the organic part. In fact, while the organic part of physiological calcifications is well defined by mother Nature, in the case of pathological calcification, a large number of organic molecules have been identified. Of note, in the case of kidney stones, many organic compounds present in trace amounts in urine, have been reported as potential inhibitors and thus may play a significant role in the crystal's morphology. Among them are chondroitin sulfate, phosphocitric acid, citric acid, ethylenediaminetetraacetic acid (EDTA), glycosaminoglycans, heparin homopolyribonucleotides, polyacrylate, polyaspartic acid, polyglutamic acid and RNA. Nevertheless, the fact that some specific morphology is related to a pathology (as it is the case for primary hyperoxaluria) seems to indicate that it is possible that an organic molecule may play a key role. Another possibility is related to peculiar sursaturation conditions related to the fact that the nucleation is done under “microfluidic” conditions.
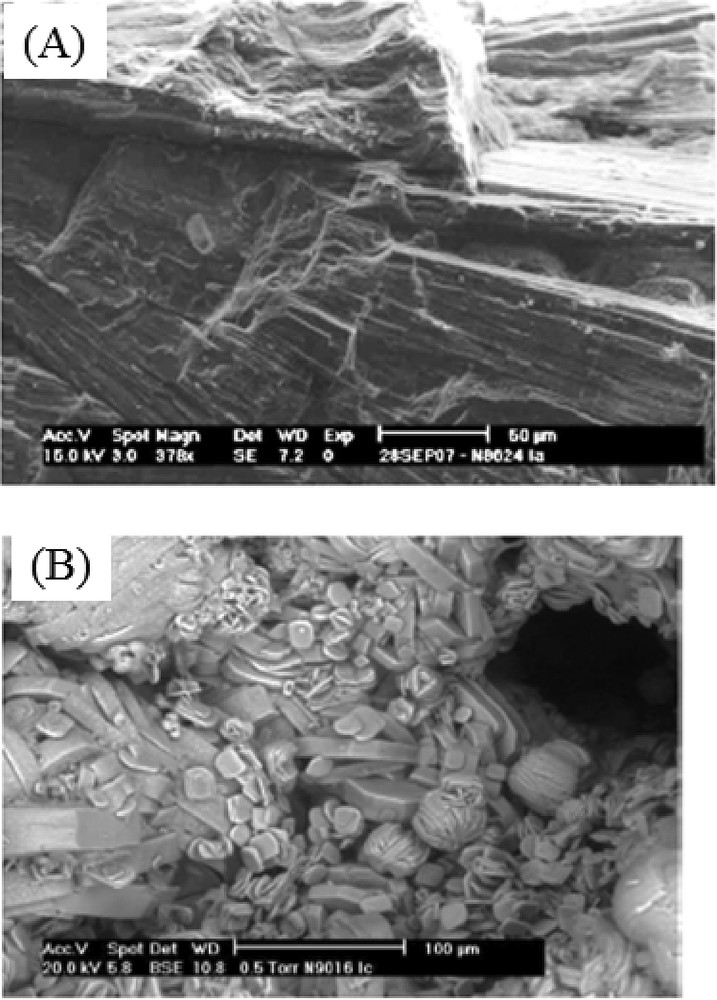
SEM images of (A) a Ia sample (N8624) and (B) a Ic sample (N9016).
We would like to present some recent results obtained thanks to a close collaboration with M. Daudon to whom this contribution is dedicated.
2 Some historic considerations
Clinical and experimental investigations within the sphere of pathological calcifications constitute an exciting research field at the interface between medicine, chemistry and physics. A necessary condition to perform such research is the existence of a model between structural characteristics of concretions and the pathology responsible for their occurrence. In the case of urolithiasis, a model developed 25 years ago by Dr. Michel Daudon was able to encompass different pathologies namely genetic, alimentation disorders, infection as well as drugs [27–30].
A parallel between the accomplishments of Michel Daudon regarding the study of biological crystals and the research performed by Louis Pasteur (1822–1895) on the role of the morphology of the tartaric acid crystal on polarized light crystal can be made [31]. In both cases, a careful observation of crystals led to a major breakthrough for the scientific community. Also, Louis Pasteur has already underlined through his investigation on crystals the usefulness of strong interactions between chemistry and crystallography [32].
Other outstanding scientists have performed a research at the interface between medicine, physics and chemistry. In a letter (Fig. 4) of Prof. J. Friedel (1921–2014) to whom we send different papers regarding pathological calcifications, the key role of major scientific people such as M. Curie (1867–1934) [33] or Prof. G. Friedel (1865–1933) [34] have been underlined. In the letter, Prof. J. Friedel wrote “On peut aussi penser à Marie Curie, partant vers le front en 14–18 avec sa fille, pilotant des camionnettes d'appareil de rayons X, puis développant dans l'institut Curie l'effet des radiations sur le cancer.”
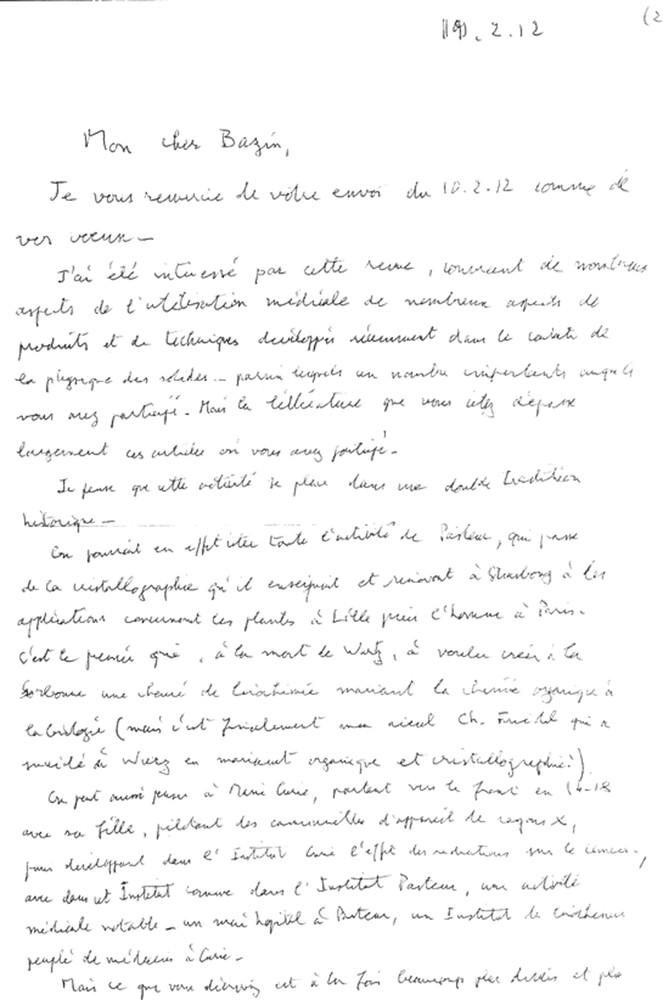
Part of the letter of J. Friedel regarding the interface medicine chemistry.
3 Bottom (molecules) – up (patients) versus top (patients) – down (molecules) approaches
Numerous papers have discussed the nucleation and the growing process of mineral composite [35–38] using two stratagems namely top-down rand bottom-up. Basically, the first one refers to the microfabrication method where tools are used to cut into the desired shape and order while the second one is related to methods where devices ‘create themselves’ by self-assembly. Regarding research on pathological calcifications, we may also consider bottom (molecules) – up (patients) and top (patients) – down (molecules) stratagems. For the first one, we may consider the research performed on the interaction of drug with cells in order to develop new therapeutic treatments or the interaction between the mineral and the organic part of the calcification. In our case, we are more close to a top (patients) – down (molecules) approach in which we consider directly biological samples such as kidney stones in order to develop original diagnostic tools or/and a precise description of the biochemical parameters related to the pathogenesis of these calcifications.
We would like now to present some selected results regarding these two stratagems. Regarding the bottom (molecules) – up (patients) approach, we would like to present a limited number of contributions regarding a new model on nucleation, as well as the role of the organic part and water. We will then present some of our results.
D. Gebauer et al. [39] show that dissolved calcium carbonate contains stable pre-nucleation ion clusters occurring even in an undersaturated solution. Such mechanisms are also important for the crystallization of other minerals. In Fig. 5, the conventional model and the new paradigm are compared. As shown, the very first steps of the genesis of the mineral are quite different.
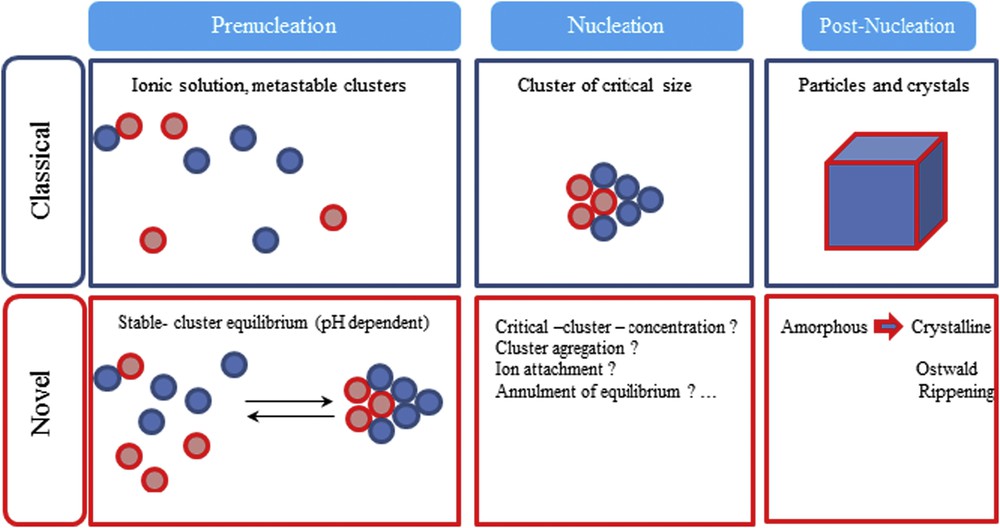
Schema of the classical and novel view on precipitation (not to scale). Prenucleation-stage calcium carbonate clusters provide an early precursor species of different amorphous calcium carbonate phases giving rise to an alternative crystallization-reaction channel (from Ref. 39).
Regarding the organic part, acidic extracellular matrix proteins play a pivotal role during biomineral formation. G. He et al. [40] report that dentin matrix protein 1 (DMP1), an acidic protein, can nucleate the formation of hydroxyapatite in vitro in a multistep process that begins by DMP1 binding calcium ions and initiating mineral deposition. Also, by combining high-resolution cryo-transmission electron microscopy and tomography with molecular modeling of the electrostatic potential energy distribution along a collagen chain in a fibril, Nudelman et al. [41] pointed out the presence of pre-nucleation clusters stabilized by polyaspartic acid. The following steps are related to the deposition of a dense network of pre-nucleation clusters, their subsequent transformation into amorphous calcium phosphate and finally into oriented crystalline hydroxyapatite inside the fibrils (Fig. 6).
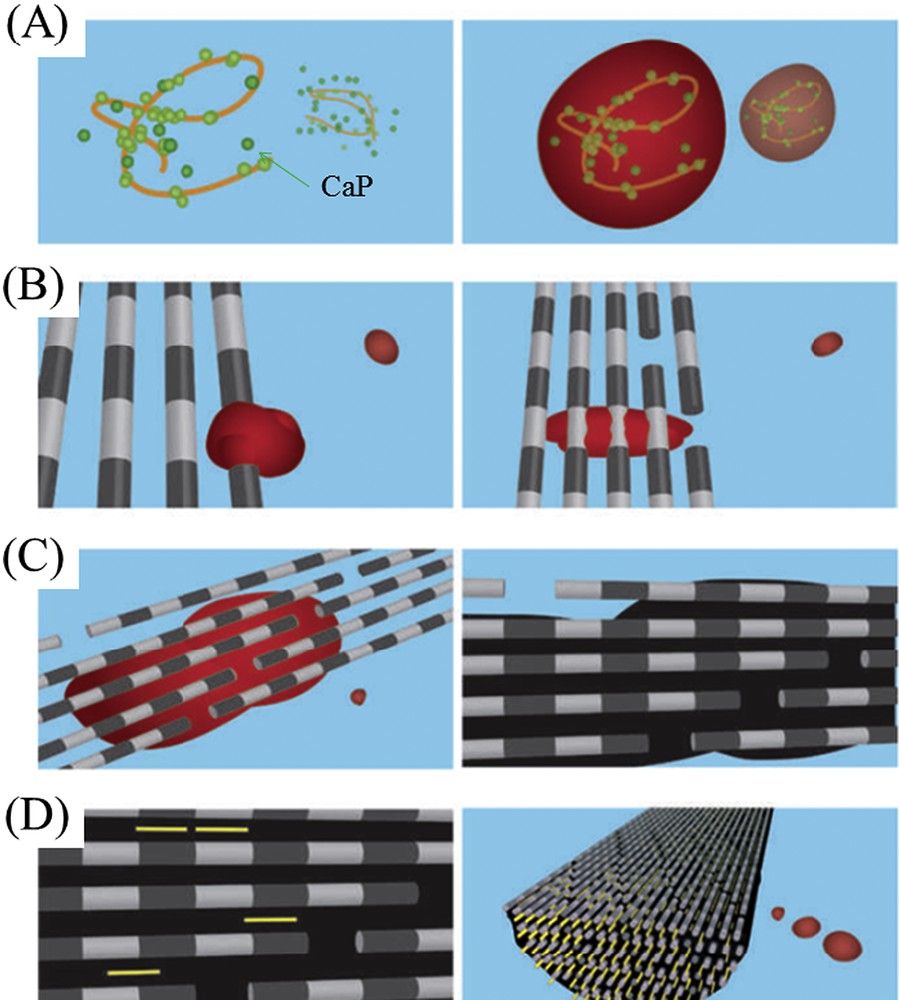
Mineralization of a collagen fibril (from Ref. 42). (A) Calcium phosphate (CaP) clusters (green) form complexes with the polymer (orange line), forming stable mineral droplets. (B), Mineral droplets bind to a distinct region on the collagen fibers and enter the fibril. (C) Once inside the collagen, the mineral in a liquid state diffuses through the interior of the fibril and solidifies into a disordered (amorphous) phase (black). (D) Finally, directed by the collagen, the amorphous mineral transforms into oriented apatite crystals (yellow).
Quite recently, J. Ihli et al. [43] underlined the role of water in the crystallization process (Fig. 7). These authors show that amorphous calcium carbonate can dehydrate before crystallizing. The high activation energy of this step suggests that it occurs by partial dissolution/recrystallization, mediated by surface water, and the majority of the particle then crystallizes by a solid-state transformation. Of note, water is also implicated in the ordering process [44].
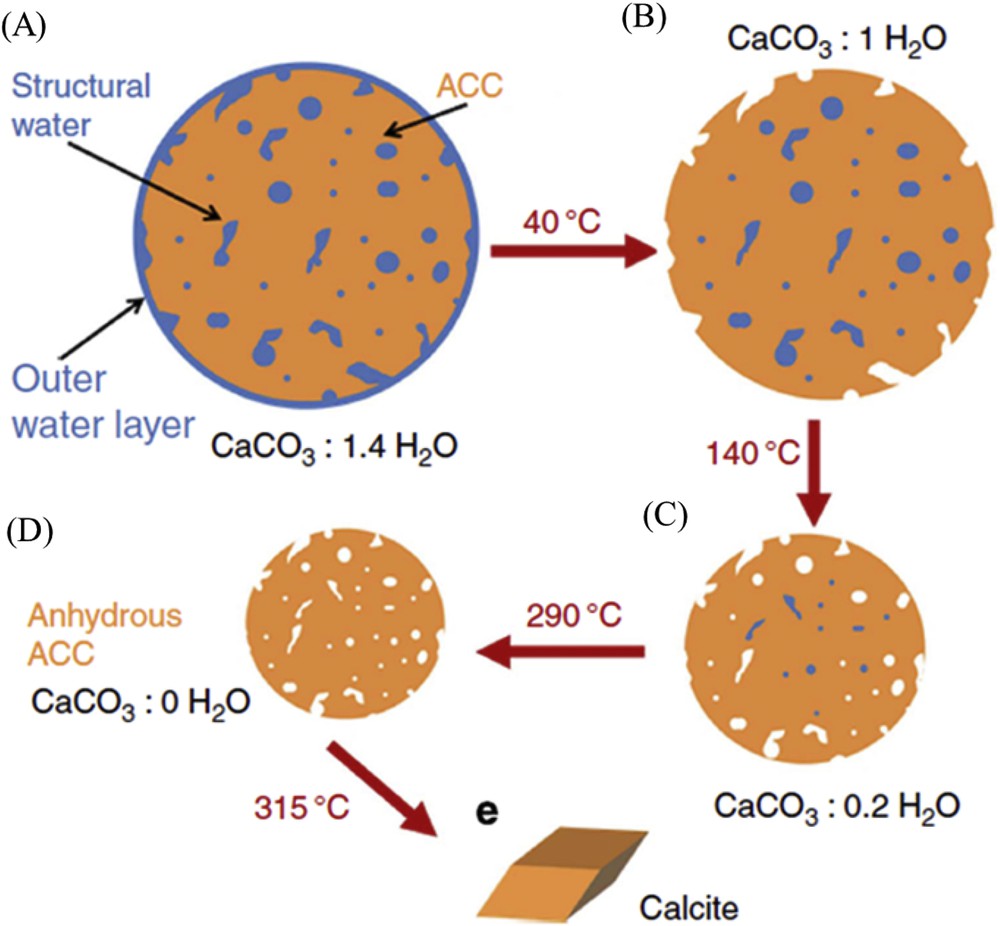
Schematic of stages of dehydration. On going from a to b, surface-bound water is lost, during b to c water is lost from the interior of the ACC and the ACC particle shrinks. On going from c to d, the most deeply located water is expelled and on going from d to e, crystallization to calcite occurs.
4 Some results regarding pathological microcalcifications
All the improvements listed above in the understanding of the mineralization process are of major importance. Nevertheless, in the case of tissue calcifications present in kidney papillae called Randall's plaque, their pathogenesis is intimately related to the tissue, a parameter which has not really been discussed in Bottom (molecules) – Up (patients) and which seems to play the role of a template.
Eight decades ago, Alexander Randall identified calcium phosphate deposits at the tip of renal papillae as the origin of renal calculi [45,46]. In France, stones harboring an umbilication (surface structure related to its interaction with the kidney tissue) corresponding to the attachment of stones to the papillae (Fig. 8) were found to be three times more frequent in the recent years than at the beginning of the 1980s and especially in younger patients population were younger and younger [47]. Such an increase observed in all industrialized countries has motivated numerous structural investigations [48–55].
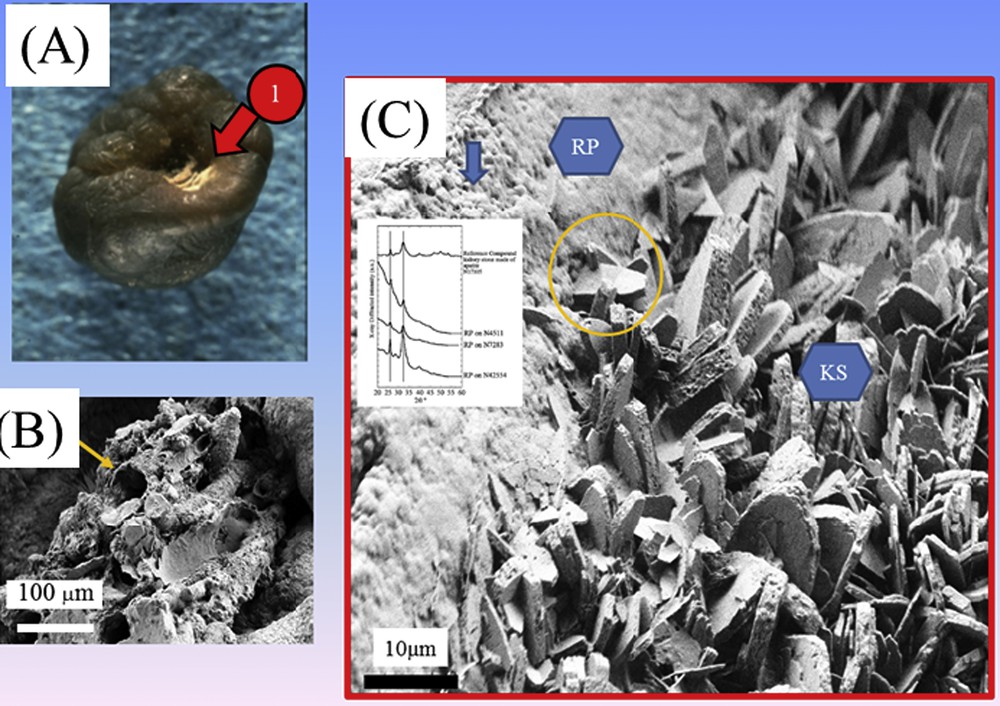
(A) Randall's plaque at the surface of a kidney stone made of calcium oxalate. (B) Randall's plaque at the mesoscopic scale showing an agglomeration of calcified tubes. (C) Interaction between the Randall's plaque and the kidney stones.
We have also made several publications on this subject [56–61]. In our case, we have assessed the structure of Randall's plaque at the mesoscopic scale (Fig. 8), the nature of the interaction between the Randall's plaque made of calcium phosphate apatite and the crystallites of calcium oxalate which constitute the kidney stones [61] as well as its relationship with inflammation through the Zn content [59]. The role of the tissue is visualized in Fig. 8 which shows that the plaque is made of a mixing of tubules with calcified walls and of tubules obstructed by calcium phosphate plugs. At this point, it is worth to underline that 3.5% of RP are made of sodium urate. If this number is small, it represents however a high number of patients, about 70,000 subjects in France.
5 Physico-chemical characterization techniques as diagnostic techniques
Regarding the mineral part of pathological calcifications, we used a set of characterization techniques which allowed us to assess their chemical composition as well as their topology and the presence of trace elements, the measurements being performed at the micrometer scale (Fig. 9).
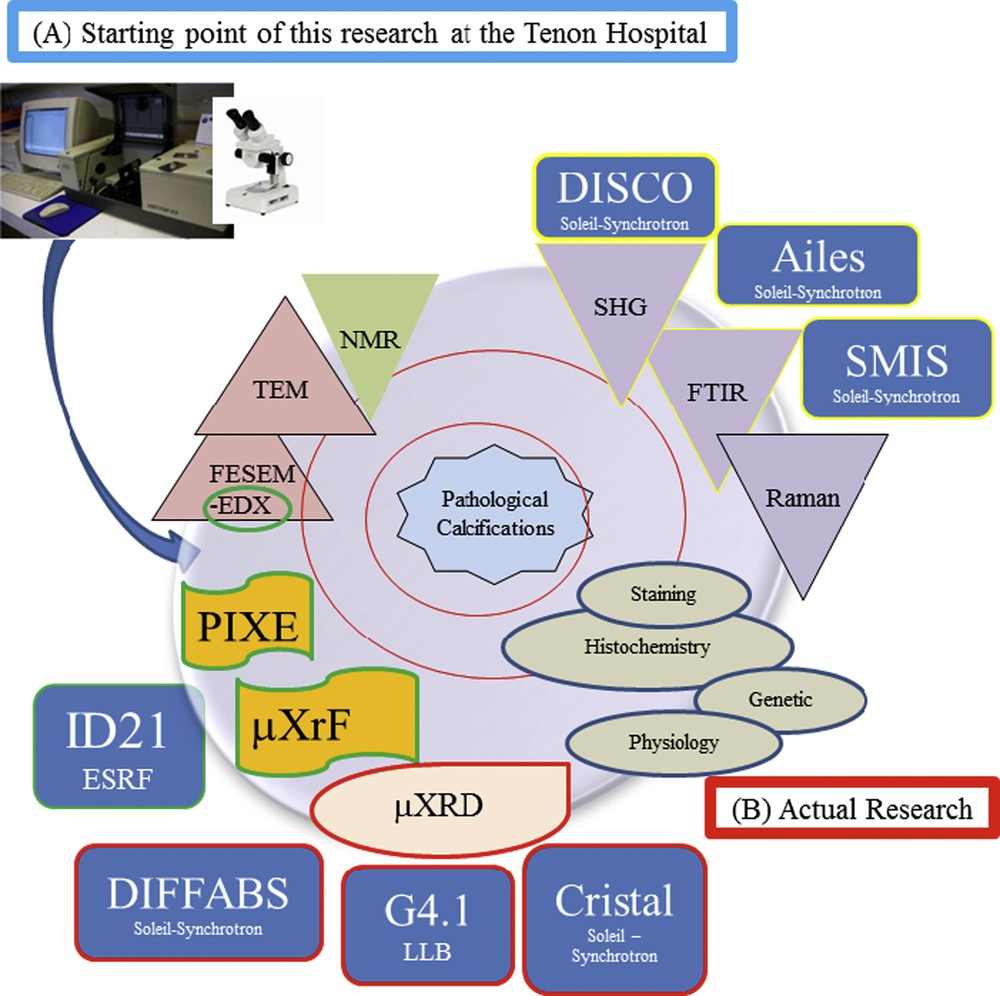
(A) Starting point of this research performed by M. Daudon at the Tenon Hospital combining FTIR spectroscopy and optical binocular. (B) Different characterization techniques used in this research.
We have already presented the benefits of Field Emission – Scanning Electron Microscopy in the case of hyperoxaluria [26]. During these last ten years, we have published other clinical cases where physicochemical techniques bring valuable information to the clinicians. I would now like to present these different improvements.
5.1 Synchrotron radiation – FTIR & a genetic pathology inducing ectopic calcifications
Adenine phosphoribosyltransferase deficiency is an inherited disease that is able to induce recurrent kidney stones and/or kidney failure [62–64]. This disease for which recent data suggest that it is not probably as rare as initially thought is responsible of the formation of dihydroxyadenine crystals [65]. For this genetic disorder, we use SR μFTIR spectroscopy for indirect diagnosis through the chemical identification of abnormal deposits [66]. Now, thanks to a new generation of μFTIR experimental devices, such measurements can be performed at the Tenon hospital. In our first series, two patients were diagnosed after renal impairment of the grafted kidney. They were treated with allopurinol and they recovered a large part of their kidney function. Such observations underline the clinical interest of early identification of crystals in the tissue. Finally, this investigation has motivated a characterization through infrared microspectroscopy (IR–MS) of kidney transplant crystal deposits [67] that are underdiagnosed [68].
5.2 SEM and asymptomatic infection
From an epidemiologic point of view, struvite stones are strongly associated with urinary tract infection (UTI) [69–71]. These stones represent 10–15% of all kidney stones in patients in industrialized countries [72] and are common in children. In a recent investigation [73], statistical analysis of the chemical composition of urolithiasis suggests that a family of chemical compounds namely struvite, amorphous carbonated calcium phosphate, whitlockite, proteins, triglycerides and mucopolysaccharides (MPS) share the particularity of being present mainly or exclusively in calculi related to urinary tract infection. These data pinpoint the importance of identify the different compounds present in stones in order to drive significantly the diagnosis [73].
At the hospital, physical methods such as FTIR or XRD are used to determine the chemical composition urolithiasis. Moreover, it was well known that in the case of infection the presence of bacterial imprints at the surface of kidney stones can be detected through SEM [74,75]. Based on a set of 39 urinary calculi mainly composed of CA without struvite, we were able to establish a relationship between the presence of bacterial imprints, indicative of past or current urinary tract infection, and both the presence of amorphous carbonated calcium phosphate (or whitlockite) and a high CR of CA [76]. A difficulty comes from asymptomatic patients with recurrent kidney stones for whom the chemical analysis cannot be related to infection process (i.e. without struvite, a low carbonatation level of the apatite…). For these patients, if we want to propose SEM investigations to underline the presence of bacterial imprints, it was necessary to understand why bacterial imprints cannot be found at the surface of struvite.
The answer of this apparent contradiction was given by neutron powder diffraction which points out the high crystal size for struvite [77]. Powder neutron diffraction allows probing the average size of the nanocrystals in struvite kidney stones. The high quality of the signal-to-noise ratio allowed a complete Rietveld-type refinement. The complete set of data shows that struvite and calcium carbonated apatite have very different sizes i.e. 25 nm for struvite crystals versus 5 nm for carbonated calcium apatite. To explain the absence of bacterial imprints on struvite stones, an analogy can be given by a man walking on a beach. If the beach is made of sand, footprints may be observed, but if the beach is made of stone, no footprints appear. Therefore, a relationship between the size of bacteria and the size of nanocrystals when looking for bacterial imprints is possible. This explains why bacterial imprints may appear with small calcium carbonated apatite nanocrystals rather than large struvite nanocrystals.
6 Conclusion
Research on pathological calcifications using last generation physicochemical techniques constitute an exciting research field. Basically, this research tries to
- - determine if a relationship between their physicochemical characteristics and the pathology responsible of their pathogenesis exists,
- - describe the biochemical parameters related to the pathogenesis in order to elaborate some drugs that are able to inhibit their formation
- - develop new diagnostic tools. Such characterization can be performed on microcalcifications and thus early diagnosis can be proposed
Now, thanks to the model proposed by M. Daudon, interesting results have been gathered also on pathological calcifications present in the breast, pancreas and thyroid. Studies are in progress.
Acknowledgments
We thank Dr. I. Brocheriou (Necker Hospital), Dr. X. Carpentier (Nice Hospital), Dr. Ch. Chappard (Lariboisiè̀re Hospital), Prof. P. Conort (La Pitié-Salpétrière Hospital), Dr. P. Dorfmüller (La Pitié-Salpétrière Hospital), Dr. E. Esteve (Tenon Hospital), Prof. D. Hannouche (Lariboisiè̀re Hospital), Dr. J.P. Haymann (Tenon Hospital), Prof. P. Jungers (Necker Hospital), Prof. B. Knebelman (Necker Hospital), Dr. E.A. Korng (Lariboisiè̀re Hospital), Dr. E. Letavernier (Tenon Hospital), Prof. F. Lioté (Lariboisiè̀re Hospital), Prof. M. Mathonnet (Limoges Hospital), Prof. P. Meria (Stain-Louis Hospital), Dr. C. Nguyen (Lariboisiè̀re Hospital), Dr. J. Rodes (Tenon Hospital), Pr. P. Ronco (Tenon Hospital), Dr. I. Tostivint (La Pitié-Salpétrière Hospital), Prof. O. Traxer (Tenon Hospital), and Prof. J.C. Williams (Department of Anatomy and Cell Biology, Indiana University School of Medicine, Indianapolis, Indiana, USA) for providing samples and useful discussions.
Also, regarding the physicochemistry, this research could not have been performed without the scientific advice of Dr. P.-A. Albouy (LPS), Dr. G. André́ (LLB), Dr. A. Bianchi (INSERM-U7561), Dr. P. Chevallier (LURE), Dr. A. Cousson (LLB), Dr. P. Dumas (Soleil Synchrotron), Dr. B. Fayard (LPS-ESRF), Dr. E. Foy (Laboratoire Pierre-Süe), Dr. J. Guicheux (Laboratoire d’Ingé́nierie Osté́o-Articulaire et Dentaire), Dr. J.-L. Hazemann (ESRF), Dr. A. Lebail (Laboratoire des Fluorures), Dr. F. Lenaour (Hôpital Paul-Brousse), Dr. O. Mathon (ESRF), Dr. G. Matzen (CEHMTI), Dr. C. Mocutta (Soleil Synchrotron), Dr. P. Reboul, (UMR 7561), Dr. M. Refringiers (Soleil Synchrotron), Dr. S. Reguer (Soleil Synchrotron), Dr. S. Rouziè̀re (LPS), Dr. J.-P. Samama (Soleil Synchrotron), Dr. C. Sandt (Soleil Synchrotron), Dr. D. Thiaudiè̀re (Soleil Synchrotron), Dr. E. Vé́ron (CEHMTI) and Dr. R. Weil (LPS).
This work was supported by the Physics and Chemistry Institutes of CNRS and by contracts ANR-09-BLAN-0120-02, ANR-12-BS08-0022, ANR13JSV10010-01, convergence UPMC CVG1205 and CORDDIM-2013-COD130042. The authors are grateful to the Soleil SR Facility and the Leon-Brillouin laboratory for beam time allocation.