1 Introduction
Learning from Mother Nature is not a specificity of materials science [1–3]. In the music of Olivier Messiaen (1908–1992), one of the most important composers of the twentieth century, birdsongs are considered as a section of music. A birdsong can be short with repetitive features but, more commonly, it is melodic and more elaborate than a simple call [4]. Another example can be related to the work of an architect, Otto Frei (1925–2015) who passed away recently. He wanted his buildings to contribute to improve everyone's living conditions. He tried to make as little impact as possible on nature and to learn from natural design, i.e. from the structures of crab shells, birds’ skulls or spider webs [5,6].
Biomineralisation processes constitute an exciting research field in which we have already learned a lot from Mother Nature [7–9]. In this contribution, we review a range of research outputs we have obtained regarding pathological calcifications [10–14], in order to show that it is possible to propose at least one example of a bioinspired approach to the field based on kidney physiology. Because the chemistry of the kidney is quite a wide subject, and one hundred chemical phases have been identified in kidney stones (KSs), here we decided to focus on biological entities, which can be considered as Janus particles. The concept of Janus particles was first proposed by De Gennes [15] 20 years ago in his Nobel Prize lecture, following the results obtained by C. Casagrande and M. Veyssié [16,17]. As defined by M.D. McConnell et al. [18], Janus particles are traditionally composed of two chemically distinct regions, making them suitable for applications as biological sensors, nanomotors, antireflection coatings, optical sensing devices, and two-phase stabilizers, as well as for fundamental studies in asymmetric particle assembly. As an illustration of Janus particles, a schematic representation of such entities has been provided in Fig. 1. For the sake of clarity, we only consider here metallic Janus particles [18–20]. As can be seen in the following figures (Fig. 1A–C), Janus particles may display distinct morphologies. In the case of nanotubes (Fig. 1D1 and D2), peculiar distributions of the second metal (in black) can lead to the construction of two enantiomers, leading to helimagnetism (in the case where the first metal is Pt and the second Co, for example) or enantiocatalysis [21].
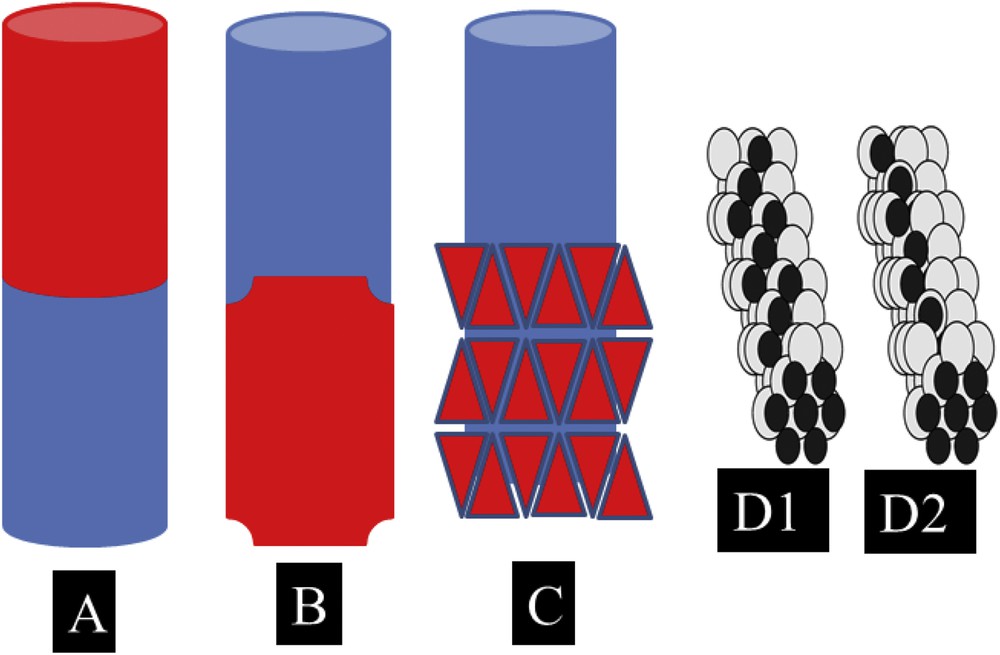
Schematic representation of metallic Janus particles. The lack of centrosymmetry may come from the chemistry (A) but also from both the chemistry and the structure (B and C). In some cases, peculiar Janus particles may be related to enantiomers (D1 and D2).
Following the definition provided by M.D. McConnell et al. [18], we start this contribution with a description of KSs at the macroscopic scale, in which at least two chemical phases have been identified. Details at the mesoscopic scale are then provided through observations performed using a latest generation scanning electron microscope (Field Emission Scanning Electron Microscope, or F.E.-S.E.M.). We will see that pathological calcifications exhibit peculiar mineral/organic ratios as well as crystal/amorphous interfaces. To the best of our knowledge, such a kind of interface is not used to generate Janus particles. Finally, we would like to propose that a chemical process based on the pathogenesis of Randall's plaque (RP) generates a deposit made of calcium phosphate apatite (CA) at the origin of a calcium oxalate KS.
2 Observations at the macroscopic scale
Regarding most of the KS samples, several chemical phases are identified. In the case of the KS made of calcium oxalate monohydrate (COM), (Fig. 2A), the presence of a whitish deposit termed RP [22–27], generally made of CA in a local hollow point on the surface of COM KS induces the dissymmetry. Actually, RP serves as a nucleus of the kidney stone and constitutes today a major public health problem in western countries. More complex structures exist, however, and the presence of more than one hundred chemical compounds has been identified in KSs. Fig. 2B shows a typical KS made of COM and calcium oxalate dihydrate (COD) on which a RP made of CA is present.
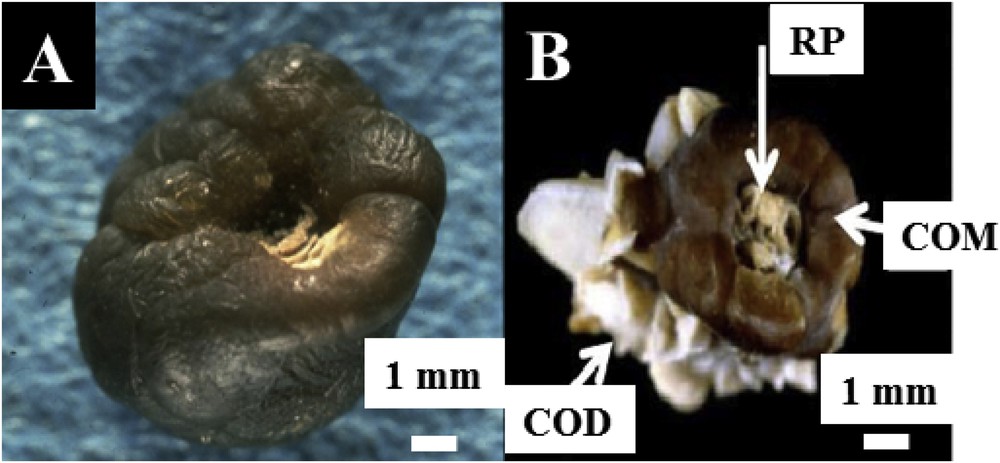
Different KSs observed at the macroscopic scale. (A) Classic KS made of COM. The white deposit on the surface of this COM is made of CA and is named RP. (B) Two calcium oxalate, COM and COD are clearly visible for this kidney stone. Again, a RP is present on the surface of COM.
3 Dissymmetry at the mesoscopic scale
Observations through FE-SEM underline that the complexity of pathological calcifications (Fig. 3A) exists also at the mesoscopic scales (Fig. 3B). For example, in the case of RP, observations (Fig. 3B) show that these calcifications are composed of a mixture of tubules with calcified walls and tubules obstructed by calcium phosphate plugs. Thus, the lack of centrosymmetry can be induced by the chemistry and the structure at the macroscopic and mesoscopic scales. In some cases, RP is made of sodium hydrogen urate monohydrate (UrNa) mixed with plates of COM (Fig. 2C).
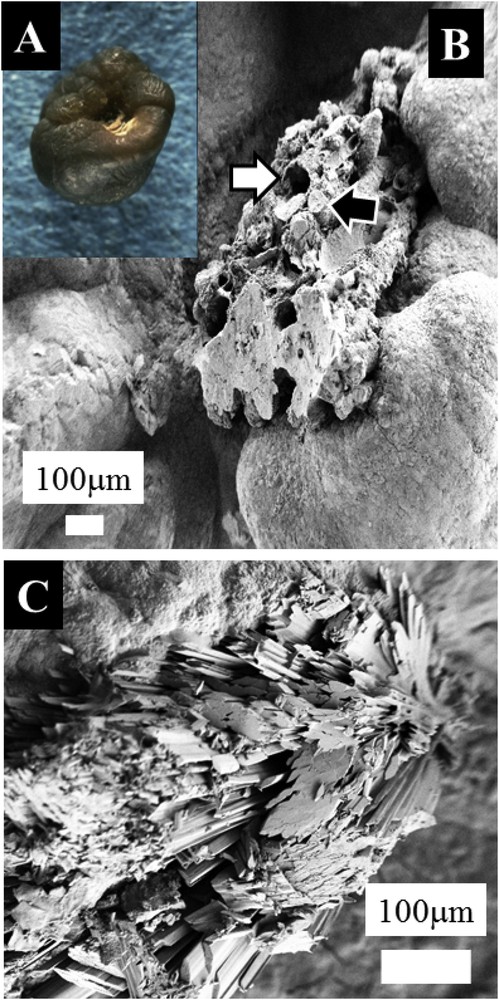
Optical (A) and scanning electron microscopy photographs (B) of calcium oxalate calculi initiated from a RP. The plaque is made of tubules with calcified walls and tubules obstructed by calcium phosphate plugs. (C) RP is made of NaUr and plates of COM.
The final illustration is crystals present in urine [28,29]. For these biological entities, the lack of centrosymmetry may be due to the morphology as well as the chemistry. In Fig. 4A we can see calcite crystals at the corners of a crystal made of COD, whereas in Fig. 4B the photograph shows weddellite crystals deposited on the surface of anhydrous uric acid.
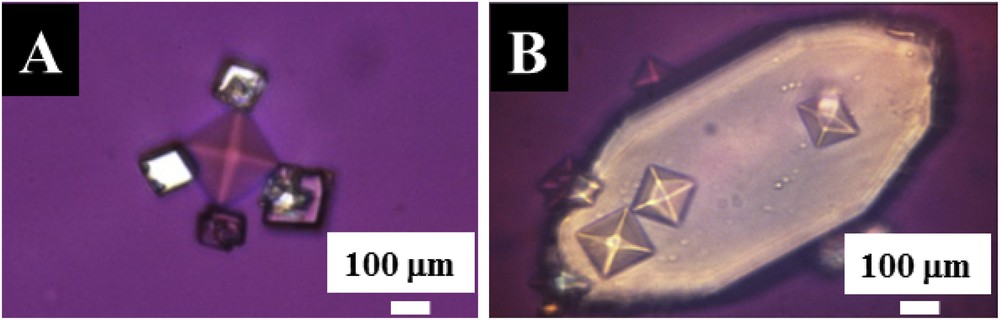
Crystals present in urine as Janus particles. (A) Calcite crystals at the corners of a crystal made of COD (B) weddellite crystals deposited on the surface of anhydrous uric acid.
4 Chemistry at the interface
A precise understanding of the pathogenesis of calcifications lies in the description of the interface. The observation that the nucleus of a kidney stone is often chemically different from the material on the surface led K. Lonsdale [30] to suggest that epitaxial relationships between crystalline phases may be an important factor contributing to their formation. The word epitaxy comes from Greek words taxis meaning in an orderly manner and epi meaning above. In the case assessed by K. Lonsdale [30], the term heteroepitaxy is therefore more appropriate. In heteroepitaxial growth, atoms of different species are deposited onto a substrate of different type (which may also be composed of various atomistic species).
Modelling heteroepitaxial growth is not an easy task. Such formalism has to take into account not only a match between interatomic distances, but also relaxation processes as well as interdiffusion phenomena. However, the study of interfaces in the presence of solvents at the ab initio level is becoming state-of-the-art in the field of material modelling; the study of adsorption of small biomolecules having been studied extensively [31]. Ab initio molecular dynamics has already been used successfully in several studies, even for very complex amorphous systems in water [32]. Modelling the interface – amorphous solid/(amorphous) liquid – implies that several difficulties have to be overcome. The special case of silica can be generalized to other interfaces showing water molecules or hydroxyl groups on their surface, the orientation of these groups depends on the local surface topology. However, in the presence of liquid water, only the strong inter-OH bonds are maintained in the case of silica, whereas the weaker ones are replaced by H-bonds formed with interfacial water molecules. Some other surface OH groups are simultaneously found to be H-bond acceptors or donors to water. Interestingly, we have found that the first water layer above the silica surface is overall rather disorderly and strongly adsorbed. This result was confirmed in another application, using amorphous silica [33,34].
Regarding the different chemical compounds identified in KSs, M.C. Frincu et al. [35] reported a comprehensive list of different heterogeneous mineral interfaces that are epitaxially matched. In our study, FE-SEM experiments have been performed in order to gather information on these biological interfaces. The first set of observations is performed on RP made of NaUr. At high magnification, we can see through FE-SEM the presence of small spherical entities at the extremity of NaUr needles (Fig. 5A). Complementary information is given by EDS (Energy dispersive spectroscopy) measurements regarding the elementary composition of these spherical entities and needles (Fig. 5B). The EDS spectrum indicates that spherical entities are made of Ca, P and O, while needles are made of Na, C, O and N, highlighting an interface between apatite and sodium urate. We have already shown that spherical apatite entities are built from an agglomeration of apatite nanocrystals [36–40]. As pointed out by C. Rey et al. [41,42], a remarkable characteristic of apatite is the existence of a hydrated poorly crystalline calcium phosphate region on the surface of apatite nanocrystals, which is at the core of protein/inorganic recognition and interaction. The case of an “amorphous” interface has not been really discussed in the paper of M. Crina Frincu et al. [35], although it is often found in biological materials.
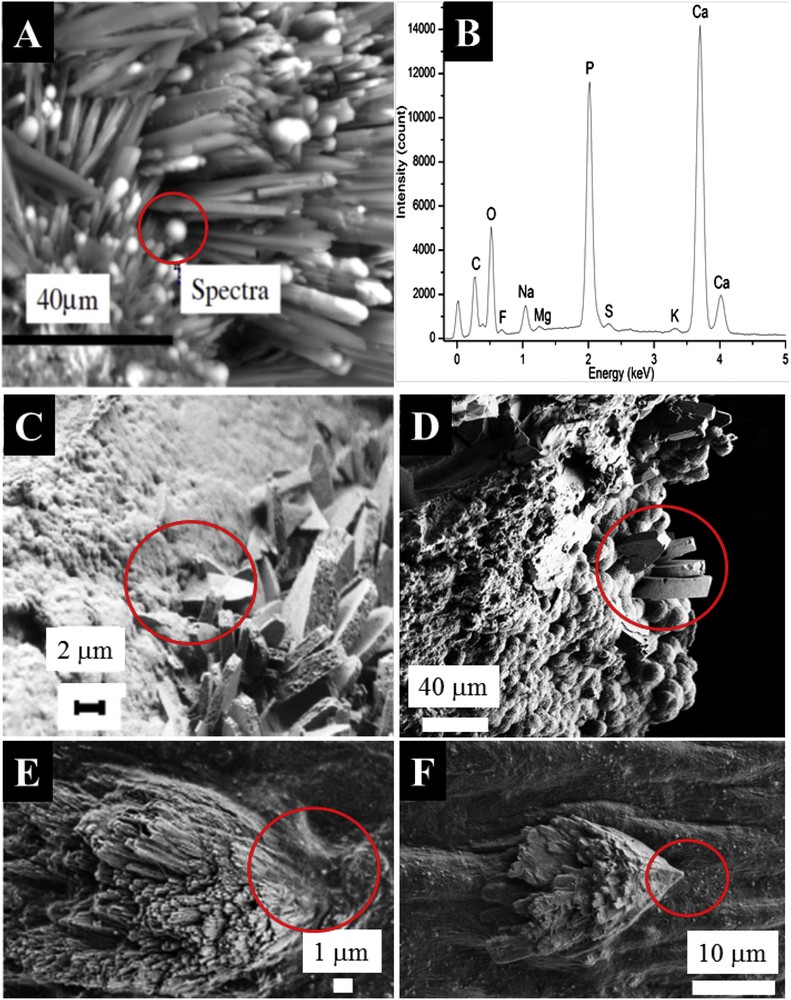
Specificity of the interface in the case of pathological calcifications. (A) RP made of NaUr. At the tip of the sodium urate needle, spherical entities made of CA are present. (B) EDX spectrum confirms the elementary composition of these spherical entities, (C and D) Calcium oxalate crystallites are engaged in a mix of proteins and Ca phosphate crystals in the case of RP (C) and a kidney stone made of Ca phosphate apatite (D). In the case of the cardiac valve (E and F), crystallites may be inserted in the tissue.
Moreover, in the case of KSs, the surface of the mineral cannot be considered as a “free surface”. Regarding calcium oxalate, which is the most common crystalline phase in urinary calculi, different papers have discussed the presence of osteopontin as a critical inhibitor on the surface of calcium oxalate crystals [42]. A. Okada et al. [44] identified osteopontin in calcium oxalate crystals by immunohistochemical staining. D.G. Reid et al. [45] used nuclear magnetic resonance spectroscopy for phosphatic stones to provide evidence of the close coexistence of biomacromolecules, especially glycosaminoglycans, with apatite. Very exciting results have been obtained also through the investigations of precipitation of mineral phases on organic monolayers [46,47], giving information on the growing process of the mineral phase. First, R. Backov et al. [46] demonstrated that domain boundaries within the monolayer might act as nucleation sites. Second, A. Uysal et al. [48] assessed the deformation of the COM mineral crystals at the early stage of the growing process in order to achieve a lattice match with the organic monolayer. Third, the final morphology of the crystal depends intimately on the protein. For example, boat-shaped crystals sometimes encountered in COM stones are observed in the presence of mucoproteins [49]. If these observations are not in line with approaches based on the similarity of interatomic distances between chemical phases identified in KSs, the complete set of data clearly underlines strong mineral/organic interactions during the biomimetic crystallization of COM.
In vitro experiments also provide valuable information about the nature of the interaction between mineral and organic phases [50–55]. Regarding the growth of hydroxyapatite on dipalmitoyl phosphatidyl choline monolayers, L.J. Zhang et al. [55] noticed that the hydroxyapatite crystallization process is controlled predominantly by electrostatic interactions between the template and hydroxyapatite. Other interactions may play a key role in the growing process, including hydrogen bonding, stereochemistry, and polarisation. For example, Z. Chen et al. [56] indicated that hydrogen bonds between the tea extract and calcium oxalate host crystals play a pivotal role in prohibiting COM formation and in the morphological control of COD crystals. J.J. De Yoero et al. [57] investigated the interactions of citrate with steps and faces on COM crystal surfaces and have provided links between the stereochemistry of interaction and binding energy levels. Such an approach allows the authors to underline mechanisms of growth modification and changes in the overall crystal morphology.
Finally, we have investigated the interface between a KS made of COM and a RP made of CA. Fig. 5C shows randomly oriented large COM crystals trapped in a phase of carbapatite crystals embedded in proteins acting as ‘glue.’ Such an interaction between COM crystallites and apatite was observed also in the case of KSs made of mixtures of COM and apatite without any RP (Fig. 5D). For such an interface, the hypothesis of heteroepitaxy between Ca phosphate (RP) and Ca oxalate (KS) is not relevant. Of note, in the case of ectopic calcifications present in cardiac valves, acicular crystallites have been observed which can be inserted in the tissue (Fig. 5E).
5 Bioinspired approach from pathological calcifications
We would now like to compare a classic microfluidic experimental set up with others, which can be inspired from pathological calcifications. As an example, we have selected the microfluidic device developed by R.A.L. Leon et al. [58]. These authors demonstrated the fabrication of engineered pharmaceutical formulations of drug(s) and excipient as monodisperse spherical micro-particles. More precisely, they fabricated monodispersed micro-particles (∼200 μm size) containing crystals of a hydrophobic model drug embedded within a hydrophilic matrix (here sucrose), which in turn may also contain a hydrophilic model drug (glycine). From an experimental point of view, this set up consists of three syringe pumps and two side-by-side capillaries placed in the centreline of an outlet tubing (Fig. 6A). The dispersed phases are injected into a continuous phase and biphasic droplets are generated at the tip of the capillaries.
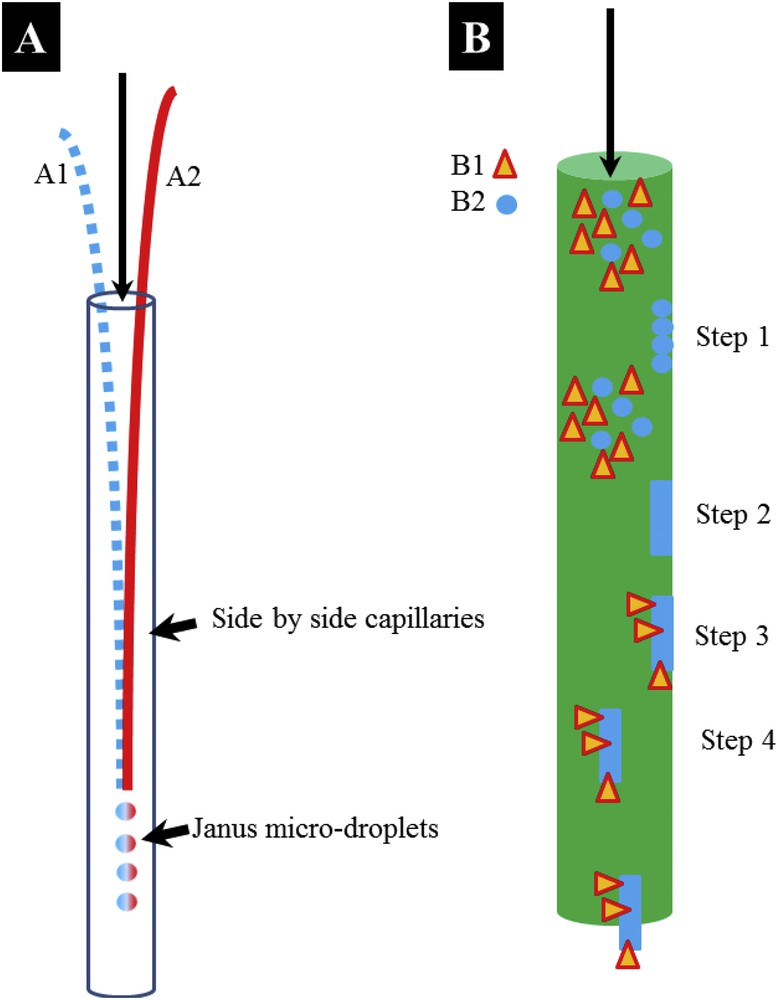
(A) Schematic representation of the microfluidic experimental set up developed by R.A.L. Leon et al. [58] (B) Design of a microfluidic experimental set up following the pathogenesis of a RP.
We have seen previously that whewellite kidney stones on the surface of which a RP is present display a peculiar interface. At this point, it is worth pointing out that the genesis of these ectopic calcifications takes place in the interstitium of the kidney, in the deep medulla, close to the papilla while other lithogenic processes involve calcium oxalate and/or phosphate formation in the tubules of the kidney, which have typical microfluidic dimensions. In Fig. 6B, we distinguish different steps in the genesis. As it is the case for the previous device (Fig. 6A), the two components B1 (anisotropic particle which can be made of COM) and B2 (spherical particle made of apatite and proteins) are mixed. The first difference arises with the agglomeration of spherical entities (B2) on the walls of the capillaries (step 1) and their agglomeration (step 2) to generate a pseudo RP. Then, a second difference results from the interaction between the two components (B1 and B2), such an interaction being similar to the ones described previously (Fig. 5C,D). Finally, we noticed that particles detach. Not only is this process (Fig. 5B) far more complex than a classic example (Fig. 6A), but it is also clear that the peculiar interaction between the two components B1 and B2 opens up new opportunities in chemistry. The morphology of the B1 component, which has to be associated with an acicular morphology, is now a key parameter to successfully generate particles that can be considered as Janus particles.
6 Conclusion
While physiological calcifications are often used to design new material, we tried to show in this contribution that it is possible to use the biological asymmetrical growth in pathological calcifications as inspiration in biomimetic strategies.
Acknowledgements
This work was supported by the Physics and Chemistry Institutes of CNRS and by contracts ANR-09-BLAN-0120-02, ANR-12-BS08-0022, ANR13JSV10010-01, convergence UPMC CVG1205 and CORDDIM-2013-COD130042.