1 Introduction
Polyphenol trans-resveratrol (trans-3,5,4'-trihydroxystilbene) is a famous member of the stilbene family as this compound has been associated with the “French paradox” (Fig. 1). Its daily consumption, for example, in the form of red wine [1], has been linked to beneficial effects in humans [2] and protection against coronary heart diseases [3]. First isolated from the white hellebore (Veratrum grandiflorum O. Loes) [4], it was then also found in Japanese knotweed (Polygonum cuspidatum syn. Fallopia japonica) [5], the current source for its industrial extraction in China. In grapevine leaves and berries, trans-resveratrol is a phytoalexin produced in response to stresses, such as wounding or pathogen attack [6] showing an antifungal activity against plant pathogens [7–11] or human pathogens [12]. In grapevine stem (wood) resveratrol is produced constitutively and acts as a phytoanticipin. In humans, resveratrol might play a role in preventing cardiovascular diseases [13]; it might also provide some protection against certain types of cancer [14], diabetes [15] and retard some neurodegenerative diseases [16]. In metazoans and mice, resveratrol has been demonstrated to extend lifespan by acting as a mimic-agent for the caloric restriction-longevity effect through sirtuin protein activation [17,18]. Nowadays, the main market for resveratrol is in the nutraceuticals sector using the Polygonum root as a source but some American companies have focused on grapevine as the raw material (Longevinex®). In the cosmetics field, the grapevine seems to be the most suitable raw material for resveratrol and its derivatives for products such as face creams [19,20].

Chemical structures of some hydroxystilbene monomers and dimers.
The role of resveratrol in skin protection has to be linked first to its well known antioxidant properties. For example, in a study evaluating the peroxydal scavenging activities of various wine polyphenolic monomers, resveratrol was found to be the strongest compound over catechin, epicatechin/gallocatechin and gallic acid/ellagic acid [21]. In the same way, a formulation containing 1% resveratrol (FAMAR, Athens, Greece) developed for Calidora Skin Clinics (Seattle, WA) has shown a 17-fold increase in antioxidant potency against a formulation containing 1% of the coenzyme Q analog idebenone using the ORAC test (Oxygen Radical Absorbance Capacity, Brunswick Laboratories, Norton, MA), the latter compound being recognized as the strongest topical antioxidant [22]. The resveratrol skin care formulation indeed yielded 4845 μmoles vitamin E equivalents/g against 279 for the 1% idebenone-containing formulation [23]. Another aspect of interest is the potential role of resveratrol and derivatives as whitening agents in cosmetology. Tyrosinase (monophenol, dihydroxyphenylalanine: oxygen oxidoreductase EC 1.14.18.1) is a catecholase capable of oxidizing ortho-diphenolic systems, namely dihydroxyphenylalanine (DOPA), which is known as the rate-limiting enzyme of melanin anabolism in melanocytes. Melanin provides protection against UV light-induced photoaging and photocarcinogenesis. It has indeed been reported that artificially enhancing melanin biosynthesis reduced the incidence of skin cancer in mice [24] and that the frequency of malignant melanoma was lowered in dark-skinned humans [25]. However, the overproduction and uneven deposition of melanin, which results in skin spots, can be the cause of esthetic problems. This justifies the research for cosmeceuticals capable of reducing or inhibiting melanin synthesis and accumulation in humans, that is, whitening agents used to decrease hyperpigmentation [26]. Resveratrol or analogs have long been described as potent inhibitors of the tyrosinase [27]. There are some reports of resveratrol activity as an inhibitor of that enzyme using either the mushroom or the human tyrosinase as a model enzyme [26,28–30]. However, opinions differ as to its mechanism of action on tyrosinase inhibition. Some studies suggest that resveratrol itself does not function as an inhibitor of tyrosinase but is rather oxidized by it [26,28]. The oxidation forms of resveratrol in turn become true inhibitors of tyrosinase activity playing the role of “suicide substrates” for this enzyme [26,28]. Resveratrol was thus suggested to act as a kcat type inhibitor for tyrosinase [28]. In other studies, resveratrol was characterized as a direct inhibitor of the tyrosinase activity of mushroom or humans with an IC50 of 57.05 μg/L on mushroom tyrosinase activity [29] and 0.39 μg/L on human tyrosinase activity [30], respectively. Whatever its mechanism of action on tyrosinase inhibition, these results suggest a possible usage of resveratrol as a tyrosinase and melanogenesis inhibitor as well as a whitening agent. However, if the antioxidant activity of resveratrol as well as its effects on tyrosinase inhibition are well demonstrated, other ways that may explain the resveratrol action on skin protection probably exist. Altogether, these results confirm the potential of resveratrol as a cosmeceutical.
Beside resveratrol, it is now well known that grapevine is able to produce a high diversity of molecules belonging to the stilbene family (Fig. 1). All these molecules are powerful antioxidants or at least are suspected to be more active than resveratrol itself but their extraction is rather difficult, likely explaining why they are not currently sold by chemical suppliers, except the dehydrodimer (+)-ɛ-viniferin. The biosynthesis of resveratrol is quite simple, involving four steps from phenylalanine or three steps from tyrosine as starting molecules, respectively (Fig. 2). The synthesis of viniferins by peroxydases, on the other hand, remains incompletely elucidated. The production of recombinant resveratrol is now a reality and many plants, bacteria or yeast [31,32] have been modified genetically in order to produce resveratrol for the assessment of its potential role in human health promotion and plant disease control. However, there is considerable interest in searching sourcing of resveratrol without recombinant genetic modification. In this context, plant biotechnology techniques could thus represent a powerful means for large-scale production of resveratrol and its derivatives. The use of plants also allows for low cost and rapid production of biologically active molecules in large amounts.
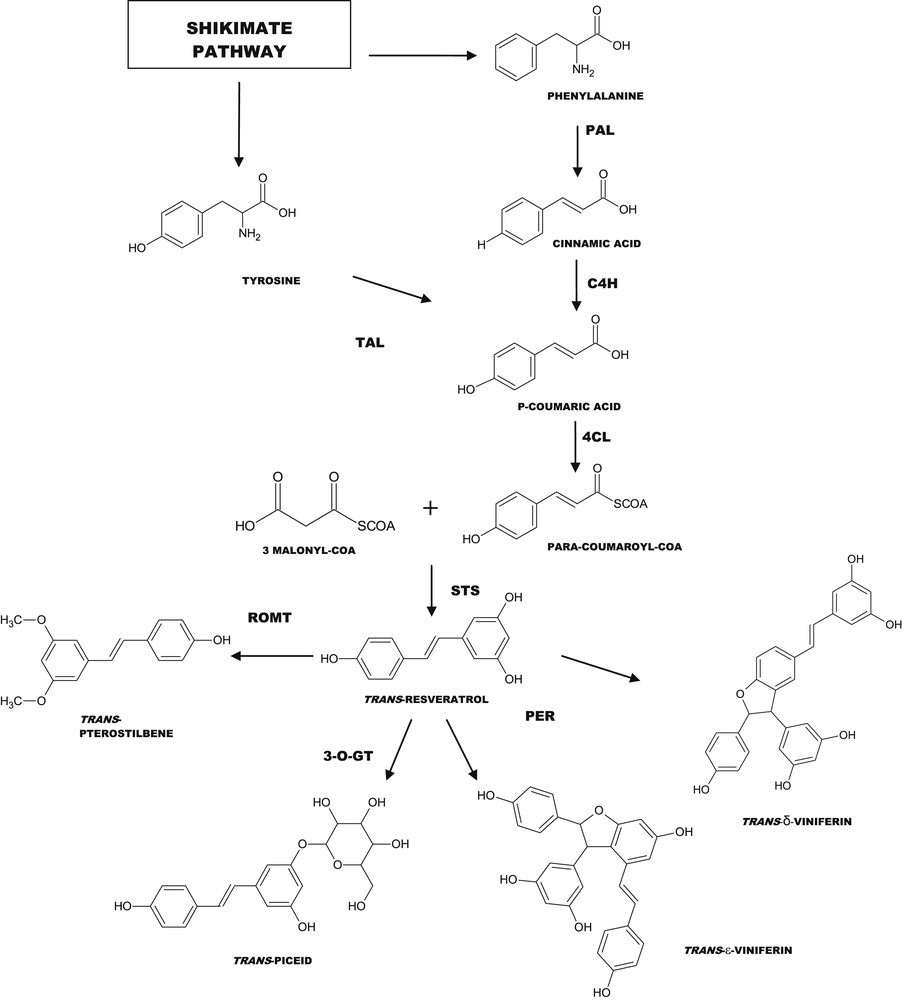
Biosynthesis of resveratrol and derivatives via the phenylalanine/polymalonate pathway. PAL, Phenylalanine ammonia lyase; TAL, Tyrosine ammonia lyase; C4H, Cinnamate-4-hydroxylase; 4CL, 4-Coenzyme A ligase; STS, Stilbene synthase; PER, Peroxidases; 3-O-GT, 3-Glucosyl-O-transferase; ROMT, Resveratrol-O-methyl transferase.
Since the 1980s, plant cell cultures have been used extensively to investigate the production of secondary metabolites under controlled conditions. The objective was to prevent intensive cutting and decimation of natural resources. For instance in the case of taxol, a potent anti-cancer drug isolated from Taxus brevifolia (Pacific yew tree), it should be noted that a sufficient dosage of this compound for one patient requires sacrificing two to four fully grown trees of this species when the bark is used for extraction. Additionally, the chemical synthesis of taxol is extremely complex (requiring 35 to 51 steps), with the highest total yield of the best synthesis of 0.4% [33 and references therein]. Plant cell cultures thus appear as a valuable alternative for the production of such a compound eliminating reliance on yew tree plantations. Moreover, plant biotechnology techniques avoid unsustainable extraction processes or chemical synthesis which include multiple steps and the use of polluting solvents and metal catalysts. The advantages afforded by in vitro plant cell culture systems also result from their potential low production costs (plant media only contain minerals, sugars and traces of growth regulators). In contrast, microorganisms need complex and costly fermentation media and engineering the entire metabolic pathways is needed [34]. Moreover, the axenic culture conditions afforded by plant cell systems allow one to avoid contaminants such as pesticides, fungi or heavy metals and offer the possibility for a continuous metabolite production. Although a basal production of secondary metabolites does exist in plant cell systems, elicitation has been shown as the most efficient way to induce the synthesis or to enhance the yield of various compounds of pharmaceutical interest such as alkaloids, terpenoids, phenolic compounds or heterosides [35,36]. Two plant cell model systems are currently under development through the use of undifferentiated cells (plant cell suspensions) or plant organ cultures (hairy roots obtained from Agrobacterium rhizogenes). Both culture systems typically take place in liquid media under aseptic conditions and are adaptable to their use in large-scale bioreactors [37]. Thereafter will be described plant cell systems usable for the production of resveratrol and related phytostilbenes.
2 General requirements for establishing cell suspensions for the production of phytostilbenes in shake flasks
Resveratrol and phytostilbene production has mainly been achieved using grapevine cell suspensions. To our knowledge, there is only one report of a resveratrol production by cell suspensions of other species, in cotton (Gossypium hirsutum L.) [38]. Plant cell cultures only require minerals, vitamins, sugars and minute quantities of growth regulators [39]. The media used for culturing grapevine cells are mainly the Murashige and Skoog medium [40,41–46], the Gamborg medium [47,41,48–57] and supplemented in some cases with vitamins of the Morel medium [58,41,49,51,54,56,59]. In all experiments, plant growth regulator combinations including auxins + cytokinins were used. Plant cell suspensions are rotary shaken (100–110 rpm) and maintained in total darkness [46,50,52–57,59], with a 16 h light/8 h dark and 14 h light/10 h dark cycle [44,48,49,51] or under continuous fluorescent light [41–43,45]. When discontinuous or continuous illumination is used, light can induce the biosynthesis of pigments such as anthocyanins, or the photoisomerization of trans-resveratrol to the cis-form, all by-products that will have to be removed during the purification process. Sucrose is generally added as the carbon source at a concentration of 10 g/L [44], 20 g/L [48,49,51,52,54–56], 30 g/L [46,53,57] or even 60 g/L [41,42]. In the latter case, such a high sucrose concentration has been suggested to constitute an osmotic stress capable of inducing the production of secondary metabolites [36].
3 Use of elicitors for the induction of resveratrol production in shake flasks
Resveratrol production by grapevine cell systems has mainly been achieved in Erlenmeyer flasks of 250 mL and in some cases in small working volumes of 20 mL with or without elicitors and cell suspensions are also able to synthesize resveratrol and its glucoside constitutively [38,41,43,60]. Nonetheless, most described experiments employed biotic agents, the so-called elicitors [61], for inducing phytostilbene biosynthesis in plant cell suspensions. Some of the used elicitors are signal molecules involved in many plant cell signaling pathways [61]. For instance, jasmonic acid (JA) which is implied in transduction cascades and its derivative, methyljasmonate (MeJA) have been used as inducers of hydroxystilbene production in various plant cell culture systems [42,44–46,51,52,54,55,62–66]. MeJA is indeed known to trigger overproduction of compounds of medical interest in cell suspensions: the anti-cancer drugs, taxol (paclitaxel) in T. brevifolia Pacific yew tree [64] and ginsenoides in Panax ginseng [65] or the synthesis of rosmarinic acid, a caffeic acid ester with potent applications for Alzheimer's disease in Coleus blumei cultures [66]. Unexpectedly, MeJA alone was found to be a low elicitor of phytostilbene production in grapevine cell suspensions. Moreover, it was also reported to decrease cell growth, this decrease being associated with a 20–60% lower biomass generation rate despite any effect on cell viability [46,50,54]. MeJA is generally added at doses ranging from 5 to 100 μM to grapevine (Vitis vinifera) cell suspensions resulting in low to moderate synthesis of resveratrol or its glucoside, piceid, in shake flasks (Table 1). For instance, 10–20 μM MeJA promoted the endogenous production of 24 μg/g FW resveratrol [44] and 120 μg/g FW piceid [45] in grapevine cell suspensions. The best elicting experiments with MeJA were obtained in cell suspensions of V. vinifera cv Monastrell with a resveratrol production of 3.74 mg/g DW using 100 μM MeJA [54] and in those of the cultivar Gamay Fréaux var Teinturier with a piceid production of 840 mg/L using 10 μM MeJA [42].
Plant cell systems in shake flasks for the bioproduction of phytostilbenes.
Plant | Elicitors | Produced stilbenes | References |
Vitis vinifera cv Gamay Fréaux var. Teinturier | None | 150 mg/L stilbene glucosides | [41] |
Vitis vinifera cv Gamay Fréaux var. Teinturier | None | 40 mg/L piceids | [60] |
Vitis vinifera cv Gamay Fréaux var. Teinturier | None | 330 mg/L stilbenes | [43] |
Gossypium hirsutum | None | 7.2 μg/g DW t-resveratrol | [38] |
Vitis vinifera cv Gamay Fréaux var. Teinturier | MeJA 25 μM | 200 mg/L piceids | [62] |
Vitis vinifera cv Cabernet Sauvignon Vitis vinifera cv Gamay Fréaux var. Teinturier | MeJA 25 μM MeJA 10 μM | 300 mg/L piceids 840 mg/L piceids | [42] |
Vitis vinifera cv Gamay Fréaux var. Teinturier | MeJA (20 μM) | 12 μg/g FW resveratrol and piceids extracellular 120 μg/g FW resveratrol and piceids Intracellular | [45] |
MeJA 20 μM + 20 g/L sucrose | 46 μg/g FW resveratrol and piceids extracellular 103 μg/g FW resveratrol and piceids Intracellular | [45] | |
Vitis vinifera cv Barbera | MeJA 10 μM | 23.94 μg/g DW resveratrol intracellular 7.98 μg/g DW resveratrol extracellular | [44] |
Roostock 41 B | MeJA 200 μM | 150 mg/L resveratrol | [46] |
Vitis vinifera cv Monastrell | MeJA 5 μM MeJA 100 μM | 798 μg/g DW resveratrol 3.74 mg/g DW resveratrol | [54] |
Vitis vinifera cv Alphonse Lavallée | MeJA 25 μM + low energy ultrasounds | Viniferins | [55] |
Vitis vinifera cv Cabernet Sauvignon | MeJA 100 μM + UV-C | 2 mg/g DW resveratrol intracellular | [63] |
Vitis vinifera cv Gamay | MeJA 100 μM | 20 mg/L resveratrol | [51] |
Vitis vinifera cv Italia | MeJA 25 μM JA 25 μM | 0.970 mg/g DW stilbenes 1.023 mg/g DW stilbenes | [52] |
Vitis vinifera cv Gamay Fréaux var. Teinturier | MeJA 10 μM + SA 500 mM + resin H2MGL | 2667 mg/L resveratrol | [53] |
Vitis vinifera cv Gamay Fréaux var. Teinturier | JA 10 μM + β-glucan + Amberlite resin | 2400 mg/L resveratrol | [67] |
Vitis vinifera cv Gamay | DIMEB 5 mM | 148–184 mg/L t-resveratrol | [48] |
Vitis vinifera cv Monastrell | DIMEB 50 mM HYPROBa50 mM CAVASOLb 50 mM | >4000 mg/L resveratrol 5000 mg/L resveratrol 5000 mg/L resveratrol | [69] |
Vitis vinifera cv Monastrell albino | DIMEB 50 mM HYPROB 50 mM | 3400 mg/L resveratrol 3000 mg/L resveratrol | [49] |
Vitis vinifera cv Gamay Rouge | DIMEB 50 mM HYPROBa 50 mM | 3000 mg/L resveratrol 990 mg/L resveratrol | [49] |
Vitis vinifera cv Gamay | Methylated CDs 50 mM | 900 mg/L resveratrol | [51] |
Vitis vinifera cv Monastrell | Undefined CDs 50 mM | 600 mg/L resveratrol | [54] |
V. riparia × V. berlandieri V. amurensis Vitis vinifera cv Merzling Vitis vinifera cv Pinot noir | DIMEB 50 mM DIMEB 50 mM DIMEB 50 mM DIMEB 50 mM | 911 mg/L resveratrol 225 mg/L resveratrol 4 mg/L resveratrol 0.5 mg/L resveratrol | [70] |
Vitis vinifera cv Monastrell albino | DIMEB 50 mM + MeJA 100 μM DIMEB 50 mM alone | 365 mg/g DW resveratrol 60 mg/g DW resveratrol | [50] |
Vitis vinifera cv Gamay | Methylated CDs 50 mM + MeJA 100 μM | 3100 mg/L resveratrol | [51] |
Vitis vinifera cv Monastrell | Undefined CDs 50 mM + MeJA 100 μM | 3000 mg/L resveratrol | [54] |
Vitis vinifera cv Monastrell | CAVASOLb 50 mM + ethylene 1 mM CAVASOLb 50 mM + SA 100 μM CAVASOL b50 mM + MeJA 100 μM+ ethylene 1 mM CAVASOLb 50 mM + MeJA 100 μM + SA 100 μM | 680 mg/L resveratrol 260 mg/L resveratrol 1540 mg/L resveratrol 1040 mg/L resveratrol | [68] |
Vitis vinifera cv Monastrell | CD 50 mM + 1 μM coronatine CD 50 mM + MeJA | 943 mg/L resveratrol 1600 mg/L resveratrol | [59] |
Vitis vinifera cv Negramo | Coronatine 10 μM | 35 mg/L resveratrol 210 mg viniferins/L | [57] |
Arachis hypogea Hairy roots | Sodium acetate 10.2 mM | 300–588 μg/g DW of root Resveratrol extracellular | [80] |
Vitis rotundifolia Hairy roots | MeJA 100 μM | <23 μg/g DW Resveratrol extracellular 1.81 μg/g DW ɛ-viniferin extracellular 182 μg/g DW Piceids intracellular | [81] |
a Cyclodextrin.
b Cyclodextrin.
Other signaling molecules such as salicylic acid (SA) and ethylene are also capable of inducing phytostilbene biosynthesis in grapevine cell suspensions. A hyper-elicitation of resveratrol production in the order of 2.7 g/L was obtained upon the addition of 10 μM JA and 500 μM SA together with a non aromatic resin dedicated to suppress the toxic effects of SA and JA in V. vinifera cv Gamay Fréaux var Teinturier cells [53]. A high resveratrol production of 2.4 g/L has also been reported in cell suspensions of the same cultivar combining treatment with a β-glucan, an Amberlite resin and 10 μM JA [67]. Finally, 1 mM ethylene combined with 50 mM cyclodextrins (see below) and 100 μM MeJA was shown to induce more than 1.5 g/L resveratrol in V. vinifera cv Monastrell cell suspensions [68].
Resveratrol and its derivatives are not readily soluble in aequous solutions as well as in cell suspensions. Moreover, phytostilbenes are subjected to oxidation and aggregation phenomena. In order to increase the solubility of these compounds and diminish subsequent degradation of the synthesized stilbenes, a number of studies have reported the use of cyclodextrins (CDs) in cell suspension systems [48–51,54,69,70]. CDs act as chelating agents, constituting a family of cyclic oligosaccharides composed of α-(1,4) linked glucopyrannose units [71]. CDs possess a cage-like supramolecular structure comprising a central hydrophobic cavity while the rims of the surrounding walls are hydrophilic (Fig. 3). Such a particular molecular architecture allows the formation of complexes with hydroxystilbenes increasing the solubility of the latter in cell suspensions and protecting them from oxidative degradation and aggregation phenomena [72]. Beside their role as drug carriers, CDs are also potent elicitors of resveratrol biosynthesis in grapevine suspensions alone or in combination with MeJA [48–51,54,68–70,73]. Various methylated β-cyclodextrins with methylation degrees ranging from 11 to 14 and used at 50 mM in grapevine cell suspensions were able to induce high resveratrol levels from 3 g to 5 g/L [69] (Table 1). The influence of the genotype on the response to elicitation with CDs was directly evidenced in the work of Zamboni et al. [70], the non-vinifera genotypes producing 60–1800 times more phytostilbenes than vinifera genotypes. CDs tested as elicitors of hydroxystilbene biosynthesis against MeJA, JA or SA generally showed the strongest activity (Table 1). Moreover, in contrast to MeJA, they did not affect cell viability or cell growth [48–50]. CDs possess structural motifs which resemble the alkyl-derivatized oligosaccharides produced during pectin hydrolysis by fungal pathogens [49], thus explaining their eliciting activity on phytostilbene biosynthesis in plant cell suspension systems.

Schematic representation of a cyclodextrin.
The best eliciting conditions for the production of phytostilbenes by cell suspensions consist of the use of methylated CDs in combination with MeJA. Some studies have indeed reported the synergistic effect of MeJA and CDs in their ability to induce namely the biosynthesis of taxol and related taxanes in cell cultures of Taxus species [74] as well as the resveratrol bioproduction in grapevine cell suspensions [50,51,54]. Though a weak to moderate extracellular accumulation of this compound was observed in V. vinifera cell suspensions of the cv Gamay as a response to 100 μM MeJA or 50 mM of a methylated CD, respectively 20 mg/L and 900 mg/L, a high resveratrol production (3.1 g/L) was obtained with those two elicitors used in combination [51]. In the same way, 50 mM DIMEB ((heptakis [2,6-di-O-methyl]-β-cyclodextrin) in combination with 100 μM MeJA induced a 5-fold increase in the resveratrol biosynthesis by grapevine cell suspensions of the cv Monastrell albino (365 mg/g DW) as compared to the obtained 68 mg/g DW in response to the cyclodextrin alone [50]. Recently, coronatine, a phytotoxin from Pseudomonas syringae acting as a mimic molecule of the isoleucine conjugated form of JA [75] and known for its ability to induce phytoalexin biosynthesis in rice [76], was shown to stimulate at a concentration of 1 μM in combination with 50 mM CDs, a production of around 0.94 g/L resveratrol in cv Monastrell grapevine cultures [59]. However, this resveratrol production was lower than that induced by the combination of methyljasmonate and a cyclodextrin (1.6 g/L). Ten fold-higher concentrations of coronatine (10 μM) induced similar effects on resveratrol biosynthesis in cv Negramaro grapevine cells and importantly the production of intracellular viniferins [57].
4 Transfer from shake flasks to bioreactors
Transfer of the production of resveratrol by the means of biotechnological methods from the laboratory to the industrial scale relies on the use of cell suspensions in bioreactors. Typically the capacity of the bioreactors ranges from 1 L to over 75,000 L as those used for the large-scale production of the anti-cancer drug, paclitaxel (taxol) by Phyton Biotech (www.phytonbiotech.com). Although most experiments describing resveratrol or phytostilbene synthesis in grapevine cell cultures take place in shake flasks of 20–250 mL with 10–100 mL volumes of cell suspensions [41–46,48–55,60,62,63,68–70], there are only few reports of production of those compounds in bioreactors [43,46,60,77–79]. The main apparatus employed include classical configurations such as stirring tanks [43,46,60,77] and V-shaped or cylindrical bubble columns [78,79]. Besides the question of the bioreactor design, the transfer from flasks to bioreactors requires the optimization of a lot of parameters such as 1) the sucrose concentration which typically ranges from 20 to 60 g/L [41,42,44,46,48,49,51–57], 2) the speed of agitation in the case of stirred tanks, 50 rpm [46], 75 rpm [77] and 100 rpm [60], 3) the density inoculums, and 4) the aeration rates fixed at 0.075 to 2 vvm [43,78,79]. In our own experiments, we observed that grapevine cells from the roostock 41 B (V. vinifera cv Chasselas × V. berlandieri) only tolerated low aeration and agitation rates, respectively, 0.025 vvm and 50 rpm [46]. This latter remark underlines the fact that transferring plant cell suspensions from shake flasks to bioreactors raises several issues which depend on the cultivated species or even on the used cell line. Although a constitutive production of the resveratrol glucoside piceid was already reported in bioreactors with Gamay Fréaux cell suspensions [60] (Table 2), the other experiments were conducted using elicitors or signaling molecules, mainly, MeJA and CDs alone or in combination. The maximal stilbene production utilizing the combination CDs + MeJA expressed as the resveratrol production in mg/g FW multiplied by the maximal biomass in grams obtained in a given system can reach the high value of 7.03 g/L resveratrol in cell suspensions of the variety Gamay in a stirred tank [78], 3.3 g/L in a V-shaped bubble column [78] and 6 g/L in a bubble column cylindrical with a three cycle-one stage culture system [79]. Here again a synergistic eliciting effect between CDs and MeJA was observed as the combination of these two elicitors resulted in a 4–6-fold increase in the resveratrol production (Table 2). The quantities of resveratrol recovered in bioreactors are thus in the same order, a few grams per liter, as those obtained in shake flasks using the best eliciting combinations (CDs + MeJA). Chitosan, which is a β-1,4-linked N-glucosamine polymer of the cell wall of numerous phytopathogenic fungi, was also found to induce the bioproduction though low of phytostilbenes, reaching 48 mg/L, in liquid cultures of V. vinifera cv Barbera petiole cells in a 1L-bioreactor at a concentration of 50 mg/L [77].
Resveratrol production in bioreactors.
Type of bioreactor | Working volume (L)/Grapevine cv | Aeration rate (vvm) | Maximal Biomass (g FW/L) | Produced stilbenes/Elicitor | References |
Stirred bioreactor | 15/Gamay Fréaux | 0.2 | 269 | 30 mg/L piceidsa None | [60] |
Stirred bioreactor | 2/Roostock 41 B | 0.025 | 546 | 209 mg/L resveratrol/MeJa | [46] |
Stirred bioreactor | Not given/Gamay Fréaux | 0.075–0.15 | 518 | 280 mg/L stilbene monomersa/None | [43] |
Stirred bioreactor | 0.8/Barbera | 0.2 L O2/min | 40 | 48 mg/L resveratrol, piceid resveratrolosidea/Chitosan | [77] |
Bubble column V-shaped | 1.1/Gamay | 0.95 | 246 | 3.3 g/L resveratrola/CDs + MeJA 0.5 g g/L resveratrola/CDs | [78] |
Stirred bioreactor | 1.1/Gamay | 2 | 521 | 7 g/L resveratrola/MeJA + CDs 1.6 g/L resveratrola/CDs | [78] |
Bubble column cylindrical | 5.8 L × 3/Gamay | 0.47–0.54 | 183 | 6 g/L resveratrola/MeJA + CDs | [79] |
a Maximal biomass (g FW/L) × Stilbene production (mg/g FW).
5 Resveratrol production in hairy roots
Hairy root cultures have also been used for stilbene production. This valuable system for the production of plant secondary metabolites [36] is obtained through the transformation of plant tissues by transferring the bacterial plasmid Ri T-DNA from A. rhizogenes, which causes a genetic modification leading to the development of roots. These roots are able to grow in liquid media and produce secondary metabolites. For the production of resveratrol, hairy root cultures of peanut (Arachis hypogea) were elicited with sodium acetate (10.2 mM) leading to a low production of extracellular resveratrol (300–588 μg/g DW of root) (Table 1) [80]. Recently, hairy roots of Muscadine grape (Vitis rotundifolia Michx.) were obtained in which an extracellular production of resveratrol (<23 μg/g DW; <100 nmol/g DW) and ɛ-viniferin (1.81 μg/g DW; <4 nmol/g DW) as well as an intracellular production of piceids of 182 μg/g DW (467 nmol/g DW) following elicitation by 100 μM MeJA [81] were characterized.
6 Conclusion
Resveratrol is a secondary metabolite which could be of great interest in the field of cosmetology given its antioxidant and anti-aging properties as well as its action on skin as a whitening agent. There is thus the need for resveratrol production methods using sustainable sourcing and without the use of genetically-modified organisms. Grapevine cell suspensions have been reported to produce resveratrol and derivatives, mainly its glucoside piceid and one of its dimeric compound, ɛ-viniferin. Most resveratrol production assays have been conducted in shake flasks and resveratrol was synthesized constitutively or as a response to elicitor treatments. Impressive levels of 3–5 g resveratrol per liter have been observed in shake flasks upon treatment with methyljasmonate/cyclodextrins in combination or cyclodextrins alone. One question still remains on how the transfer from shake flasks to bioreactors can be achieved. There are only few experiments reporting the transfer of the resveratrol production from small working volumes (10–100 mL of plant suspensions) to bioreactors (1–15 L of plant suspensions). However some results have suggested that resveratrol production in bioreactors is feasible with very good yields up to 7 g/L. In most experiments, resveratrol was described as being mainly excreted in the extracellular medium, meaning that its purification can be easily performed using apolar solvents such as ethyl acetate. Piceid remains within the cells and its extraction will need a greater number of steps. Elucidation of the resveratrol excretion-mechanisms through the plant cell wall, which are still not very well known, might thus be of help in further enhancing its production/secretion in the culture medium.