1 Introduction
Biocatalysis is now an essential tool for chemical industry and is the core of industrial biotechnology. Biocatalysts such as enzymes or whole cells are currently employed in chemical processes as an alternative to chemical catalysts [1] and applications of biocatalysis to produce fine chemicals especially for the pharmaceutical industry are now developed [2] as green chemistry processes [3] for the preparation of non racemic compounds. Hydrolytic enzymes, which usually display high efficiencies and functional specificities, are among some of the most exploited enzymatic catalysts used for biotransformations [4]. The most important method to produce a single enantiomer in industrial synthesis remains the resolution of racemates [5]. Lipases (triacylglycerol hydrolases, EC 3.1.1.3) are considered to be particularly efficient due to a broad substrate scope, excellent stability, and by displaying high regio and stereoselectivity in various media. They have been used in a wide range of industrial applications under mild and environment friendly conditions [6].
Lipases offer the advantage of accessing a wide range of possible reactions [7] and are thus convenient for obtaining optically enriched compounds from their racemic form by kinetic resolution [8]. In lipase kinetic resolution involving an enzyme, various parameters have to be taken into account for controlling both reactivity and enantioselectivity. A variation of the reaction parameters can induce a large change, depending on the microenvironment of the enzyme. For instance, in the kinetic resolution of a racemic alcohol with a lipase, the parameters such as the nature of the enzyme [9], additives [10], residual water in the reaction media [11], solvent [12], amount of lipase [13] and nature of acylating agent [14] are often examined for the optimization of selectivity.
Alcohols are often conveniently resolved through lipase-catalyzed acylations through an irreversible reaction [15] by the use of suitable acylating agents such as enol esters [16] or anhydrides [14b,17]. The choice of the acyl donor allows to improve the enantioselection in the production of optically active compounds. The chain-length of substrate and its effect on the selectivity of various lipases has been studied in a number of works [18]. The effect of alcohol chain length on enzymatic esterification is the most studied. However, there are still a few investigations regarding the structure of the acyl group in both transesterification and acylation reactions [19].
Kazlauskas' rule predicts which enantiomer will react faster in the acylation of secondary racemic alcohols. The stereo-preference for the (R)-enantiomer is observed for the hydrolysis of esters or acylation of alcohols [20]. Enzymatic hydrolysis and transesterification reactions can thus be complementary processes for the resolution of secondary alcohols, and when the selectivities of the transesterification and hydrolysis are high (E > 100), alcohols and acetates enantiomers are obtained with high enantiopurity [21]. However, the influence of the migrating group on enantioselectivity in hydrolysis and acylation reaction catalyzed by lipases has been rarely studied in the literature. We have thus investigated the selectivity of three lipases according to the acyl moiety of the acyl donor using: (i) the enzymatic acylation of 1-phenylethanol by six various enol esters (ii) the hydrolysis of the corresponding phenylethylesters with the same lipases (Scheme 1).
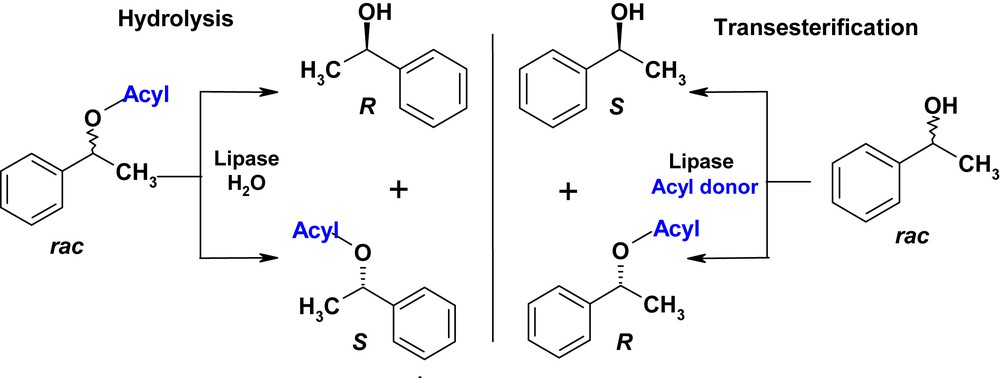
Enantiocomplementarity of hydrolysis and transesterification reactions.
The acyl donor can influence the enantioselectivity of the lipase by its acyl moiety or the alkyl moiety. Some studies justified this behavior on the basis that the enzyme would be imprinted by the migrating group during the formation of the acyl-enzyme intermediate and influencing thus the selectivity of the enzyme [22]. This substrate matching strategy is described as a new approach for enhancing effectively the lipase enantioselectivity in organic solvents [22a]. A combination of molecular modeling and kinetic studies allowed to build models that explain and predict the enantioselectivity of lipases [23], and structural factors improving affinity enzyme-substrate and influencing enantioselectivity have been identified [24].
We report here a study of the effect of the acyl moiety of enol esters 2a–f on the selectivity of the lipase in the acylation of 1-phenyl ethanol 1 as a model substrate. We compare these effects to those observed for the hydrolysis reaction of the corresponding ester 3a–f with the same lipase (Scheme 2).
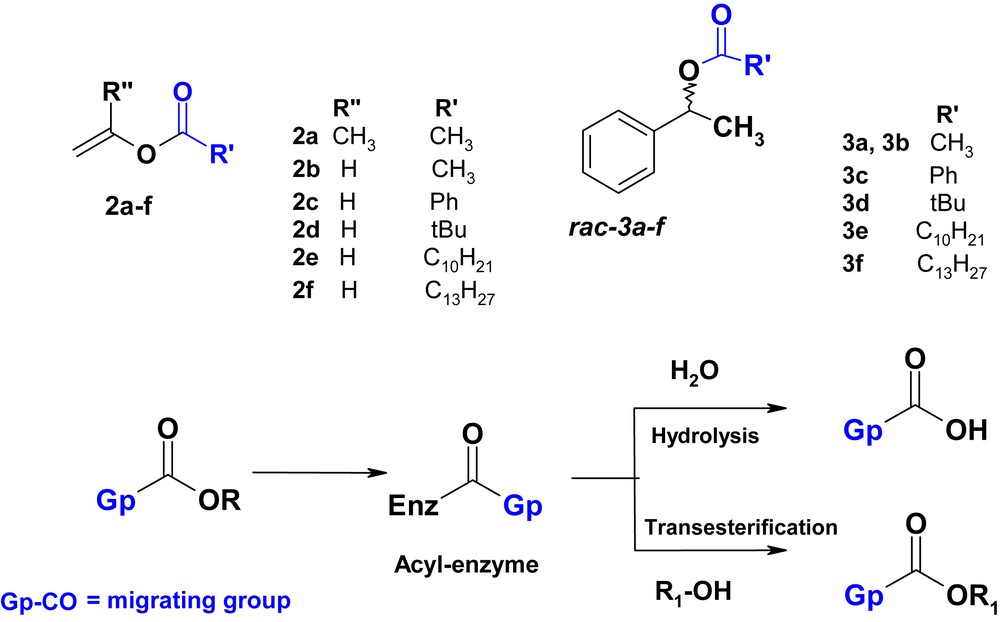
Lipase-resolution by acylation by 2a–f and by hydrolysis of 3a–f.
2 Results and discussion
In this study, activities and enantioselectivities of three different microbial lipases frequently used in enzymatic resolution were screened: immobilized Candida antartica lipase-type B (CAL-B), Pseudomonas cepacia lipase (PCL), and Candida cylindracea lipase (CCL). Six enol esters of various structures (isopropenyl acetate, vinyl acetate, vinyl pivalate, vinyl benzoate, vinyl decanoate, and vinyl laurate) were selected as acylating agents of (R,S) 1-phenylethanol. Phenylethylesters 3a–3f are hydrolyzed with the same lipases. The goal is to determine their affinity to the substrate relative to the acyl moiety in the acyl donor or in the racemic esters. It is interesting to determine the influence on the selectivity and reactivity of lipase and the impact on both acylation and hydrolysis reactions.
2.1 Lipase-catalyzed transesterification of 1-phenylethanol with enol esters 2a–f
We have examined the effect of enol esters 2a–f on the activity and selectivity of three lipases on 1-phenylethanol (Scheme 3). The enzymatic-acylation is carried out with 1 mmol of 1-phenylethanol, 3 mmol of enol ester 2a–f in 6 mL of diethyl ether at 40 °C for 24 h. The unreacted alcohol and the acetate were separated by silica-gel flash chromatography. The ee's of both compounds are measured before separation through analysis by GC or HPLC on a chiral phase. Results of transesterification of 1-phenylethanol by vinyl esters are collected in Table 1.
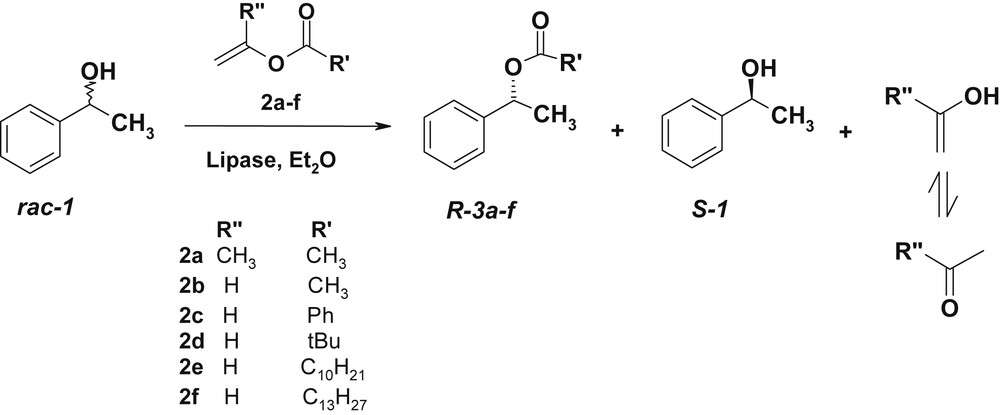
Vinyl ester reagents for the lipase-catalyzed resolution of rac-1.
Lipase-catalyzed transesterification of 1-phenylethanol with vinyl esters 2a–f.
Entrya | Lipase (mg) | Acyl donor | eesb (S)d (yield)c | eepb (R)d (yield)c | C(%)b | Eb |
1 | Candida antarctica lipase B (20) | 2a (Isopropenyl acetate) | 99 (44) | 99 (48) | 50 | >200 |
2 | 2b (Vinyl acetate) | 99 (43) | 98.7 (50) | 50 | >200 | |
3 | 2c (Benzoate) | — | — | — | — | |
4 | 2d (Pivalate) | — | — | — | — | |
5 | 2e (Decanoate) | 99 (42) | 95.6 (44) | 50.8 | >200 | |
6 | 2f (Laurate) | 99 (45) | 96.3 (43) | 50.7 | >200 | |
7 | Pseudomonas cepacia lipase (20) | 2a (Isopropenyl acetate) | 11.4 (85) | 99 (09) | 10.3 | >200 |
8 | 2b (Vinyl acetate) | 44 (58) | 98.5 (28) | 31 | 148 | |
9 | 2c (Benzoate) | — | — | — | — | |
10 | 2d (Pivalate) | 2.2 (95) | 98.7 (2) | 2.2 | 111 | |
11 | 2e (Decanoate) | 99 (49) | 99 (48) | 50 | >200 | |
12 | 2f (Laurate) | 99 (42) | 98.7 (48) | 50 | >200 | |
13 | Candida cylindracea lipase (80) | 2a (Isopropenyl acetate) | 8.9 (57) | 50 (10) | 15 | 3 |
14 | 2b (Vinyl acetate) | 13 (76) | 51.3 (19) | 20.2 | 5 | |
15 | 2c (Benzoate) | 52.4 (55) | 78.8 (38) | 40 | 16 | |
16 | 2d (Pivalate) | 0.04 (95) | 1.5 (2) | 2 | 1 | |
17 | 2e (Decanoate) | 10.8 (75) | 77.8 (11) | 12.2 | 9 | |
18 | 2f (Laurate) | 8.5 (72) | 81.4 (6) | 9.4 | 11 |
a Each experiment was carried out with 1 mmol of racemic 1-phenylethanol and 3 mmol of acylating agent in diethyl ether (6 mL) at 40 °C for 24 h.
b Measured by chiral chromatography, GC or HPLC. Conversion: C = eeS/eeP + eeS; selectivity: E = ln[(1 − C) (1 − eeS)]/ln[(1 − C) (1 + eeS)] [25].
c Isolated yields after silica gel chromatography, hexane/ethyl acetate 90:10.
d Absolute configuration of the products was assigned through comparison of the sign of the optical rotation with the literature data.
Results of Table 1 show that the conversion and enantioselectivity of the kinetic resolution of 1-phenylethanol with vinyl esters 2a–f depends on the lipase and the enol ester. High selectivities are observed with CAL-B and PCL for the vinyl decanoate and vinyl laurate (E > 200, C = 50%) (entries 5,6 and 11,12). With these acylating agents, CCL shows low activity (9.4% < C < 12.2%) and selectivity (9 < E < 11) (entry 17,18). With isopropenyl acetate and vinyl acetate, the selectivities are very high E > 100 (entries 1,2 and 7,8) but with different reactivities. The conversion is C = 50% for CAL-B but decreases with PCL (10% < C < 31%). CCL is active 15% < C < 20% and nonselective (E < 5) with these enol esters (entry 13,14). The vinyl pivalate is inactive with CAL-B lipase (entry 4), while low activity but selectivity are observed with PCL (C = 2% E = 111, entry 10). CCL is neither active nor selective (C = 2%, E = 1) with this acylating agent (entry 16). No reaction was observed with vinyl benzoate using CAL-B (entry 3) and PCL (entry 9), but with CCL, we obtained a good conversion C = 40% with moderate selectivity E = 16 (entry 15).
According to Kazlauskas' rule [20a], the R-enantiomer reacts faster in the kinetic resolution of secondary alcohols by lipases for substrates with a small and a large substituent (Scheme 4). In all cases, the R-enantiomer was acylated faster by the three studied lipases.
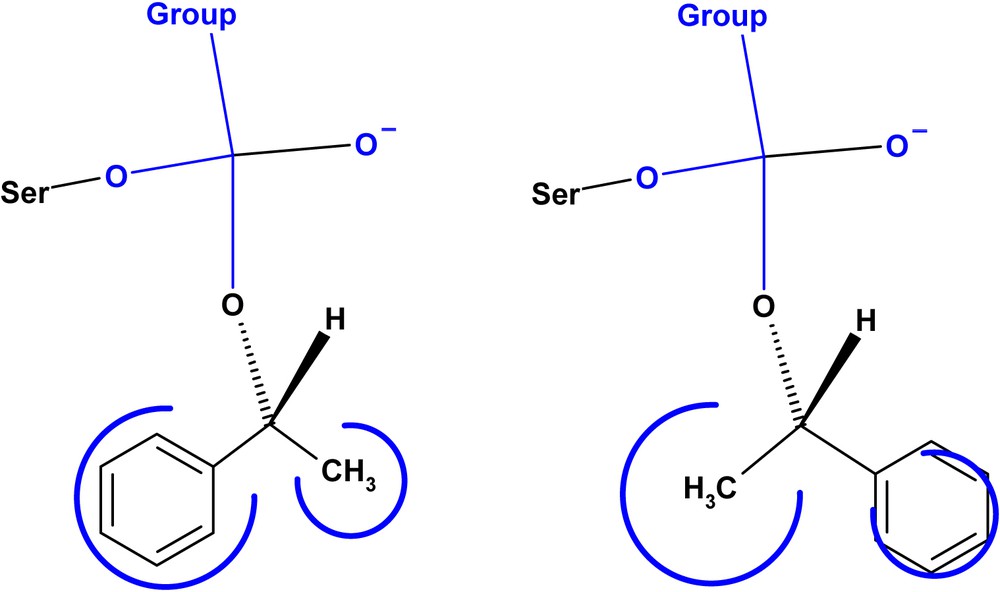
Enantio-preference for lipases with secondary alcohols.
These results show that the structure of vinyl esters significantly affects the reactivity and the selectivity of the lipases. In the case of vinyl pivalate and vinyl benzoate, steric hindrance of the esters probably inhibits the formation of the acyl enzyme. The origin of the enantioselectivity depends on the stereo-pocket structure whose shape can be different according to the lipase [26]. The stereopreference of the lipase from P. cepacia is determined predominantly by the substrate structure and the stereoselectivity depends on atomic details of interactions between substrate and lipase. A simple model has been found by Schulz et al. [27] to predict the enantioselectivity of P. cepacia lipase toward a broad range of secondary alcohols. This model is based on the HNε–Oalcohol hydrogen bond distance in an average minimized structure for the slow enantiomer. Another explanation would be that the energy curve of enzyme/substrate interaction is different between R and S, showing a different accessibility.
2.2 Enzymatic hydrolysis of racemic esters 3a–f
The hydrolysis of ester bond is catalyzed by lipases [28]. This reaction is part of a complementary process of acylation in the kinetic resolution of secondary alcohols [13b]. We investigated the hydrolysis of phenylethylesters 3a–f (1-phenyl-ethyl acetate 3a, 1-phenyl-ethyl benzoate 3c, 1-phenyl-ethyl pivaloate 3d 1-phenyl-ethyl decanoate 3e, 1-phenyl-ethyl laurate 3f) to examine the influence of the acyl part in the hydrolysis with the same lipases we used in the enzymatic acylation CAL-B, PCL and CCL (Scheme 5). The hydrolysis reaction was performed with 1 mmol racemic ester dissolved in 2 mL solvent, which was added to a buffer solution (pH = 7) with the appropriate amount of lipase.
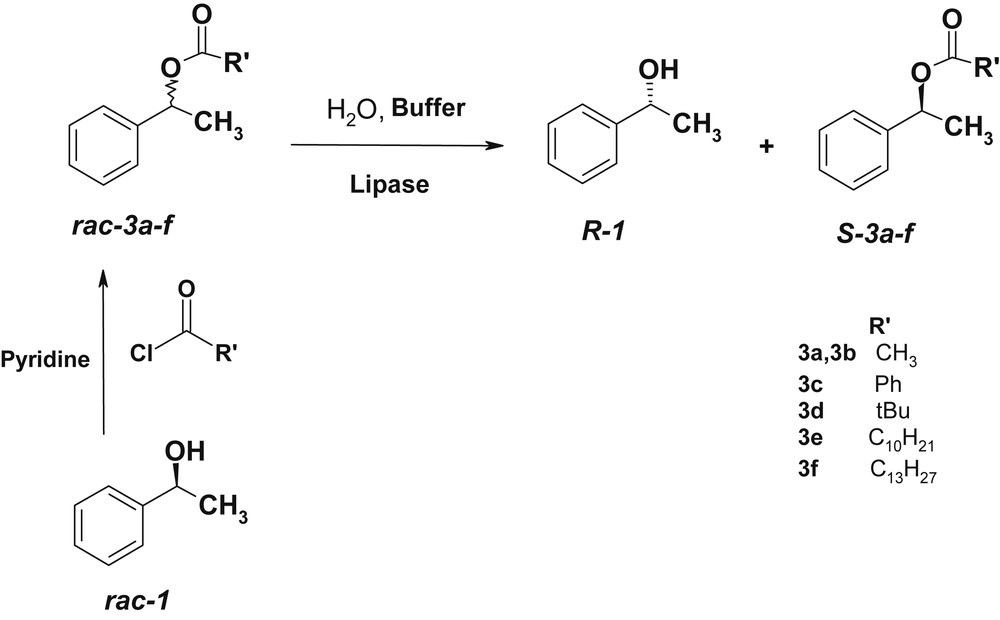
Lipase-catalyzed hydrolysis of racemic esters 3a–f.
The results are reported in Table 2.
Lipase-catalyzed hydrolysis of racemic esters 3a–f.
Entrya | Lipase (mg) | Substrate | eesb (Yield)c | eepb (Yield)c | Cb (%) | Eb |
1 | Candida antarctica lipase B (20) | 3a (Acetate) | 96 (36) | 99 (42) | 49 | >200 |
2 | 3c (Benzoate) | 6 (78) | — | — | — | |
3 | 3d (Pivalate) | 0.6 (45) | 0.6 (50) | 52 | 1 | |
4 | 3e (Decanoate) | 43 (43) | 98 (21) | 30 | 150 | |
5 | 3f (Laurate) | 99 (33) | 97 (12) | 51 | >200 | |
6 | Pseudomonas cepacia lipase (20) | 3a (Acetate) | 67 (30) | 99 (28) | 40 | >200 |
7 | 3c (Benzoate) | 7.4 (78) | — | — | — | |
8 | 3d (Pivalate) | 6 (23) | 4.6 (56) | 58 | 1 | |
9 | 3e (Decanoate) | 23 (67) | 99 (14) | 19 | >200 | |
10 | 3f (Laurate) | 24 (76) | 99 (15) | 20 | >200 | |
11 | Candida cylindracea lipase (80) | 3a (Acetate) | 14 (78) | 54 (20) | 21 | 4 |
12 | 3c (Benzoate) | 14 (64) | 91 (9) | 13 | 24 | |
13 | 3d (Pivalate) | 6.5 (2) | 0.1 (67) | 98 | 1 | |
14 | 3e (Decanoate) | 47 (51) | 57 (43) | 45 | 6 | |
15 | 3f (Laurate) | 35 (60) | 86 (24) | 29 | 19 |
a Each experiment was carried out with 1 mmol of racemic esters 3a–f in buffer solution and diethyl ether at 40 °C for 24 h.
b Measured by chiral chromatography, GC or HPLC. Conversion: C = eeS/eeP + eeS; selectivity: E = ln[(1 − C) (1 − eeS)]/ln[(1 − C) (1 + eeS)] [25].
c Isolated yields after silica gel chromatography.
Analysis of the results in Table 2 shows that the activity and selectivity of the CAL-B lipase in hydrolysis of racemic esters are highly comparable to those observed in the acylation reaction, except 3d where reactivity increased in hydrolysis (Table 1). The results in Table 2 show that the activities and selectivities of the three lipases in the hydrolysis of racemic esters are related to the effect of the migrating group structure. With CAL-B and PCL, the derivatives 3a, 3e and 3f are hydrolyzed with high selectivities (E > 150) but PCL is less active than CAL-B for the hydrolysis of the three substrates; the conversion decreases respectively from C = 49% to C = 40% for 3a (entry 1 vs. 6), from C = 30% to C = 19% for 3e (entry 4 vs. 9) and from C = 51% to C = 20% for 3f (entry 4 vs. 9). CCL is active but not very selective for the hydrolysis of 3a (C = 21%, E = 4) (entry 11), 3e (C = 45%, E = 6) (entry 14) and 3f (C = 29% E = 19) (entry 15).
In the hydrolysis of 1-phenyl-ethyl benzoate 3c with CCL, the selectivity obtained is E = 24 for a conversion of C = 13% (entry 12), CAL-B and PCL are inactive with this ester derivative (entries 2 and 7). The three lipases are very active without any selectivity for the hydrolysis of 1-phenyl-ethyl pivaloate 3d, C = 52% and E = 1 for CAL-B (entry 3), C = 58% and E = 1 for PCL (entry 8), C = 98% without selectivity (E = 1) for CCL (entry 13).
The reactivity of CCL is better over the two other lipases for the hydrolysis of all substrates, albeit much less selective. For an irreversible hydrolysis procedure, the fast reacting enantiomer is (a) and the slow reacting one is (b) in the active side model for lipases derived from Kazlauskas' rule (Scheme 4). It is noted that the three lipases exhibit R-enantiopreference esters in accordance with the literature [29].
The results of this study can be explained by taking into account the structure of lipase binding sites. It is established that the length, the form and the binding site of the lipases vary considerably according to their nature. The lipases from Candida antarctica, P. cepacia have a funnel-like binding site, larger funnel for PCL and narrower for CAL-B, but for CCL, it is a tunnel-like binding site [30]. Thus, the enantioselective pocket of CAL-B and PCL are tailored to accept linear substituents, such as decanoate or laurate, which can easily penetrate the funnel. Moreover, these two lipases can adapt to accept a variety of similar ester forms. According to form, size and substitution, the insertion of such substrates is more difficult and activity of the lipase is lowered in acylation as well as in hydrolysis. For CCL, the long and narrow site will affect the insertion of the substrate at the active site.
We observed the affinity of three lipases relative to the impact of the migrating group of the ester derivatives on vinyl esters in acylation or on the corresponding racemic phenylethylesters in hydrolysis. It is interesting to note that the lipases studied display different selectivities and reactivities in these two complementary reactions (acylation/hydrolysis). The results can be correlated to the three-dimensional shape of each lipase and conditions of each reaction.
3 Conclusion
We compared the structural and environmental parameters governing the enantioselectivity of three lipases CAL-B, CCL and PCL for resolving chiral secondary alcohols by acylation with vinyl esters of different lengths and shapes, and by hydrolysis of the corresponding racemic esters for a better understanding of the phenomena involved in both reactions.
The results show the strong influence of the migrating group of the acyl donor. There is a significant difference in reactivity and selectivity of the lipases according to the structure of the acylating agents. This can be attributed to their respective paths to the active site. CAL-B is highly selective in the transesterification of 1-phenyl ethanol with IA, VA, VD, VL (E > 200), while vinyl benzoate and vinyl pivalate do not react. With CAL-B, 1-phenyl-ethyl acetate, 1-phenyl-ethyl decanoate and 1-phenyl -ethyl laurate are hydrolyzed with high selectivities (E > 150).
Comparison of the reactivity and selectivity of lipases for acylation and hydrolysis shows, for the first time, many similarities for the two reactions, which depend on the structure of the migrating group. C. antarctica lipase B is by far the most effective in both reactions. This enantiocomplementarity offers a very interesting additional catalysis tool; the strategy can be adapted to the desired enantiomer.
4 Experimental section
4.1 General
NMR spectra were performed with Bruker spectrometers (300 MHz for 1H, 75 MHz for 13C). Chemical shifts were reported in d ppm from tetramethylsilane with the solvent resonance as internal standard for 1H NMR and chloroform-d (d 77.0 ppm) for 13C NMR. The enantiomeric excesses (ees) were determined by gas chromatography (ThermoFinnigan Trace GC) equipped with an automatic autosampler and using a CHIRALSIL-DEX CB column (25 m; 0.25 mm; 0.25 μm), or by a chiral stationary phase HPLC on Chiralcel-ODH column using racemic compounds as references. Retention times are reported in minutes. C. antarctica lipase fraction B immobilized on acrylic resin (CAL-B; LA > 10,000 U/g), P. cepacia lipase (PCL; LA > 30,000 U/mg), and C. cylindracea lipase (CCL; LA = 3.85 U/mg) were from Sigma-Aldrich. Vinyl esters 2a–f and both enantiomers of 1-phenylethanol 1 are commercially available (Aldrich). The organic solvents were dried over molecular sieves prior to use.
4.2 General procedure for the synthesis of racemic acetates 3a–3f
The acetates 3a–b were synthesized by chemical acetylation via the corresponding racemic alcohol (1 mmol), using 1.5 mmol of anhydride acetic, 1.2 mmol of Et3N, and a catalytic amount of 4 dimethylaminopyridine (0.1 mmol) in 4 mL of ether. The alkyl esters 3c–f were prepared from 1-phenylethanol (10 mmol) and pyridine (12 mmol) in dry diethyl ether (10 mL) and cooled (0 °C) in an ice bath, the acyl chloride (12 mmol) is added dropwise and the mixture was stirred at room temperature for 24 h. Next, the reaction mixture was diluted with diethyl ether and washed with 0.1 M aqueous HCl, saturated NaHCO3, and finally with brine. The ether solution was dried over MgSO4. The solvent was evaporated and the crude product was purified by column chromatography (silica gel, petroleum ether/ethyl acetate, 9:1). The ester derivatives were obtained with good yields (72% < yields < 99%). The 1H and 13C NMR spectra of these products were in good agreement with the literature.
4.3 General procedure for enzymatic acylations
The selected enzyme was added to a solution of racemic 1-phenylethanol (1 mmol) and the vinyl ester 2a–f (3 mmol) in diethyl ether (5 mL), and the mixture was stirred at 40 °C for 24 h. The reaction mixture was filtered on Celite and concentrated in vacuo. The (S)-1 phenylethanol and the corresponding (R)-ester were separated by flash chromatography on silica gel (petroleum ether/ethyl acetate: 90/10) and analysed by chiral HPLC or GC.
4.4 General procedure for enzymatic hydrolysis of racemic esters
One mmol of racemic esters of 1-phenylethanol 3a–f was dissolved in 2 mL of ether and added to 4 mL of phosphate buffer pH 7. The reaction was initiated by the addition of lipase and the mixture was shaken at 300 rpm at 40 °C for 24 h. The reaction mixture was filtered on Celite. The alcohol formed and the remaining ester were separated by aqueous base-organic solvent liquid–liquid extraction and finely by flash chromatography on silica gel (petroleum ether/ethyl acetate: 90/10) and analysed by chiral HPLC or GC.
4.5 Chiral GC analysis and/or Chiral HPLC analysis
Retention times are reported in minutes. The conditions for the analysis of alcohols (R)-1–9 and acetates (S)-1a–9a are reported below.
(R,S)-1-Phenylethanol 1: GC (Chiralsil-Dex CB): tR = 3.9 min, tS = 4.1 min (Tcolumn = 140 °C, flow 1.2 mL/min).
(R,S)-1-Phenylethyl acetate 3a,b: GC (Chiralsil-Dex CB), tS = 2.9 min, tR = 3.2 min (Tcolumn = 140 °C, flow 1.2 mL/min). Eluent: petroleum ether–AcOEt: 8/2.
(R,S)-1-Phenylethyl benzoate 3c: HPLC (Chiralcel OD-H, hexane/EtOH: 95/5, flow 1.0 mL/min): tS = 4.69 min, tR = 5.26 min. Eluent: petroleum ether–AcOEt: 9/1.
(R,S)-1-Phenylethyl pivaloate 3d: HPLC (Chiralcel OD-H, Isohexane/iPrOH: 90/10, flow 1.0 mL/min): tS = 7.68 min, tR = 9.37 min. Eluent: petroleum ether–AcOEt: 9/1.
(R,S)-1-Phenylethyl decanoate 3e: HPLC (Chiralcel AD-H, isohexane/iPrOH: 98/2, flow 1.0 mL/min): tS = 4.23 min, tR = 5.06 min. Eluent: petroleum ether–AcOEt: 9/1.
(R,S)-1-Phenylethyl laurate 3f: HPLC (Chiralcel AD-H, isohexane/iPrOH: 98/2, flow 1.0 mL/min): tS = 3.98 min, tR = 4.57 min. Eluent: petroleum ether–AcOEt: 9/1.
Acknowledgements
The Algerian Ministry of Higher Education and Scientific Research (FNR 2000 and PNR) and the Fund for Scientific Research (FNRS) are gratefully acknowledged for financial support of this work. The Catholic University of Louvain is acknowledged for a grant “Cooperation development” awarded to Nedjma Melais.