1 Introduction
2,5-Dihydroxybenzaldehyde (2,5DHB) is found in olive processing effluents [1] and in Beta vulgaris seeds [2]. 2,4-dihy-droxy-benzaldehyde (2,4DHB) is found in the heartwood of Toxicodendron vernicifluum [3], in red wines aged in acacia barrels [4] or in the aerial parts of Cassia grandis [5]. Both compounds showed bactericidal activities against Campylobacter jejuni, Escherichia coli, Listeria mono-cytogenes, and Salmonella enteric [6]. They can also act as sensitizers of antifungal agents [7,8] and as antioxidants [3,9–11].
The antioxidant activity is typically measured far to the physiological conditions. For example, in the DPPH method (scavenging of α,α-diphenyl-β-picrylhydrazyl free radicals) the medium is methanol, and electrochemical measurements using the H2O2 oxidation on mercury electrodes are made in the pH range of 10–10.5 due to restrictions of the electrode [9–11].
The dissociation state of phenolic benzaldehydes and gallic acid derivatives [12] is crucial to understand their chemical and bacteriological activities that take place under physiological conditions. However, only one reference in the literature deals with the value of the higher dissociation constant of gentisaldehyde [13]. Only the main maximum wavelengths of the UV spectra in methanol have been reported for both derivatives [14].
The aim of this paper was, first, to obtain the dissociation constants of the 2,4DHB and 2,5DHB to show the relevance of the dissociation of the OH groups in the interpretation of the antioxidant activities. To reach this goal, the UV spectra of the aldehydes must be investigated in order to obtain their dissociation constants and antioxidant activities are measured at two pH values.
2 Experimental
2,5DHB and 2,4DHB were from Sigma and the rest were from Merck (both analytical quality). Supporting electrolytes used for the determination of the antioxidant activity were solutions of 0.1 M in both sodium bicarbonate, at pH = 10.5, and 0.1 M phosphoric acid at pH = 12.5. The ionic strength was adjusted to 0.5 M with solid KNO3 and the pH was adjusted with solid NaOH. Antioxidants were dissolved in ethanol and the stock solution concentrations were 5 × 10−3 M. These solutions were stored in darkness at 277 K to avoid decomposition.
UV–vis absorption spectra (190–800 nm) were recorded with a double beam Perkin–Elmer Lambda 750S spectrophotometer with Hanna quartz cuvettes of 1 cm pathlength. Solutions of 0.1 M in both phosphoric and acetic acids for pH < 8.5 and 0.1 M in both sodium bicarbonate and phosphoric acid for pH > 8.5 were used. pH was measured using a Metrohm pH-meter. Alkali error was corrected with the correction curves supplied by the manufacturer. Ultrapure water was obtained with a Millipore-Milli Q system.
A CHI650A electrochemical workstation from IJCambria coupled to an EF-1400 controlled growth mercury electrode from BAS instruments was used in the HMDE mode. The Hg drop area was 6.70 × 10−3 cm2. The temperature was kept at 298 ± 0.1 K. All potentials were measured against an Ag|AgCl|KClsat electrode (BAS MF-2052). A platinum counter electrode BAS MW-1034 was used. Differential pulse voltammetry (DPV) experiments were made with a pulse amplitude of 0.05 V, a pulse width of 0.05 s and a pulse period of 0.2 s. The antioxidant activity was determined as reported [10,11].
To ensure the reproducibility of the measurements, the experiments were made repeatedly, and the standard deviations of the data were less than 5%.
3 Results and discussion
The antioxidant activity was obtained from the decrease of the DPV signal corresponding to the H2O2 oxidation on a mercury electrode in the presence of antioxidants [10,11]. This assesses the interaction with the radicals produced during the oxidation of H2O2, being a measurement of the scavenging ability. The measurement pH must be 10 or higher, because at lower pH values the oxidation of the mercury electrode prevents the appearance of the H2O2 signal. The volume that decreases the area of the H2O2 oxidation peak in 10% is related to the scavenging activity. Its inverse was selected to express the antioxidant activity because the higher the values of this parameter the higher the scavenging activity [11].
Fig. 1 shows the DP voltammograms of the H2O2 oxidation after the addition of one of 2,4DHB at the two pH values used. The experiments were made at a constant ethanol content of 30%, both in the absence and in the presence of antioxidants, to avoid the effect of this variable on the DPV peak and to ensure the proportionality between the peak area and the H2O2 concentration [10,11].
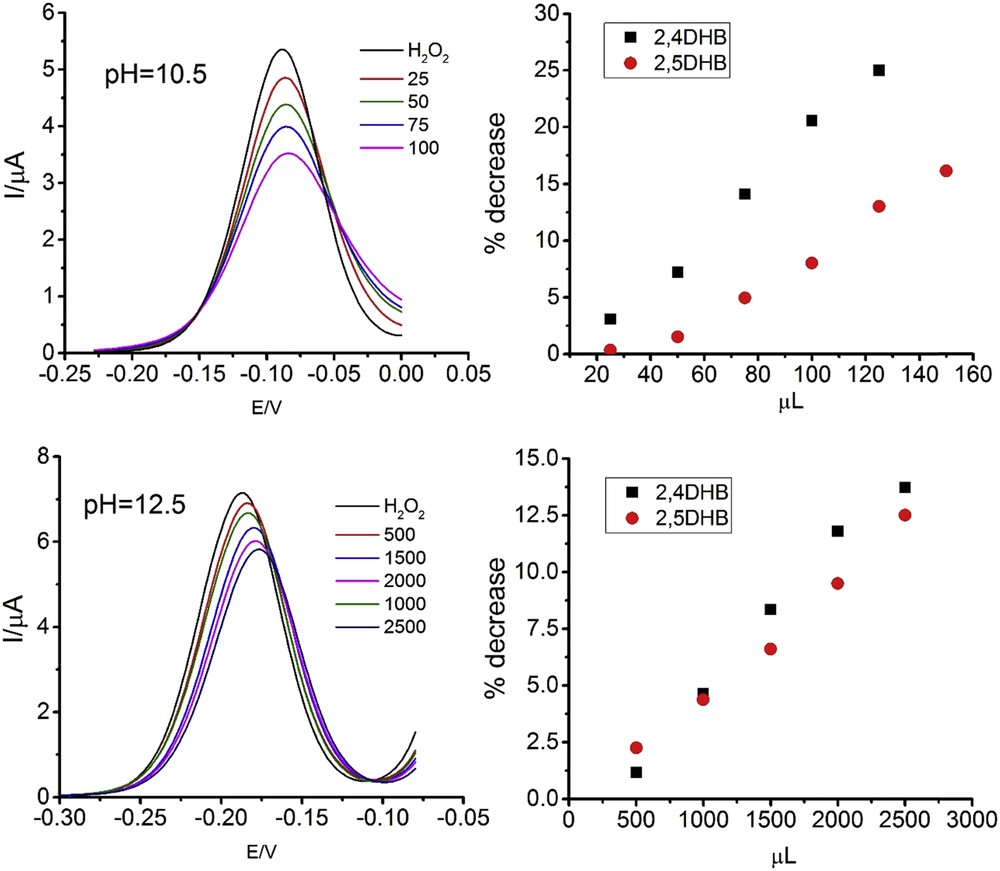
Left: DPV of 5 × 10−4 M H2O2. Numbers correspond to microliters of 5 × 10−3 M 2,4DHB in 10 mL of final volume. Right: Decrease of the DPV peak area with the added amounts of 5 × 10−3 M antioxidant solution at pH: (up) 10.5, (down) 12.5.
The oxidation peak decreases as the antioxidant concentration increases at both pH values but in the most alkaline medium higher volumes of antioxidants are needed to produce the decrease for the three compounds. Fig. 1 also shows the percentages of the area diminution.
At pH 10.5, the antioxidant activity of 2,4DHB is ca. double than that measured for the 2,5DHB, whereas at pH 12.5 the antioxidant activities of both compounds are similar, though slightly lower in the case of the 2,5 isomer. Proton dissociation is important to understand structure-activity relationships of gallic acid derivatives with antioxidant activity [12]. The above changes in the antioxidant activities, both absolute and relative, could be discussed in light of the dissociation of the hydroxyl groups. To assess this, the knowledge of the pK values is essential. The complete pK values of the dihydroxy-benzaldehydes are not reported in the literature, as stated in the introduction, and this will be the following task to do.
Fig. 2 shows the absorption spectra as functions of the acidity for both dialdehydes. The curves showed several main bands whose intensities depended on pH of the solution.
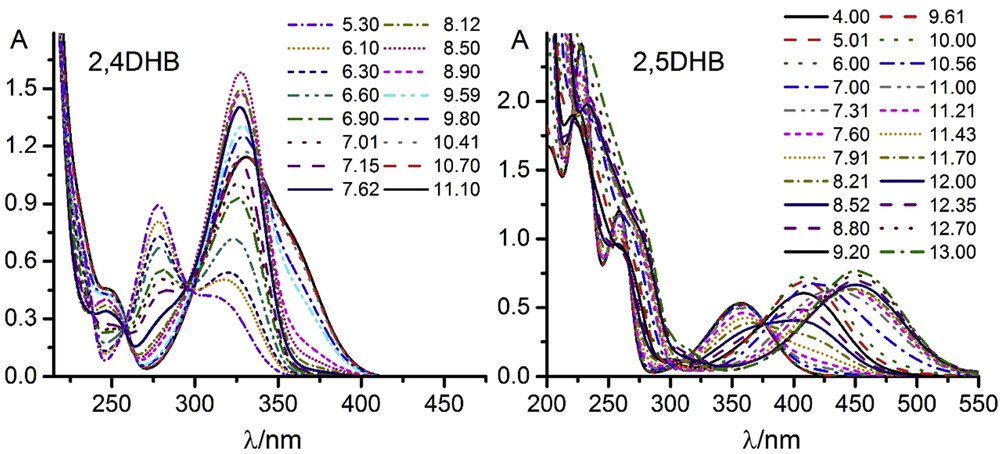
UV–visible spectra. c = 1 × 10−5 M.
The pH dependencies of the spectra indicate the occurrence of acid-base reactions that must correspond to the dissociation of the hydroxyl groups of the molecules:
The 2-hydroxyl group can form an intramolecular H-bond with the adjacent carbonyl group [15] that stabilizes the OH. For this reason, the more plausible dissociation sequences are those shown in the above scheme.
The absorption spectra were analyzed by using a computer program developed for Microsoft Visual Basic 2013, based on other programs from our laboratory and previously used in other pK determinations [16,17]. In this program, it was assumed that the bands can be represented by a log-normal band shape, which can be described by the equation [18]:
The spectra were decomposed into sets of bands integrated by the minimum number of bands possible, and with the condition that the band parameters were independent of both the pH and concentration (with the obvious exception of the absorbance). Fig. 3 shows the comparison between theoretical and experimental results for both compounds at low and high pH values.
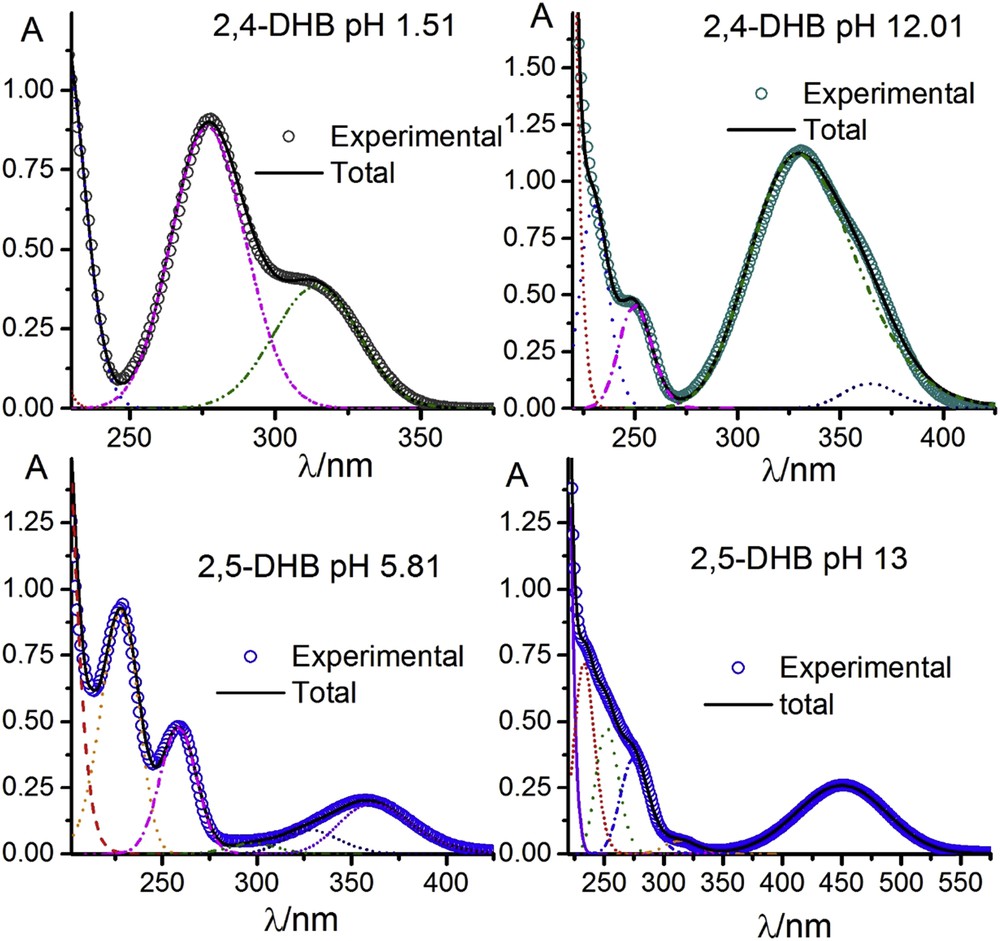
Fitting of the lognormal plots to experimental spectra.
The intensities of some of the bands presented variations with the pH (either from zero to a maximum value, either from a maximum value to zero), or present maximum values at intermediate pH values and are zero at very lower or higher values. The parameters of such bands are shown in Table 1. These parameters remained virtually unchanged in the pH range studied. Fig. 4 shows the dependence of the maximum absorbances with the pH.
Characteristic parameters of the main individual bands.
Compound | λ/nm | Δν1/2/kK | b |
2,4-DHB | 276.5 | 3.8 | 0.1 |
313.3 | 3.8 | 0.15 | |
248.0 | 3.0 | 0.0 | |
327.0 | 3.3 | 0.16 | |
357.0 | 3.0 | 0.0 | |
2,5-DHB | 258.0 | 3.3 | 0.15 |
361.0 | 3.4 | −0.05 | |
420.0 | 3.4 | −0.1 | |
452.0 | 4.4 | 0.22 |
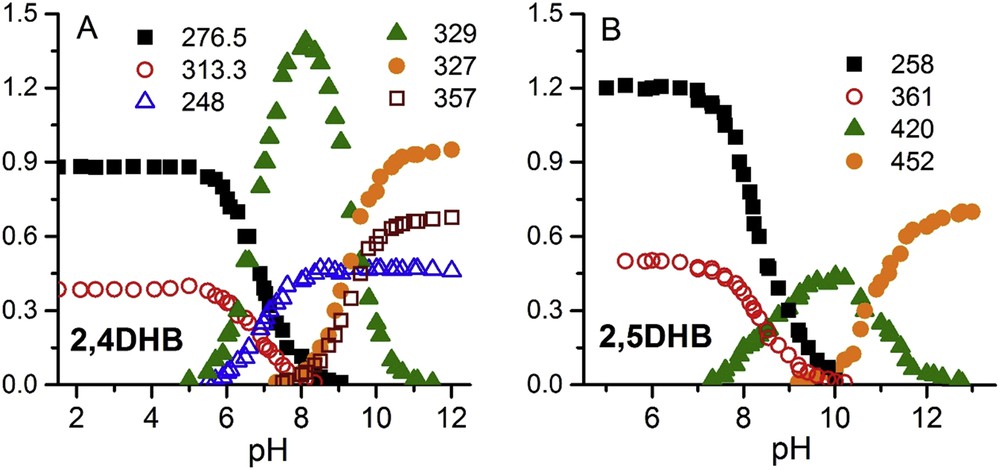
Maximum absorbances of fitted bands versus pH.
If the pH dependence of the maximum absorbance of the individual bands is due to the abovementioned acid-base equilibria, the following trivial equation must be fulfilled:
The knowledge of the pK values permits us to calculate the percentage of the different species at each pH. In all cases, the lower the pH, the higher the antioxidant activity found; that is, the occurrence of OH groups is related to high antioxidant activity. At pH 10.5, the hydroxyl group in position 4- of 2,4DHB is fully dissociated and the hydroxyl group in position 2- is dissociated in ca. 94%. For 2,5DHB, the hydroxyl group in position 5- is fully dissociated and the hydroxyl group in position 2- is dissociated in ca. 37%. So, the dianionic form of 2,4DHB is approximately 2.5 times more abundant than the dianionic form of 2,5DHB at this pH value. At pH 10.5, the antioxidant activity of the 2,4DHB is ca. double than that of 2,5DHB. At the most alkaline pH (12.5), the two OH groups of 2,4DHB are fully dissociated and ca. the 97.5% of 2,5DHB is dianionic. The antioxidant activity of this last compound at pH 12.5 is only slightly lower than that corresponding to 2,4DHB.
The above results show that the pH of the assays for antioxidant capacity is very important in light of the OH group dissociation, because of the different dissociation constants of the isomers. So to know the possible antioxidant action of a given molecule in a living organism, the assay must be made the closest possible to physiological conditions, that is, in aqueous media with a medium-high ionic strength and at pH 7–7.5, as is made in the CUPRAC-AOA assay [19].
The antioxidant capacity of phenolic derivatives is intrinsically related to the number of hydroxyl groups in the molecule. When the number of OH in the molecules is the same, the extension of the dissociation of each group is essential. Undoubtedly, the radical scavenging capacity of polyphenols depends on the interplay of other factors, other than the acidity, such as redox potential of free radicals, ionization potentials of phenol anions and ability of the molecules to form a hydrogen bond. In addition, the concentrations of hydroperoxide and superoxide anion radicals generated in the oxidation of H2O2 on the mercury electrode depend on pH. So, the measurement of scavenging ability of the DHB acid gives different absolute values at the pH 10.5 and 12.5, but the relative antioxidant activities are related as discussed above.
Acknowledgments
Financial support from Córdoba University within the framework of the “Ayudas puente para el desarrollo de proyectos de I+D precompetitivos 2015” is acknowledged.