1 Introduction
There are few reports on additions of amino acid derivatives to azodicarboxylates to afford 1,2,4-triazolines or their saturated precursors, 1,2,4-triazolidines. Thus, oxidation of N-acyl substituted amino acid esters under the Mitsunobu reaction conditions has been reported to proceed through an enolate addition to diisopropylazodicarboxylate (DIAD), at the crucial step. The resulted intermediate upon cyclization leads to the final 1,2,4-triazolidines and 1,2,4-triazolines [1]. 1,2,4-Triazolines are also formed during the phosphine mediated annulations of N-protected imines with diethyl azodicarboxylate (DEAD) [2]. In the presence of a base, the 1,3-dipolar cycloaddition of glycine ester aldimines leads to 1,2,4-triazolines through dehydrogenation of the non-isolated 1,2,4-triazolidine primary adducts [3]. Aldimines of phenylglycine esters [4] and benzylamine [5] react in a 1,3-dipolar cycloaddition manner with DEAD and afford 1,2,4-triazolidines. 1,2,4-Triazolidines are formed via a retro-1,3-dipolar cycloaddition of imidazolidines in the presence of DEAD [6]. Finally, the nonstabilized azomethine ylides generated from the desilylation of N-[(trimethylsilylmethyl]iminium triflates undergo, in the presence of CsF, a 1,3-dipolar cycloaddition reaction and afford 1,2,4-triazolidines and 1,2,4-triazolines [7]. N-substituted amino acids react in a decarboxylative 1,3-dipolar cycloaddition with DEAD to afford 4N-substituted-1,2,4-triazolidines [8].
The 1,2,4-triazoline and 1,2,4-triazole moiety is found in biologically active compounds with various activities such as antimicrobial, antifungal, antitubercular, anti-HIV, anti-inflammatory, and antioxidant activities [9]. Therefore there is a continuous need for new derivatives having structural differences in terms of substitution patterns that might affect the biological activity.
We have reported recently on the 1,3-dipolar cycloaddition of sulfide containing imines of glycine imines to N-phenylmaleimide [10] and fullerene-[60], C60 [11], and the antioxidant activity for some of the cycloadducts. Encouraged by the above results and having an interest in the chemistry and synthetic applications of N-phenyltriazolinedione (NPhTAD) [12], a cis-locked cyclic diazene [13], we decided to test it as a dipolarophile in a dipolar cycloaddition reaction with the above imines of glycine esters. Triazolinediones [14] are very reactive electrophiles that are used in ene [15], Diels–Alder [16], aromatization [17], polymer modification [18], and initiation of radical addition [19] reactions, together with their extensive use in the synthesis of polyureas [20]. In addition, the urazole ring of PhTAD in the addition products can be transformed to an azo or amino group [21].
Lipoxygenases are a family of non-heme iron-containing dioxygenases [22]. LOXs are connected with several inflammation related diseases such as allergic asthma, cancer, cardiovascular, and skin diseases [23]. There is also increasing interest in antioxidants due to prevention of harmful effects of free radicals in human body [24]. Therefore in an attempt to extend our biological interest to this new group of compounds, we decided to test most of them for their anti-lipid peroxidation activity and as possible lipoxygenase inhibitors.
2 Results and discussion
Having in mind the possible synthesis of sulfide substituted triazolidines or triazolines, we were surprised to find that imine of glycine ester 1a, react with PhTAD at room temperature in PhMe to afford α-amination adduct 2a after 24hr, Scheme 1, albeit in a very low yield, ∼3%.
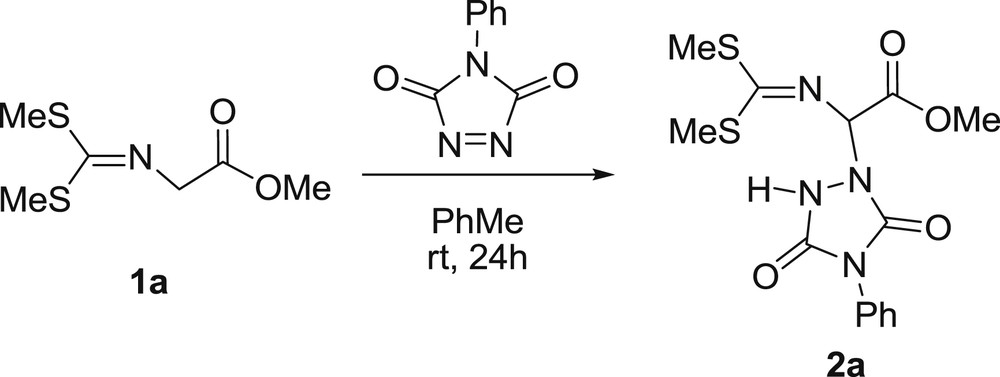
α-Amination of imine 1a by PhTAD.
α-Aminations of a variety of compounds with azodicarboxylates are known in the literature, mainly in their catalytic and asymmetric versions, due to the importance of the stereoselective synthesis of aminated compounds. Carbonyl compounds are a major class of substrates for α-amination [25], and so asymmetric aminations of aldehydes [26], ketones [27], keto- [28] and cyano-esters [29] have been reported. Recently, a nice work of a AgOAc catalyzed asymmetric amination of glycine Schiff bases with azodicarboxylates was reported to afford enantiomericaly enriched α-aminated products [30]. The stereoselectivity was induced by an asymmetric ligand present in the reaction. AgOAc/Taniaphos complex was acting as the catalyst and conjugated to the imine. Acetate anion was liberated and acted as a base for Hα abstraction. The resulted metalated azomethine ylide dipole then underwent electrophilic addition to azodicarboxylate. To our knowledge, this is the only example of α-amination of amino acid Schiff bases and for this reason, we continued the work with the initial finding of the synthesis of α-aminated glycine Schiff base 2a.
At first, we tried to find the optimal reaction conditions. The yield of 2a remained low (∼6%) even at elevated reaction temperatures (up to 180 °C) with toluene as the solvent. We examined PhCH3, THF, CH2Cl2, and CHCl3 as solvents under neutral, acidic (10% CH3COOH) and basic (10% NEt3) conditions at different temperatures. Finally it was found that the product yields were improved substantially when the reaction was performed in CH2Cl2, at 100 °C, or in CHCl3 at 120 °C (reaction in a screw capped tube) under neutral conditions for 24 h, with comparable yields. In general, the reaction was performed by placing the desired quantity of the imines of glycinates, 1, dissolved in dry CHCl3 in a screw-capped test-tube flushed with Ar. To this solution, solid PhTAD (2 mol/equivalent with respect to the imine) was added in one portion. Then the solution was stirred at 120 °C and the reaction was stopped after 24 h (Scheme 2). After that period of time, in most of the cases, the initially red (from PhTAD) solution was decolorized or color-faded, and this was taken as evidence of total PhTAD consumption. Then the solvent was removed in the rotary evaporator and aminated products 2 were isolated by column chromatography on SiO2 with EtOAc/hexanes mixtures as the eluant. Products and yields are listed in Table 1. Furthermore, cyclization products 3 were observed with low yields (8%-trace). The highest yield (8%) was observed with 3a. Compound 3b was observed with 4% yield. However, the other cyclization products were obtained with 2%-trace yields.
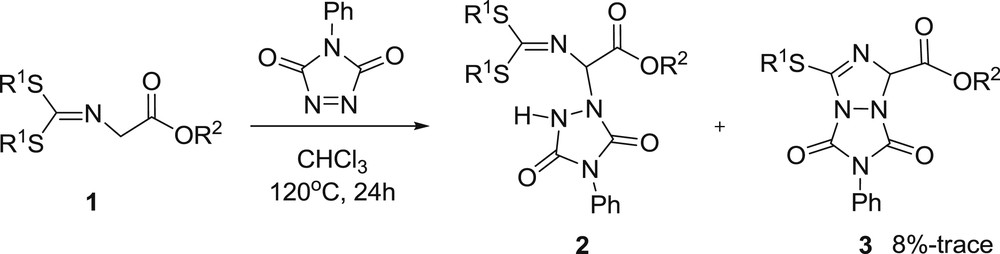
General reaction scheme for the synthesis of the α-aminated glycine ester Schiff bases 2.
Isolated yields of products 2.a
Entry | Adduct | R1 | R2 | Yield %b |
1 | 2a | Me | Me | 61 |
2 | 2b | Et | Me | 55 |
3 | 2c | Pr | Me | 56 |
4 | 2d | iPr | Me | 40 |
5 | 2e | Bu | Me | 49 |
6 | 2f | iBu | Me | 42 |
7 | 2g | secBu | Me | 46 |
8 | 2h | Bn | Me | 15 |
9 | 2i | Me | Et | 35 |
10 | 2j | Me | tBu | 30 |
11 | 2k | Me | Bn | 58 |
a All the reactions were performed with 0.290 mmol of imine 1, 0.725 mmol of PhTAD, in 5 mL of dry CHCl3, at 120 °C for 24 h.
b Isolated yields of products 2 after chromatographic purification.
Products 2 were characterized by 1H and 13C NMR, and FTIR spectroscopies and by HRMS spectrometry. Characteristic resonances to be mentioned from their 1H NMR spectra are: a) the NH signal at ∼8.30 ppm, as a single broad peak, b) the Hα hydrogen as a single peak at ∼6.45 ppm, c) the non-equivalence of the two sulfide substituents, and d) the diastereotopicity of methylene hydrogens in the EtO- and BnO- groups (entries 9 and 11). The above mentioned non-equivalence of the sulfide substituents was evident also from the 13C NMR spectra and the respective carbon atoms appeared as twin peaks for the syn and anti substituent. The methinic Cα appears at ∼73 ppm, and the iminic carbon resonates at ∼170 ppm.
In the case of 2a and 3a, crystals of adequate quality for X-ray diffraction analysis [31] were obtained from slow evaporation of CHCl3/hexanes, 1:1 v/v, Figs. 1 and 2. All the spectroscopic data obtained agree with the structures.
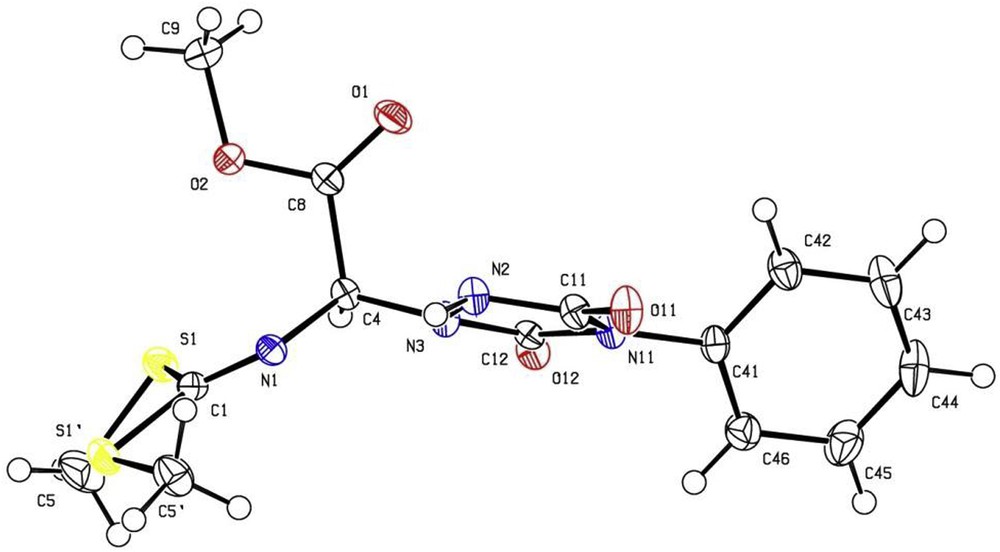
X-ray structure of compound 2a.
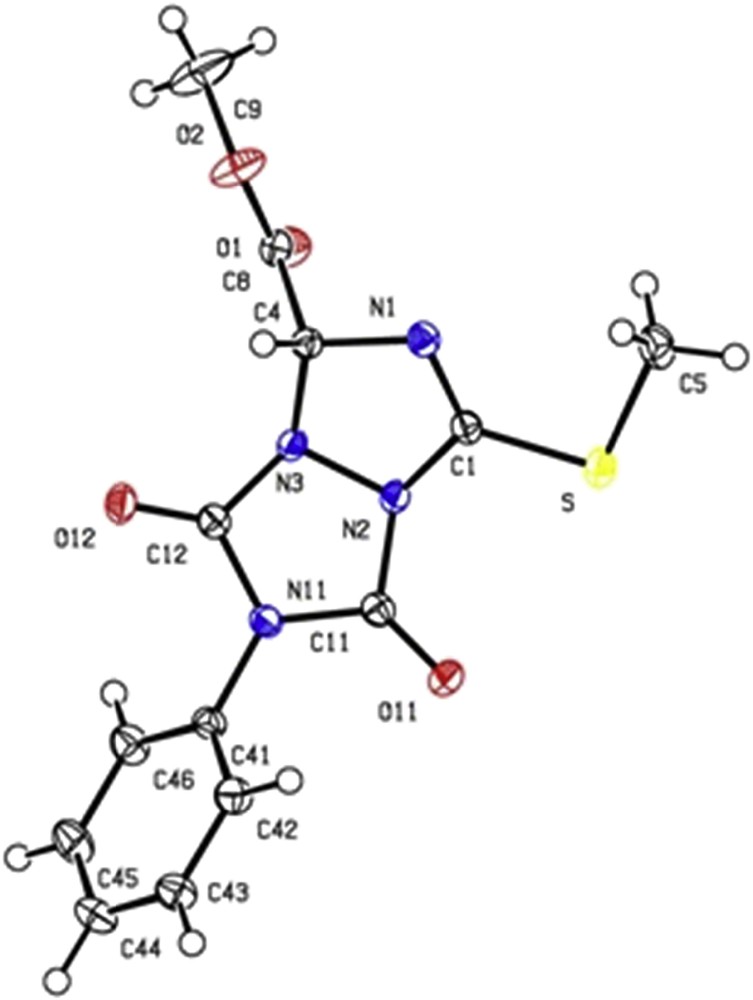
X-ray structure of compound 3a.
To gain more information about the transformation, we performed intermolecular competition experiments using equimolar mixtures of two imines 1, keeping the amount of PhTAD at the half of the stoichiometric molar ratio. Thus starting from a 1:1:0.5 M ratio of imine 1a:imine 1B:PhTAD (Scheme 3), and applying the same conditions mentioned above, we have calculated the molar ratio of the two resulted adducts 2a and 2B, from the 1H NMR spectra of the crude reaction mixture, by integration of appropriate peaks. The molar ratio of the products reflects to the ratio of their formation rates. With the above competitions, we wished to reveal any steric, influence that the sulfide substituent has on the rate of α-amination. For this reason, one imine was kept constant, 1a, and gradually changed the size of the –SR alkyl chain of the competitor imine, 1B. Competing couples used in this study and calculated molar ratios of the α-aminated products are shown in Table 2.
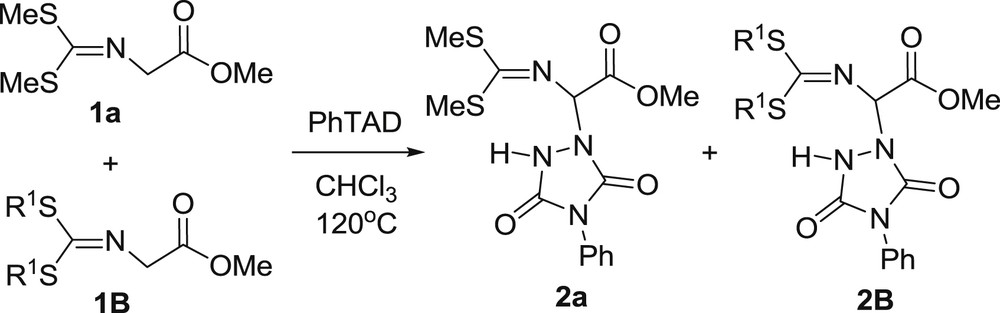
Intermolecular competition between imines 1 for α-amination by PhTAD.
Calculated molar ratios of the two products [2a]/[2B], from 1a vs 1B intermolecular competition for their amination by PhTAD.a,b
Entry | R1 | [2a]/[2B] |
1 | Et | 0.18 |
2 | Pr | 0.12 |
3 | i-Pr | 0.23 |
4 | Bu | 0.62 |
5 | i-Bu | 0.69 |
6 | sec-Bu | 0.14 |
a All competitions were performed with 1a:1B:PhTAD 1:1:0.5 M ratio.
b Molar ratios were calculated from integrals of appropriate peaks from the 1H NMR spectra of the crude reaction mixture.
The results from Table 2 clearly show that there is a correlation between the size of the substituent and the rate of the amination. The larger the size of the substituent, the faster the reaction. If a steric factor was in operation, then a bigger than unity molar ratio would be expected due to the steric hindrance during the approach of the two reactants (in benefit of the 1a substrate).
To come up with a reliable explanation of the above observation, we turned our attention to the very first period of the 1,3-dipoles' evolution for 1,3-dipolar cycloadditions, with reports from the group of Grigg. 1,3-Azomethine dipole is an effective component for the 1,3-cycloaddition between an imine and an electrophile. A precursor 1,2-dipole needs to precede via a [1,2-H] prototropic shift of a Hα in the glycine imine, 1. The more efficient formation of the two dipoles reflects to the more efficient 1,3-dipolar cycloaddition reaction. In our opinion, the differences in molar (and rate) ratios owe their existence to the different degrees of the dipoles' stabilization. Thus the larger the alkyl chain of the sulfide substituents inductively stabilizes, more the positive charge in the dipoles, Fig. 3, together with the positive resonance effect of the sulfur atoms.

Plausible mechanistic interpretation for the α-amination sequence through the quench of the 1,2-dipole by electrophiles such as PhTAD and D+.
The intervention of the 1,2-dipole was further revealed by deuterium exchange of the methylenic Hα protons in 1, heating a solution of imine 1a (20 mg) in MeOD (0.5 mL) at 50 °C. The 1H NMR spectra of an aliquot taken after 18 h showed ∼45% di-deuterated imine 1a–d2, together with 55% mixture of 1a–d0 and 1a–d1 imines. The degree of di-deuteration increased after a second circle [removal of the MeOH(D) and dissolution of the partially deuterated imine 1a in 0.5 mL of MeOD and stirring with heating at 50 °C for 16 h] to >80% in 1a–d2 and <20% in 1a–d1. The deuterium exchange was almost complete (>95% in 1a–d2, and <5% in 1a–d1) after a third circle, in line with earlier observations in the exchange of a more acidic (methinic) Hα proton, of an imine of phenylglycine methyl ester [5].
Next, we made reactions between sulfur-containing Schiff bases of several different amino acid esters such as alanine, phenylalanine, leucine, tyrosine, aspartic acid, glutamic acid and N-PhTAD under the same conditions (Scheme 4, Table 3). In all cases, the main products observed were triazoline derivatives via the 5-exo-trig cyclization reaction, and α-amination products formed in very low yields (2%-trace). We have used TEMPO, DIAD, and DBAD as catalysts to get α-amination products encouraged in the literature [32] but we again observed triazoline derivatives as main products and the yields didn't change. Finally we made reactions with benzylamine derivatives's Schiff bases and N-PhTAD. In most cases, the triazoline derivatives were the only product observed. However, 6a gave a mixture of triazoline and α-amination products with the yields 72% and 19%, respectively (Scheme 5, Table 4). Substrates 4 and 6 gave the thermal 5-exo-trig cyclization reaction which occurs due to the side-chain steric effects and this cyclization might happen via α-amination products (Scheme 6).
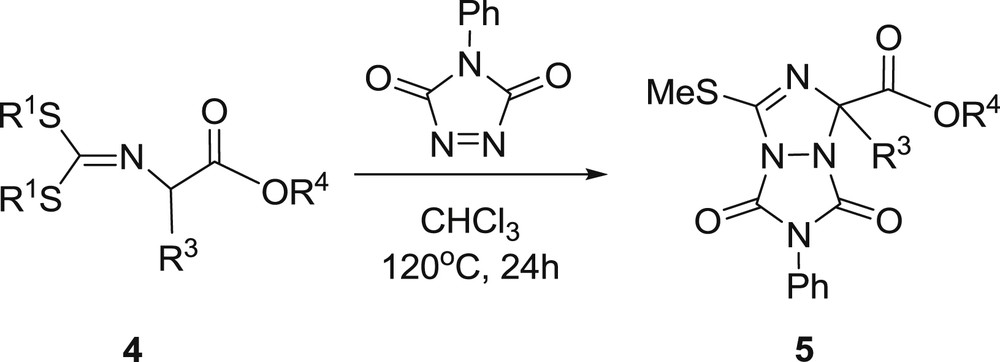
General reaction scheme for the synthesis of the triazolines 5.
Isolated yields of products 5.a
Entry | Adduct | R3 | R4 | Yield %b |
1 | 5a | Methyl | Methyl | 72 |
2 | 5b | 2-Methoxy-2-oxoethyl | Methyl | 68 |
3 | 5c | 3-Methoxy-3-oxopropyl | Methyl | 64 |
4 | 5d | 1-Isobuthyl | Methyl | 69 |
5 | 5e | 2-Methylthioethyl | Methyl | 32 |
6 | 5f | Benzyl | Methyl | 61 |
7 | 5g | 4-Methoxybenzyl | Methyl | 60 |
8 | 5h | Ethoxycarbonyl | Ethyl | 61 |
a All the reactions were performed with 0.290 mmol of imine 4, 0.725 mmol of PhTAD, in 5 mL of dry CHCl3, at 120 °C for 24 h.
b Isolated yields of products 5 after chromatographic purification.

General reaction scheme for the synthesis of the triazolines 7a–d and α-aminated Schiff base 8a.
Isolated yields of products 7a–d and 8a.a
Entry | Adduct | R5 | R6 | Yield %b |
1 | 7a | H | Ph | 57 |
2 | 7b | H | 4-F Ph | 49 |
3 | 7c | Me | Ph | 53 |
4 | 7d | Ph | Ph | 53 |
5 | 8a | H | Ph | 19 |
a All the reactions were performed with 0.290 mmol of imine 6, 0.725 mmol of PhTAD, in 5 mL of dry CHCl3, at 120 °C for 24 h.
b Isolated yields of products 7a–d and 8a after chromatographic purification.

Plausible mechanism of 5-exo-trig reaction.
Products 5 and 7 were characterized by 1H and 13C NMR, and FTIR spectroscopies and by HRMS spectrometry. Characteristic resonances to be mentioned from their 1H NMR spectra are: a) the OCH3 as a single peak at ∼3.85–3.60 ppm (for 5), b) the sulfide substituent as a single peak at ∼2.50–2.65 ppm (for 5 and 7).
3 Biological studies
We tested the new cycloadducts with regard to their anti-lipid peroxidation ability and as soybean lipoxygenase inhibitors (LOX) in comparison to well known reference compounds, for example, nordihydroguaiaretic acid (NDGA), and trolox (Fig. 4).
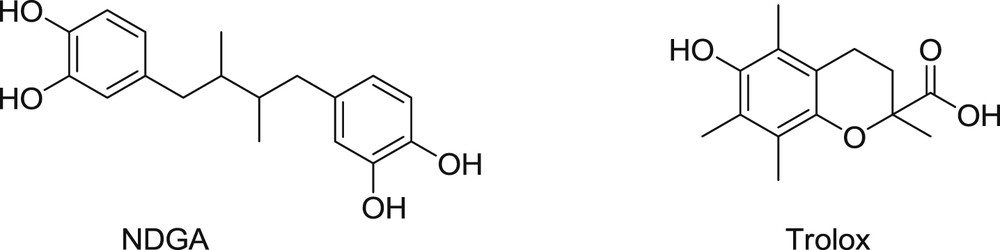
The structure of the antioxidant compounds nordihydroguaiaretic acid (NDGA) and trolox.
The use of the free radical reaction initiator 2,2-azobis(2-amidinopropane) dihydrochloride (AAPH) is recommended as more appropriate for measuring radical-scavenging activity in vitro, and anti-lipid peroxidation ability. This is supported by the fact that the activity of the peroxyl radicals produced by the action of AAPH shows a greater similarity to cellular activities such as lipid peroxidation [33]. Oxidation of sodium linoleate by a thermal free radical initiator (AAPH) is followed by UV spectrophotometry in a highly diluted sample [34]. In the AAPH assay, the highly reactive alkylperoxyl radicals are intercepted mainly by a hydrogen atom transfer (HAT) from the antioxidant [35]. Therefore, particularly effective HAT agents are compounds with high hydrogen atom donating ability, that is, compounds with low heteroatom-H bond dissociation energies and/or compounds from which hydrogen abstraction leads to sterically hindered radicals as well as compounds from which abstraction of hydrogen leads to C-centered radicals stabilized by resonance. Compounds 2c, 2h, 7b, 7c and 7d caused strong inhibition of lipid peroxidation (70–93%) and higher than trolox (65%). Compound 7d with the highest lipophilicity (clog P = 4.769) is the most potent. The presence of at least one phenyl group (R6) is correlated with higher antioxidant activity. Since these compounds lack the easily oxidizable phenol unit present in trolox, their inhibitory potency should be attributed to other factors. Perusal of Table 5 points to lower activity (29–54%) for the rest adducts whereas one (5g) was almost inactive (5%). This could be attributed to stereochemical reasons, due to the presence of bulky substituents in R3 R4 R5 R6, especially for the products of series 2 and 5.
Biological evaluation of novel compounds as antioxidants.
Entry | Adduct | %LOX Inh. @100 μMa/IC50 μΜ | AAPH% @100 μMb | Clog Pc |
1 | 2a | No | 37 | 2.15 |
2 | 2b | No | 37 | 3.21 |
3 | 2c | 85.5 μΜ (59%) | 70 | 4.27 |
4 | 2d | No | 31 | 3.83 |
5 | 2e | 13% | 29 | 5.33 |
6 | 2f | No | 21 | 5.07 |
7 | 2g | 4% | 33 | 4.89 |
8 | 2h | 60 μΜ (92%) | 93 | 4.98 |
9 | 2i | 8% | 46 | 2.68 |
10 | 2j | 16% | 44 | 3.39 |
11 | 2k | 20% | 47 | 3.38 |
12 | 5a | 7% | 52 | 2.60 |
13 | 5b | 24% | 46 | 2.37 |
14 | 5c | No | 41 | 2.089 |
15 | 5d | No | 43 | 4.11 |
16 | 5f | 33% | 48 | 4.02 |
17 | 5g | 10% | 5 | 3.94 |
18 | 5h | 26% | 54 | 2.57 |
19 | 7a | 88 μΜ | 49 | 3.21 |
20 | 7b | 57.5 μΜ (94%) | 90 | 3.35 |
21 | 7c | 17% | 79 | 3.87 |
22 | 7d | 65 μΜ (81%) | 92 | 4.767 |
23 | NDGA | 45 μΜ (83%) | ||
Trolox | 65 |
a In vitro % inhibition of soybean lipoxygenase (LOX).
b % Inhibition of Lipid Peroxidation (AAPH).
c Ref. 36.
Antioxidants are defined as substances that, even at low concentration, significantly delay or prevent oxidation of easily oxidizable substrates. The formation of Reactive Oxygen Species (ROS) is a characteristic of aerobic organisms, as an unavoidable consequence of cell metabolism lipoxygenase (LOX) catalyzes the first two steps in the metabolism of arachidonic acid, (which is cleaved from membrane phospholipids) to leukotrienes (LTB4). LTB4 generation is considered to be important in the pathogenesis of neutrophil-mediated inflammatory diseases [35] with a marked relation to the severity of cardiovascular diseases, asthma and cancer. In this context, we decided to further evaluate the synthesized cycloadducts as soybean LOX inhibitors by the UV absorbance based enzyme assay [36]. NDGA, a known inhibitor of soybean LOX, has been used as a reference compound (83% or 45 μΜ) and as a positive control. The compounds exhibited low or no inhibition under the reported conditions. Most potent was found to be compound 7b followed by 2h, 7d and 2c. Since the differences among 7b and 2h (as well as 7d) adducts in terms of IC50 are very small, their structural characteristics are quite different. Comparing 2c and 2h, it is obvious that higher lipophilicity is correlated with higher inhibition (clog P 4.98/IC50 = 60 μΜ, clog P 4.27/IC50 = 85.5 μΜ). It should be mentioned that most of the LOX inhibitors are antioxidants or free radical scavengers. Since these compounds present the highest antioxidant abilities, their lipoxygenase inhibition is proceeded in parallel through this mechanism.
4 Conclusion
In summary we presented here a simple procedure for the α-amination of glycine Schiff bases with PhTAD as the aminating component, under neutral conditions without the use of a catalyst, and 5-exo-trig cyclization reactions of several sulfur-containing imines with PhTAD under the same conditions. These products present interesting biological activities as inhibitors of lipid peroxidation and of lipoxygenase. Especially the 7b, 7d and 2h derivatives highly inhibit lipid peroxidation and soybean LOX and they might serve as lead compounds.
5 Experimental
5.1 General
All NMR spectra were taken in CDCl3 98% D, on a Bruker-Spectrospin, Avance spectrometer. ESI MS spectra were taken on an Agilent Technologies 1100 Series LC/MSD-Trap-SL spectrometer. HRMS were taken on an Orbitrap LTQ/XL instrument. FTIR spectra were taken on a Perkin Elmer Spectrum GX, FTIR System spectrometer. All reagents and solvents were obtained from commercial suppliers and used without further purification. Dry quality solvent was obtained according to literature procedures and stored under an Ar atmosphere. Compound 1 was prepared by known literature methods [37].
5.2 Typical procedure for preparation of the α-aminated Schiff bases and triazoline derivatives
A screw-capped 10-mL tube flashed with Ar. In it were placed 0.290 mmol sulfur substituted amino acid esters 1 or 4, or sulfur substituted imines 6, and 0.725 mmol N-Phenyltriazolinedione (N-PhTAD) in 5 mL of dry CHCl3. This solution was stirred at 120 °C for 24 h. The tube was left to reach room temperature. Volatiles were removed in a rotary evaporator and the remaining material was then chromatographed on a silica gel column with a mixture of EtOAc/n-hexane, 1/3, v/v, as eluant in order to separate pure products.
5.2.1 Methyl-2-(bis(methylthio)methyleneamino)-2-(3,5-dioxo-4-phenyl-1,2,4-triazolidin-1-yl)acetate (2a) C14H16N4O4S2
Colorless powder, yield 61%, mp: 134–136 0C. FTIR (KBr), vmax: 3477, 3151, 3070, 3004, 2950, 2922, 1779, 1749, 1697, 1564, 1503, 148, 1285, 1252, 1138, 1075, 1048, 1024, 1005, 818, 767, 727, and 713 cm−1. 1H NMR (250 MHz, CDCl3) δ: 7.86 (br s, 1H), 7.50–7.44 (m, 5H), 6.42 (s, 1H), 3.81 (s, 3H), 2.62 (s, 3H), and 2.38 (s, 3H) ppm. 13C NMR (62.5 MHz, CDCl3) δ: 171.03, 166.59, 153.71, 1 53.19, 130.94, 129.13, 128.37, 125.64, 73.03, 53.40, 15.81, and 15.04 ppm. HRMS (ESI-Orbit trap) m/z: [M+H]+ calcd for C14H16N4O4S2+H, 369.1265; found 369.1265.
5.2.2 Methyl-2-(bis(ethylthio)methyleneamino)-2-(3,5-dioxo-4-phenyl-1,2,4-triazolidin-1-yl)acetate (2b) C16H20N4O4S2
Colorless powder, yield 55%, mp: 122–124 0C. FTIR (KBr), vmax: 3464, 3151, 3055, 2972, 2935, 2870, 1781, 1734, 1709, 1600, 1562, 1504, 1431, 1340, 1277, 1233, 1138, 1077, 1026, 821, 768, 725, 709 cm−1. 1H NMR (250 MHz, CDCl3) δ: 8.32 (br s, 1H), 7.47–7.49 (m, 5H), 6.46 (s, 1H), 3.80 (s, 3H), 3.16 (qd, 2H, J1 = 7.25 Hz, J2 = 5.50 Hz), 2.98 (qd, 2H, J1 = 7.25 Hz, J2 = 6.00 Hz), 1.37 (t, 3H, J = 7.38 Hz), 1.19 (t, 3H, J = 7.38 Hz) ppm. 13C NMR (62.5 MHz, CDCl3) δ: 169.70, 166.60, 153.83, 153.32, 131.03, 129.13, 128.37, 125.68, 73.38, 53.37, 27.89, 26.28, 14.99, 13.61 ppm. HRMS (ESI-Orbit trap) m/z: [M+H]+ calcd for C16H20N4O4S2+H, 397.0999; found 397.0996.
5.2.3 Methyl-2-(bis(propylthio)methyleneamino)-2-(3,5-dioxo-4-phenyl-1,2,4-triazolidin-1-yl)acetate (2c) C18H24N4O4S2
Colorless powder, yield 56%, mp: 111–113 0C. FTIR (KBr), vmax: 3483, 3114, 2963, 2863, 1744, 1690, 1559, 1502, 1465, 1398, 1336, 1221, 1137, 1072, 1023, 1003, 815, 779, 707 cm−1. 1H NMR (250 MHz, CDCl3) δ:7.87 (br s, 1H), 7.49–7.47 (m, 5H), 6.46 (s, 1H), 3.78 (s, 3H), 3.12 (td, 2H, J1 = 7.25 Hz, J2 = 5.75 Hz), 2.93 (td, 2H, J1 = 7.25 Hz, J2 = 6.25 Hz) 1.73 (sextet, 2H, J = 7.25 Hz), 1.53 (sextet, 2H, J = 7.25 Hz), 1.01 (t, 3H, J = 7.25), 0.88 (t, 3H, J = 7.38). 13C NMR (62.5 MHz, CDCl3) δ: 169.91, 166.54, 153.89, 153.35, 131.02, 129.06, 128.30, 125.65, 73.40, 53.30, 50.73, 35.38, 33.67, 23.27, 21.87, 13.23 ppm. HRMS (ESI-Orbit trap) m/z: [M+H]+ calcd for C18H24N4O4S2+H, 425.1312; found 425.1313.
5.2.4 Methyl-2-(bis(isopropylthio)methyleneamino)-2-(3,5-dioxo-4-phenyl-1,2,4-triazolidin-1-yl)acetate (2d) C18H24N4O4S2
Colorless powder, yield = 40%, mp: 108–110 °C. FTIR (KBr), vmax: 3467, 3165, 3078, 2969, 2927, 2866, 1781, 1738, 1713, 1600, 1556, 1504, 1434, 1385, 1367, 1236, 1139, 1078, 1056, 1025, 818, 773, 713 cm−1. 1H NMR (250 MHz, CDCl3) δ: 8.11 (br s, 1H), 7.48–7.38 (m, 5H), 6.53 (s, 1H), 3.85 (septet, 2H, J = 6.75 Hz), 3.80 (s, 3H), 1.38 (d, 6H, J = 7.00 Hz), 1.26 (d, 6H, J = 7.00 Hz). 13C NMR (62.5 MHz, CDCl3) δ: 169.43, 166.66, 153.83, 153.50, 131.01, 129.19, 128.40,125.70, 73.83, 53.37, 39.62, 37.05, 24.58, 23.12, 22.32, 21.74 ppm. HRMS (ESI-Orbit trap) m/z: [M+H]+ calcd for C18H24N4O4S2+H, 425.1312; found 425.1318.
5.2.5 Methyl-2-(bis(butylthio)methyleneamino)-2-(3,5-dioxo-4-phenyl-1,2,4-triazolidin-1-yl)acetate (2e) C20H28N4O4S2
Colorless powder, yield = 49%, mp: 100–102 °C. FTIR (KBr), vmax: 3468, 3151, 3076, 2957, 2928, 2871, 1781, 1760, 1708, 1569, 1495, 1427, 1276, 1234, 1181, 1138, 1080, 1069, 1027, 821, 778, 740, 716 cm−1. 1H NMR (250 MHz, CDCl3) δ: 8.30 (br s, 1H), 7.49–7.44 (m, 5H), 6.46 (s, 1H), 3.79 (s, 3H), 3.13 (td, 2H, J1 = 7.25 Hz, J2 = 5.25 Hz), 2.97 (td, 2H, J1 = 7.25 Hz, J2 = 5.25 Hz), 1.67 (quintet, 2H, J = 7.25 Hz), 1.49 (sextet, 2H, J = 7.25), 1.34 (sextet, 2H, J = 7.25), 0.93 (t, 3H, J = 7.25 Hz), 0.85 (t, 3H, J = 7.25 Hz). 13C NMR (62.5 MHz, CDCl3) δ: 170.07, 166.62, 153.79, 153.31, 131.05, 129.09, 128.30, 125.98, 125.61, 73.31, 53.31, 33.18, 31.75, 31.55, 30.47, 21.83, 21.78, 13.63, 13.48 ppm. HRMS (ESI-Orbit trap) m/z: [M+H]+ calcd for C20H28N4O4S2+H, 453.1625; found 453.1626.
5.2.6 Methyl-2-(bis(isobutylthio)methyleneamino)-2-(3,5-dioxo-4-phenyl-1,2,4-triazolidin-1-yl)acetate (2f) C20H28N4O4S2
Colorless powder, yield = 42%, mp: 95–97 °C. FTIR (KBr), vmax: 3472, 3153, 3065, 2967, 2926, 2870, 1784, 1746, 1713, 1602, 1569, 1504, 1434, 1385, 1368, 1331, 1250, 1192, 1138, 1082, 1026, 822, 778, 717 cm−1. 1H MNR (250 MHz, CDCl3) δ: 7.73 (br s, 1H), 7.49–7.47 (m, 5H), 6.51 (s, 1H), 3.81 (s, 3H), 3.10 (dd, 2H, J1 = 7.75 Hz, J2 = 5.50 Hz), 2.81 (dd, 2H, J1 = 7.00 Hz, J2 = 6.25 Hz), 1.90 (dt, 2H, J1 = 6.75 Hz, J2 = 6.50 Hz), 1.03 (dd, 6H, J1 = 4.75 Hz, J2 = 1.75 Hz), 0.96 (dd, 6H, J1 = 6.00 Hz, J2 = 0.75 Hz) ppm. 13C NMR (62.5 MHz, CDCl3) δ: 170.39, 166.77, 153.61, 153.41, 131.07, 129.17, 128.37, 125.57, 73.31, 53.36, 42.01, 40.12, 29.24, 28.18, 22.00, 21.86, 21.68 ppm. HRMS (ESI-Orbit trap) m/z: [M+H]+ calcd for C20H28N4O4S2+H, 453.1625; found 453.1627.
5.2.7 Methyl-2-(bis(sec-butylthio)methyleneamino)-2-(3,5-dioxo-4-phenyl-1,2,4-triazolidin-1-yl)acetate (2g) C20H28N4O4S2
Colorless powder, yield = 46%, mp: 97–99 °C. FTIR (KBr), vmax: 3441, 3100, 2965, 2915, 2878, 1782, 1749, 1713, 1598, 1560, 1503, 1428, 1380, 1332, 1222, 1170, 1133, 1056, 1026, 820, 772, 690 cm−1. 1H NMR (250 MHz, CDCl3) δ: 7.75 (br s, 1H), 7.49–7.38 (m, 5H), 6.56 (4s, 1H, J = 2.42 Hz), 3.81 (s, 3H), 3.75–3.65 (m, 2H), 1.74–1.50 (m, 4H), 1.38 (dd, 3H, J1 = 4.00 Hz, J2 = 2.75 Hz), 1.29 (dd, 3H, J1 = 6.00 Hz, J2 = 1.00 Hz), 1.06–0.88 (m, 6H) ppm. 13C NMR (62.5 MHz, CDCl3) δ: 170.00, 166.82, 153.69, 153.67, 131.03, 129.20, 128.40, 125.65, 125.58, 73.75, 53.38, 46.35, 45.87, 43.32, 43.19, 29.77, 29.10, 20.59, 11.60, 11.41 ppm. HRMS (ESI-Orbit trap) m/z: [M+H]+ calcd for C20H28N4O4S2+H, 453.1625; found 453.1629.
5.2.8 Methyl-2-(bis(benzylthio)methyleneamino)-2-(3,5-dioxo-4-phenyl-1,2,4-triazolidin-1-yl)acetate (2h) C26H24N4O4S2
Colorless powder, yield = 15%, mp: 159–161 °C. FTIR (KBr), vmax: 3468, 3166, 3062, 3033, 2953, 2922, 2848, 1778, 1745, 1709, 1599, 1561, 1495, 1450, 1235, 1174, 1136, 1071, 1026, 813, 770, 699 cm−1. 1H NMR (250 MHz, CDCl3) δ: 8.21 (br s, 1H), 7.48–7.30 (m, 15H), 6.43 (s, 1H), 4.32 (d, 2H, J = 14.50 Hz), 4.22 (d, 2H, J = 13.75 Hz), 3.75 (s, 3H) ppm. 13C NMR (62.5 MHz, CDCl3) δ: 168.50, 166.41, 153.81, 153.12, 136.44, 135.18, 130.92, 129.04, 128.94, 128.74, 128.53, 128.28, 127.83, 127.38, 125.58, 73.13, 53.37, 50.81, 38.03, 36.32 ppm. HRMS (ESI-Orbit trap) m/z: [M+H]+ calcd for C26H24N4O4S2+H, 521.1312; found 521.1316.
5.2.9 Ethyl-2-(bis(methylthio)methyleneamino)-2-(3,5-dioxo-4-phenyl-1,2,4-triazolidin-1-yl)acetate (2i) C15H18N4O4S2
Colorless powder, yield = 35%, mp: 118–120 °C. FTIR (KBr), vmax: 3460, 3165, 3070, 2987, 2930, 1778, 1749, 1694, 1574, 1504, 1434, 1277, 1136, 1078, 1068, 1016, 832, 773, 711 cm−1. 1H NMR (250 MHz, CDCl3) δ: 8.60 (br s, 1H), 7.49–7.37 (m, 5H), 6.37 (s, 1H), 4.33 (qd, 1H, J1 = 7.00 Hz, J2 = 3.50 Hz), 4.15 (qd, 1H, J1 = 7.25 Hz, J2 = 3.50 Hz), 2.60 (s, 3H), 2.34 (s, 3H), 1.28 (t, 3H, J = 7.25 Hz) ppm. 13C NMR (62.5 MHz, CDCl3) δ: 170.68, 165.88, 153.86, 153.21, 131.05, 129.07, 128.29, 125.60, 73.30, 62.74, 15.83, 14.99, 14.06 ppm. HRMS (ESI-Orbit trap) m/z: [M+H]+ calcd for C15H18N4O4S2+H, 383.0842; found 383.0839.
5.2.10 tert-Butyl-2-(bis(methylthio)methyleneamino)-2-(3,5-dioxo-4-phenyl-1,2,4-triazolidin-1-yl)acetate (2j) C17H22N4O4S2
Colorless powder, yield = 30%, mp: 160–162 °C. FTIR (KBr), vmax: 3474, 3195, 3062, 2976, 2924, 1778, 1745, 1699, 1574, 1493, 1435, 1369, 1262, 1225, 1151, 1084, 1024, 1004, 844, 775, 721, 695 cm−1. 1H NMR (250 MHz, CDCl3) δ: 8.45 (br s, 1H), 7.53–7.39 (m, 5H), 6.28 (s, 1H), 2.59 (s, 3H), 2.35 (s, 3H), 1.45 (s, 9H) ppm. 13C NMR (62.5 MHz, CDCl3) δ: 169.87, 164.56, 153.78, 153.07, 131.12, 129.05, 128.17, 125.44, 84.20, 73.83, 27.91, 15.85, 14.98 ppm. HRMS (ESI-Orbit trap) m/z: [M+H]+ calcd for C17H22N4O4S2+H, 411.1155; found 411.1157.
5.2.11 Benzyl-2-(bis(methylthio)methyleneamino)-2-(3,5-dioxo-4-phenyl-1,2,4-triazolidin-1-yl)acetate (2k) C20H20N4O4S2
Colorless powder, yield = 58%, mp: 154–156 °C. FTIR (KBr), vmax: 3468, 3151, 3066, 3025, 2944, 2922, 2885, 1785, 1730, 1705, 1570, 1501, 1455, 1424, 1336, 1248, 1181, 1134, 1075, 1064, 1023, 1006, 852, 770, 750, 708 cm−1. 1H NMR: (250 MHz, CDCl3) δ: 8.16 (br s, 1H), 7.41–7.31 (m, 10H), 6.44 (s, 1H), 5.29 (d, 1H, J = 12.25 Hz), 5.15 (d, 1H, J = 12.25 Hz), 2.60 (s, 3H), 2.35 (s, 3H) ppm. 13C NMR: (62.5 MHz, CDCl3) δ: 171.03, 165.84, 153.71, 153.21, 134.58, 130.86, 129.03, 128.67, 128.19, 125.67, 73.23, 68.25, 15.82, 15.04 ppm. HRMS (ESI-Orbit trap) m/z: [M+H]+ calcd for C20H20N4O4S2+H, 445.0999; found 445.0999.
5.2.12 Methyl-3-(methylthio)-5,7-dioxo-6-phenyl-1,5,6,7-tetrahydro[1,2,4]triazolo[1,2-a][1,2,4]triazole-1-carboxylate (3a) C13H12N4O4S
Colorless powder, yield = 8%, mp: 142–144 °C. FTIR (KBr), vmax: 3084, 3025, 2952, 2925, 2848, 1798, 1755, 1594, 1561, 1500, 1428, 1404, 1362, 1233, 1213, 1170, 1141, 1115, 1070, 1019, 834, 782, 759 cm−1. 1H NMR (250 MHz, CDCl3) δ: 7.49–7.45 (m, 5H), 6.13 (s, 1H), 3.89 (s, 3H), 2.60 (s, 3H) ppm. 13C NMR (62.5 MHz, CDCl3) δ: 165.93, 158.51, 154.41, 149.91, 130.29, 129.39, 129.07, 125.70, 82.63, 53.57, 14.36 ppm. HRMS (ESI-Orbit trap) m/z: [M+H]+ calcd for C13H12N4O4S+H, 321.0652; found 321.0647.
5.2.13 Methyl-3-(ethylthio)-5,7-dioxo-6-phenyl-1,5,6,7-tetrahydro[1,2,4]triazolo[1,2-a][1,2,4]triazole-1-carboxylate (3b) C14H14N4O4S
Colorless powder, yield = 4%, mp: 148–150 °C. FTIR (KBr), vmax: 3062, 2952, 2926, 2848, 1797, 1740, 1575, 1500, 1454, 1432, 1258, 1174, 1141, 1104, 1067, 1019, 835, 762, 690 cm−1. 1H NMR (250 MHz, CDCl3) δ: 7.47–7.45 (m, 5H), 6.12 (s, 1H), 3.89 (s, 3H), 3.19 (q, 2H, J = 7.37 Hz), 1.43 (t, 3H, J = 7.37 Hz) ppm. 13C NMR (62.5 MHz, CDCl3) δ: 166.00, 158.50, 153.00, 148.00, 129.47, 129.44, 129.37, 129.12, 129.04, 125.70, 82.56, 53.55, 30.93, 13.79 ppm. HRMS (ESI-Orbit trap) m/z: [M+H]+ calcd for C14H14N4O4S+H, 335.0809; found 335.0806.
5.2.14 Methyl-1-methyl-3-(methylthio)-5,7-dioxo-6-phenyl-1,5,6,7-tetrahydro[1,2,4]triazolo[1,2-a][1,2,4]triazole-1-carboxylate (5a) C14H14N4O4S
Colorless crystals, yield = 72%, mp: 148–150 °C. FTIR (KBr), vmax: 3110, 3066, 3004, 2944, 1728, 1568, 1404, 1272, 1144, 984, 910, 750 cm−1. 1H NMR (250 MHz, CDCl3) δ: 7.38–7.53 (m, 5H), 3.81 (s, 3H), 2.56 (s, 3H), 1.96 (s, 3H) ppm. 13C NMR (62.5 MHz, CDCl3) δ: 167.20, 155.80, 153.10, 148.20, 130.60, 129.30, 128.90, 125.80, 91.60, 53.60, 23.30, 14.10 ppm. HRMS (ESI-Orbit trap) m/z: [M+H]+ calcd for C14H14N4O4S+H, 335.0809; found 335.0808.
5.2.15 Methyl-1-(2-methoxy-2-oxoethyl)-3-(methylthio)-5,7-dioxo-6-phenyl-1,5,6,7-tetrahydro[1,2,4]triazolo[1,2-a][1,2,4]triazole-1-carboxylate (5b) C16H16N4O4S
White powder, yield = 68%, mp: 152–154 °C. FTIR (KBr), vmax: 3064, 2978, 2956, 2936, 1794, 1702, 1599, 1583, 1554, 1499, 1448, 1279, 1255, 1170, 1074, 1027, 883, 747 cm−1. 1H NMR (250 MHz, CDCl3) δ: 7.28–7.53 (m, 5H), 3.87 (s, 3H), 3.87 (d, 1H, J = 17.76 Hz), 3.66 (s, 3H), 3.37 (d, 1H, J = 17.76 Hz), 2.54 (s, 3H) ppm. 13C NMR (62.5 MHz, CDCl3) δ: 167.20, 155.80, 153.10, 148.20, 130.60, 129.30, 128.90, 125.80, 91.60, 53.60, 23.30, 14.10 ppm. HRMS (ESI-Orbit trap) m/z: [M+H]+ calcd for C16H16N4O6S+H, 393.0863; found 393.0858.
5.2.16 Methyl-1-(3-methoxy-3-oxopropyl)-3-(methylthio)-5,7-dioxo-6-phenyl-1,5,6,7-tetrahydro[1,2,4]triazolo[1,2-a][1,2,4]triazole-1-carboxylate (5c) C17H18N4O4S
Yellow powder, yield = 64%, mp: 155–157 °C. FTIR (KBr), vmax: 3128, 3070, 3018, 2956, 2856, 1800, 1734, 1574, 1406, 1266, 1130, 936, 758 cm−1. 1H NMR (250 MHz, CDCl3) δ: 7.37–7.52 (m, 5H), 3.81 (s, 3H), 3.69 (s, 3H), 2.50–2.70 (m, 4H), 2.55 (s, 3H) ppm. 13C NMR (62.5 MHz, CDCl3) δ: 172.80, 166.40, 156.60, 153.60, 148.40, 130.50, 129.30, 128.90, 125.90, 93.90, 53.70, 51.80, 31.00, 28.30, 14.10 ppm. HRMS (ESI-Orbit trap) m/z: [M+H]+ calcd for C17H18N4O6S+H, 407.1020; found 407.1012.
5.2.17 Methyl-1-isobuthyl-3-(methylthio)-5,7-dioxo-1,5,6,7-tetrahydro-[1,2,4]triazolo[1,2-a][1,2,4]triazole-1-carboxylate (5d) C17H20N4O4S
Yellow crystals, yield = 69%, mp: 158–160 °C. FTIR (KBr), vmax: 3032, 2958, 2870, 1786, 1726, 1562, 1496, 1398, 1230, 1018, 768 cm−1. 1H NMR (250 MHz, CDCl3) δ: 7.37–7.52 (m, 5H), 3.80 (s, 3H), 2.56 (s, 3H), 2.26 (dd, 2H, J1 = 6.37 Hz, J2 = 14.95 Hz), 1.93 (nonet, 1H, J = 6.37 Hz), 1.02 (d, J = 6.37 Hz), 1.01 (d, J = 6.37 Hz) ppm. 13C NMR (62.5 MHz, CDCl3) δ: 167.30, 155.10, 153.10, 148.20, 130.70, 129.30, 128.80, 125.90, 94.80, 53.60, 43.10, 23.90, 23.70, 14.10 ppm. HRMS (ESI-Orbit trap) m/z: [M+H]+ calcd for C17H20N4O4S+H, 377.1278; found 377.1263.
5.2.18 Methyl-(3-methylthio)-1-(2-methylthio)ethyl)-5,7-dioxo-6-phenyl-1,5,6,7-tetrahydro-[1,2,4]triazolo[1,2-a][1,2,4]triazole-1-carboxylate(5e) C16H18N4O4S2
Yellow powder, yield = 32%, mp: 154–156 °C. FTIR (KBr), vmax: 3022, 2978, 2956, 2916, 1783, 1725, 1597, 1559, 1494, 1459, 1392, 1263, 1236, 1191, 1142, 1064, 1014, 965, 843, 795 cm−1. 1H NMR (250 MHz, CDCl3) δ: 7.39–7.54 (m, 5H), 3.83 (s, 3H), 2.61–2.68 (m, 4H), 2.58 (s, 3H), 2.15 (s, 3H) ppm. 13C NMR (62.5 MHz, CDCl3) δ: 166.50, 156.30, 153.40, 148.30, 130.60, 129.40, 129.00, 125.90, 94.10, 53.80, 35.50, 27.80, 15.70, 14.20 ppm. HRMS (ESI-Orbit trap) m/z: [M+H]+ calcd for C16H18N4O4S2+H, 395.0842; found 395.0843.
5.2.19 Methyl-1-benzyl-(3-metylthio)-5,7-dioxo-6-phenyl-1,5,6,7-tetrahydro-[1,2,4]triazolo[1,2-a][1,2,4]triazole-1-carboxylate (5f) C20H18N4O4S
Colorless powder, yield = 61%, mp: 152–154 °C. FTIR (KBr), vmax: 3110, 3068, 3032, 2960, 2944, 1788, 1732, 1570, 1496, 1402, 1282, 1212, 1144, 760 cm−1. 1H NMR (250 MHz, CDCl3) δ: 7.39–7.23 (m, 10H), 3.86 (s, 3H), 3.67 (d, 1H, J = 14.39 Hz), 3.57 (d, 1H, J = 14.39 Hz), 2.53 (s, 3H) ppm. 13C NMR (62.5 MHz, CDCl3) δ: 166.80, 155.30, 152.40, 147.50, 132.90, 131.20, 130.50, 129.20, 128.80, 127.40, 125.80, 93.90, 53.70, 40.50, 14.10 ppm. HRMS (ESI-Orbit trap) m/z: [M+H]+ calcd for C20H18N4O4S+H, 411.1122; found 411.1120.
5.2.20 Methyl-1-(4-methoxybenzyl)-3-(methylthio)-5,7-dioxo-6-phenyl-1,5,6,7-tetrahydro[1,2,4]triazolo[1,2-a][1,2,4]triazole-1-carboxylate (5g) C21H20N4O5S
Yellow oil, yield = 60%. FTIR (KBr), vmax: 3049, 2978, 2957, 2933, 2903, 2843, 1787, 1730, 1704, 1613, 1572, 1513, 1491, 1452, 1396, 1324, 1295, 1249, 1204, 1179, 1139, 1054, 1033, 1001, 953, 841, 753 cm−1. 1H NMR (250 MHz, CDCl3) δ: 7.17–7.47 (m, 5H), 7.17–7.36 (m, 2H), 6.80–6.85 (m, 2H), 3.88 (s, 3H), 3.64 (d, 1H, J = 14.51 Hz), 3.54 (d, 1H, J = 14.51 Hz), 2.57 (s, 3H) ppm. 13C NMR (62.5 MHz, CDCl3) δ: 166.90, 158.80, 155.20, 152.30, 147.50, 132.20, 130.50, 129.20, 128.80, 125.80, 124.70, 113.50, 94.0, 55.1, 53.7, 14.1 ppm. HRMS (ESI-Orbit trap) m/z: [M+H]+ calcd for C21H20N4O5S+H, 441.1227; found 441.1231.
5.2.21 Diethyl-3-(methylthio)-5,7-dioxo-6-phenyl-6,7-dihydro-[1,2,4]triazolo[1,2-a][1,2,4]triazole-1,1(5H)-dicarboxylate (5h) C17H18N4O6S
Yellow oil, yield = 61%. FTIR (KBr), vmax: 3069, 2982, 2965, 2928, 2872, 1799, 1739, 1578, 1496, 1476, 1402, 1370, 1295, 1264, 1226, 1184, 1126, 1102, 1049, 1012, 980, 862, 789 cm−1. 1H NMR (250 MHz, CDCl3) δ: 7.39–7.48 (m, 5H), 4.40–4.28 (m, 4H), 2.62 (s, 3H), 1.32 (t, 6H, J = 4.50 Hz) ppm. 13C NMR (62.5 MHz, CDCl3) δ: 163.60, 158.40, 152.50, 148.10, 130.30, 129.20, 129.00, 125.80, 92.10, 63.70, 14.30, 13.80 ppm. HRMS (ESI-Orbit trap) m/z: [M+H]+ calcd for C17H18N4O6S+H, 407.1020; found 407.1015.
5.2.22 7-(Methylthio)-2,5-diphenyl-[1,2,4]triazolo[1,2-a][1,2,4]triazole-1,3(2H,5H)-dione (7a) C17H14N4O2S
Colorless powder, yield = 57%, mp: 140–142 °C. FTIR (KBr), vmax: 3062, 3049, 3036, 2932, 2850, 1785, 1733, 1594, 1579, 1499, 1453, 1398, 1301, 1247, 1222, 1166, 1138, 1097, 1041, 1012, 852, 793, 741 cm−1. 1H NMR (250 MHz, CDCl3) δ: 7.26–7.53 (m, 10H), 6.82 (s, 1H), 2.63 (s, 3H). 13C NMR (62.5 MHz, CDCl3) δ: 169.50, 166.70, 157.70, 153.50, 149.20, 130.60, 129.30, 129.20, 128.90, 126.30, 120.00, 90.10, 53.80, 52.10, 34.70, 14.20 ppm. HRMS (ESI-Orbit trap) m/z: [M+H]+ calcd for C17H14N4O2S+H, 330.0910; found 330.0909.
5.2.23 5-(4-Fluorophenyl)-7-(methylthio)-2-phenyl [1,2,4]triazolo[1,2-a][1,2,4]triazole-1,3(2H,5H)-dione (7b) C17H13FN4O2S
Colorless powder, yield = 49%, mp: 156–158 °C. FTIR (KBr), vmax: 3076, 3013, 2970, 2925, 2851, 1787, 1735, 1606, 1578, 1510, 1459, 1402, 1310, 1256, 1225, 1173, 1141, 1107, 1048, 1016, 873, 781, 748 cm−1. 1H NMR (250 MHz, CDCl3) δ: 7.53–6.80 (m, 9H), 6.79 (s, 1H), 2.63 (s, 3H) ppm. 13C NMR (62.5 MHz, CDCl3) δ: 155.70, 155.00, 150.40, 165.10, 161.10, 133.50, 130.50, 129.40, 129.30, 128.90, 128.40, 128.30, 125.50, 125.10, 116.00, 115.60, 86.00, 14.40 ppm. HRMS (ESI-Orbit trap) m/z: [M+H]+ calcd for C17H13FN4O4S+H, 357.0816; found 357.0811.
5.2.24 5-Methyl-7-(methylthio)-2,5-diphenyl-[1,2,4]triazolo[1,2-a][1,2,4]triazole-1,3(2H,5H)-dione (7c) C18H16N4O2S
Yellow powder, yield = 53%, mp: 163–165 °C. FTIR (KBr), vmax: 3063, 2987, 2957, 2930, 2851, 1784, 1722, 1590, 1577, 1493, 1447, 1390, 1316, 1253, 1137, 1072, 1022, 833, 754 cm−1. 1H NMR (250 MHz, CDCl3) δ: 7.36–7.67 (m, 10H), 2.65 (s, 3H), 2.10 (s, 3H) ppm. 13C NMR (62.5 MHz, CDCl3) δ: 151.90, 151.40, 148.00, 142.10, 130.80, 129.20, 128.60, 128.50, 128.40, 125.60, 125.40, 94.20, 27.60, 14.10 ppm. HRMS (ESI-Orbit trap) m/z: [M+H]+ calcd for C17H16N4O2S+H, 353.1067; found 353.1061.
5.2.25 7-(Methylthio)-2,5,5-triphenyl-[1,2,4]triazolo[1,2-a][1,2,4]triazole-1,3(2H,5H)-dione (7d) C23H18N4O2S
Yellow powder, yield = 53%, mp: 166–168 °C. FTIR (KBr), vmax: 3070, 3050, 2977, 2959, 2920, 2850, 1792, 1732, 1706 (CO), 1595, 1580, 1490, 1451, 1428, 1393, 1315, 1242, 1181, 1164, 1141, 1116, 1087, 1045, 1009, 832, 778, 741 cm−1. 1H NMR (250 MHz, CDCl3) δ: 7.33–7.51 (m, 15H), 2.71 (s, 3H) ppm. 13C NMR (62.5 MHz, CDCl3) δ: 152.50, 151.10, 148.00, 139.40, 130.70, 129.10, 129.00, 128.60, 128.30, 127.50, 125.50, 97.90, 14.20 ppm. HRMS (ESI-Orbit trap) m/z: [M+H]+ calcd for C23H18N4O2S+H, 415.1223; found 415.1210.
5.2.26 Dimethyl(3,5-dioxo-4-phenyl-1,2,4-triazolidin-1-yl) (phenyl) methylcarbonimidodithioate (8a) C18H18N4O2S2
White powder yield = 19%, mp: 123–125 °C. FTIR (KBr), vmax: 3132, 3070, 3054, 2916, 2850, 1764, 1686, 1450, 1268, 1140, 980, 728. 1H NMR (250 MHz, CDCl3) δ: 7.36–7.51 (m, 10H), 6.42 (s, 1H), 2.67 (s, 3H), 2.28 (s, 3H). 13C NMR (62.5 MHz, CDCl3) δ: 159.2, 153.1, 152.3, 134.9, 130.9, 130.1, 129.3, 129.2, 129.0, 128.8, 128.4, 127.4, 126.4, 125.5, 65.7, 15.1, 15.0. HRMS (ESI-Orbit trap) m/z: [M+H]+ calcd for C17H13FN4O4S+H, 387.0944; found 387.0944.
5.3 Biological evaluation
All the reagents used were commercially available by Merck, 1,1-diphenyl-2-picrylhydrazyl (DPPH), nordihydroguaiaretic acid (NDGA) were purchased from the Aldrich Chemical Co. Milwaukee, WI, (USA). Soybean lipoxygenase, linoleic acid sodium salt, indomethacin were obtained from Sigma Chemical, Co. (St. Louis,MO, USA).
5.3.1 Soybean lipoxygenase inhibition study in vitro
(Lit. [36]) The in vitro study was evaluated as reported previously. The test compounds dissolved in ethanol were incubated at room temperature with sodium linoleate (100 μM) and 0.2 mL of enzyme solution (1/9 × 10−4 w/v in saline). The conversion of sodium linoleate to 13-hydroperoxylinoleic acid at 234 nm was recorded and compared with the appropriate standard inhibitor (nordihydroguairetic acid 45 μM) (Table 5).
5.3.2 Inhibition of linoleic acid peroxidation
(Lit. [36]) Production of conjugated diene hydroperoxide by oxidation of linoleic acid in an aqueous dispersion was monitored at 234 nm. 2, 2′-Azobis(2-amidinopropane) dihydrochloride (AAPH) is used as a free radical initiator. This assay can be used to follow oxidative changes and to determine the inhibition of linoleic acid peroxidation induced by each tested compound. Ten microliters of the 16 mM linoleic acid dispersion were added to the UV cuvette containing 0.93 mL of 0.05 M phosphate buffer, pH 7.4, thermostatted at 37 °C. The oxidation reaction was initiated at 37 °C under air by the addition of 50 μL of 40 mM AAPH solution. Oxidation was carried out in the presence of aliquots (10 μL) of the tested compounds. In the assay without antioxidant, lipid oxidation was measured in the presence of the same level of DMSO. The rate of oxidation at 37 °C was monitored by recording the increase in absorption at 234 nm caused by conjugated diene hydroperoxide formation (Table 5).
Acknowledgements
We thank the MS centers at the Universities of Wroclaw and Ioannina, and the NMR center at the University of Ioannina for the spectra. We thank Biobyte and Dr. Leo for the free access to the C-QSAR program.