1 Introduction
Encapsulation of metallocomplexes inside nanoporous materials such as zeolites is a good way to increase and control their selectivity. The framework of these nanoporous materials restricts their active species, thereby resulting in higher stability. It leads to reduction in the self-destruction and degradation of metallocomplexes [1–9]. Zeolites are good candidates due to their molecular-size channels and nanopores in well-defined crystalline structures. These structures confer shape and size selectivity and therefore, can be employed as enzymatic models. In this case, the framework of zeolite indicates a rigid protein mantle of enzymes and metallocomplexes act as active site enzymes, which catalyze a variety of reactions [10–17].
An obvious example of the above systems is the usage of porphyrin complexes in the faujasite framework as catalysts in the oxidation reactions [18–20].
Upon the formation of metalloporphyrins into the nanocage of zeolite, the complex may obtain a unique configuration, different from the solution form. In this case, cationic metalloporphyrins stabilized inside the negative framework of the zeolite supercages, through an electrostatic interaction [19–21]. These catalytic systems are used in the epoxidation, hydroxylation and photochemical reactions [8,16–19,22–27]. In this study, however, here, Y zeolite encapsulated-Zr porphyrin is used in the aldehyde oximation reaction for first time. Oximation reactions were performed under acidic conditions [28–30]. Zirconium (IV) is redox inactive and enhances Lewis acid strength, imparted by its +4 oxidation state. This feature of zirconium enables Zr (IV) ions to function effectively in acid-catalyzed reactions [31]. Here Y zeolite was dealuminated in order to inhibit zirconium hydrolysis and facilitate the formation of metallocomplexes, inside zeolite cages.
In continuation of our previous studies on the encapsulation of metallocomplexes into the nanocage of zeolites [8,18,19], here, we wish to report the synthesis, physicochemical characterization and catalytic activity of dealuminated Y zeolite encapsulated-Zr porphyrin in the aldehyde oximation at room temperature under magnetic stirring conditions.
2 Experimental
2.1 Preparation of ZrTPP–DAZY
All reagents and solvents were of commercial grade and were obtained from Sigma–Aldrich, Fluka and Merck. Dealumination of the Y zeolite (DAZY) was performed using H4EDTA by chemical treatment in accordance with the reported procedure [19]. The dealuminated Y zeolite was washed with hot distilled water, and dried and ultimately was used in the preparation of zirconium porphyrin inside the supercage of DAZY. In a typical procedure, to the solution of ZrCl4·4H2O (0.3 g) in ethanol (25 mL), 1 g DAZY zeolite was added and stirred at room temperature for 24 h. In the next step, Zr ions exchanged with zeolite were filtered, washed thoroughly with distilled water and then dried at 373 K overnight. The obtained materials were filtered and then washed with hot distilled water. In order to prepare zirconium tetraphenylporphyrin in the nanocage of zeolite, ZrY (1 g), benzaldehyde and pyrrole were mixed in a molar ratio of 1:1 in 50 mL of propionic acid in accordance with the reported procedure [18]. The mixture was refluxed in an oil bath for 18 h at 455 K under stirring and then filtered. The crude materials were purified with a Soxhlet extractor to remove the unreacted species. In order to eliminate the unreacted species of Zr cations in the zeolite pores, the sample was re-exchanged with aqueous 0.01 M solution of NaCl for 4 h under vigorous stirring. Finally, the obtained solid materials were filtered, and washed with distilled water, and then dried at 363 K. To specify the location of ZrTPP in the zeolite nanocage, the sample was analyzed using spectroscopic techniques such as XRD, FT-IR, UV–Vis, ICP, FESEM and EDX.
2.2 General procedure for the preparation of the Z-isomer of oximes in the presence of ZrTPP–DAZY under mechanical stirring
In a typical procedure, hydroxylamine hydrochloride (1.2 mmol), aldehydes (1 mmol), 0.6 g ZrTPP–DAZY equal 0.012 mmol ZrTPP and ethanol (5 mL) were mixed and stirred at room temperature. The progress of the reaction was monitored by TLC. After completion of the reactions, the catalyst was filtered and washed with ethanol, and activated at 373 °C for 10 h. For the extraction of organic materials, sodium bicarbonate (10%, 10 mL) was added to the filtrates and the organics were extracted with cold diethyl ether (25 mL) and then was dried to obtain Z-aldoximes. The crude materials were recrystallized from ethanol to yield pure oximes. These products were characterized by physical constants such as melting points and usual spectral techniques (Scheme 1).
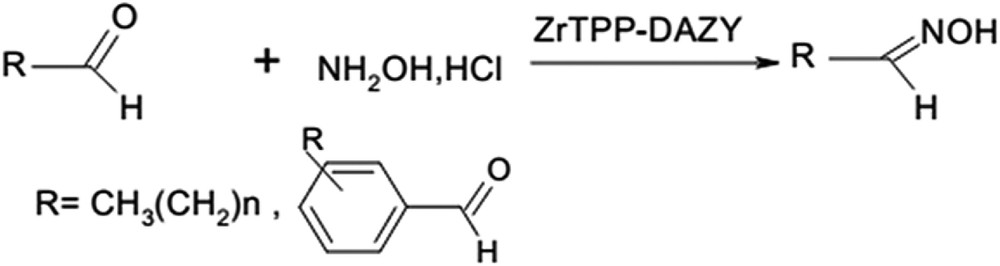
Oximation of aldehyde using ZrTPP–DAZY under mechanical stirring at room temperature.
3 Results and discussion
3.1 Preparation and characterization of ZrTPP–DAZY
Zr (IV)-porphyrin encapsulated into the nanocage of dealuminated Y zeolite was obtained through a template synthesis method [18]. In this method, ligand condensation occurs around the intrazeolite metal ion and produces the complexes with dimensions higher than the zeolite cavity, so that metallocomplexes are entrapped in the zeolite nanocage and cannot leave the nanocage of zeolite.
In this way, four pyrrole molecules diffuse together with four benzaldehyde molecules inside the matrix of zeolite, and the porphyrin ring is formed around the metal ion, which previously entered the nanocage of zeolite through an ion exchange method. In this case, the complex becomes too large to escape the zeolite cage. The crude material was purified by Soxhlet extraction with ethanol, acetone and methanol to removal the excess ligand and surface adsorbed complexes. In addition, the original color of zeolite changed into purple and remained the same after being washed by Soxhlet extraction and thus, proved the incorporation of Zr-porphyrin into the zeolite nanocage.
The content of ZrTPP in the nanocage of DAZY was obtained 0.0156 g/g equal 2 mmol/100 g of the supported catalyst based on the Zr content of ZrTPP–DAZY.
A diffuse reflectance spectroscopic (DRS) technique was used to confirm the intrazeolite complexes. UV–Vis spectra of ZrTPP and TPP and DR spectrum of ZrTPP–DAZY are presented in Table 1 for comparison. The characteristic bands of zirconium (IV) tetraphenylporphyrin (Q and Soret bands) were observed in all of the spectra. Based on the classification of electronic spectra of metalloporphyrins, TPP and ZrTPP are categorized as normal. Gouterman explained normal spectra by using the orbital's model [32]. In the Zr-TPP compound, there are four orbitals (a1u, a2u and eg) and LMCT is related to a1u-dxy (Fig. 1). In the TPP case, one intense absorbance (the Soret or B-band) at 418 nm and four absorbencies (Q bands) at 515, 550, 590, 647 nm appear. Upon the complexation of TPP with Zr into the nanocage of Y zeolite, the band number in Q bands is reduced (407 and 541 nm is related to Soret and Q bands, respectively). The point group and symmetry of ZrTPP (D4h) decrease to (D2h) in TPP due to the presence of hydrogen in the position of pyrrole nitrogen [26,33]. Blue shifts in the positions of peak, however, can be related to mixing and interaction of orbitals eg (π–π∗) and 4d of metal. The DR spectrum of ZrTPP–DAZY indicated two bands at 395 and 490 nm, which proved the encapsulation of Zr-TPP into the nanocage of zeolite with blue shifts due to the ring strain [9].
Amount of metalloporphyrins in the DAZY, DRS and UV–Vis absorption bands for ZrTPP.
Entry | Catalyst | Metalloporphyrin loading (mmol/100 g zeolite) | Wavelength (nm) | |
Soret band | Q-band | |||
1 | TPP | – | 418 | 515, 541, 550, 590 |
2 | ZrTPP | – | 407 | 541 |
3 | ZrTPP–MDAZY | 2 | 395 | 490 |
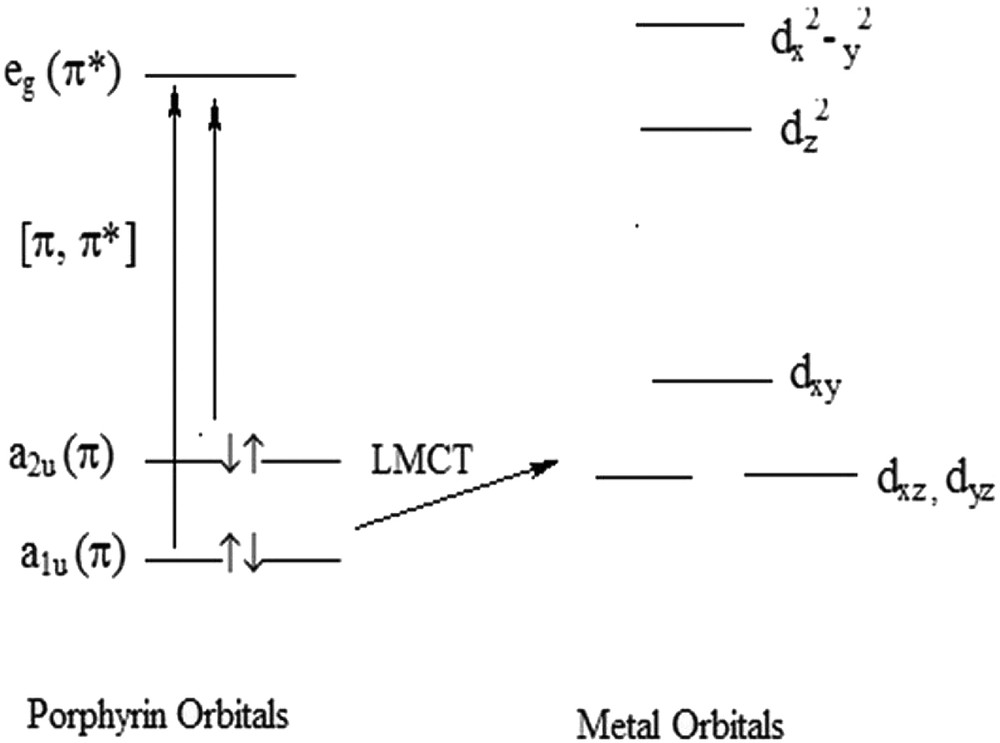
Molecular orbital diagram for d-type normal metalloporphyrins (adapted from Ref. 26).
Fig. 2 presents the XRD pattern of dealuminated Y zeolite and dealuminated Y zeolite–encapsulated zirconium porphyrin (ZrTPP–DAZY), which is an indication of similar crystallinity to that of parent DAZY. The encapsulation of metalloporphyrin in the nanocavity of zeolite has no effect on the zeolite crystallinity. This fact can be attributed to well-dispersion of metallocomplexes in the zeolite lattice [34,35].
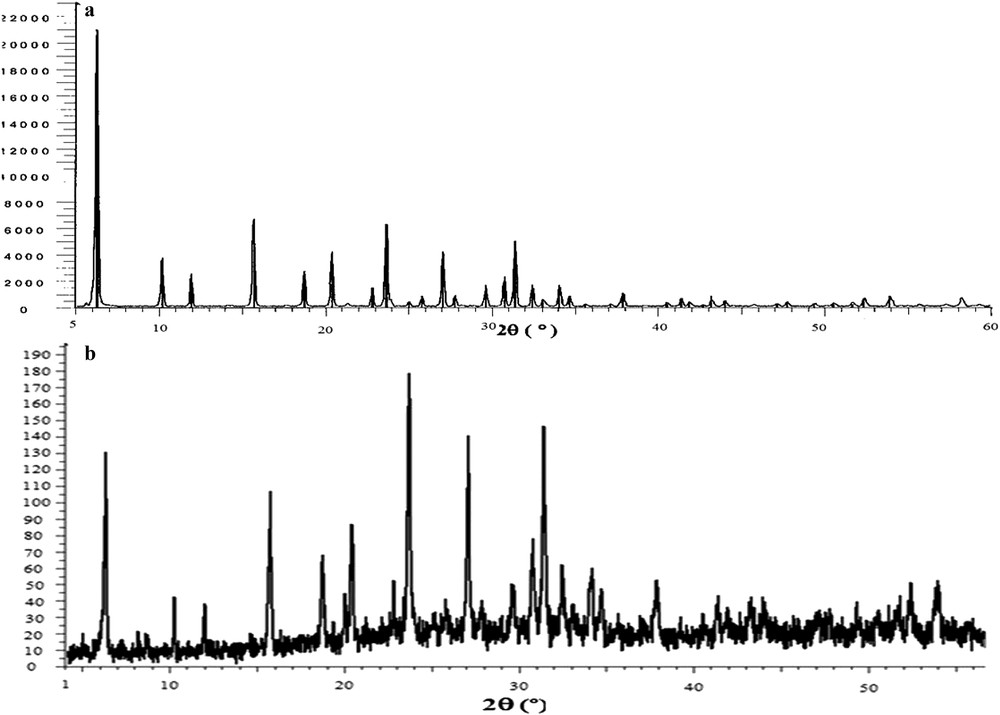
XRD patterns of a) DAZY and b) ZrTPP–DAZY.
The FT-IR spectra can also offer evidence about the formation of ZrTPP inside the zeolite nanocage. The FT-IR data of DAZY, ZrTPP, ZrY and ZrTPP/DAZY are presented in Fig. 3. As it was described in detail previously, the bands in the range of 500–1200 and 1637 cm−1 are related to the vibration of the internal and external lattice linkages of zeolite, respectively. In addition, the bands at 3200–3600 cm−1 are related to lattice water molecules and hydroxyl groups of dealuminated Y zeolite. The FT-IR bands of ZrTPP in ZrTPP–DAZY are weak because of their low concentration and the respective peaks were overlapped by vibrations of the zeolite support. However, the bands in the regions of 1200–1600 and 2800–3200 cm−1 are assigned to CC and CN vibrations of the porphyrin ring; and CH vibrations for ZrTPP, respectively [18,19]. Moreover, ZrO and ZrN bands are located in the fingerprint position, in the region of 500 cm−1, which is not presented in these figures [36,37].
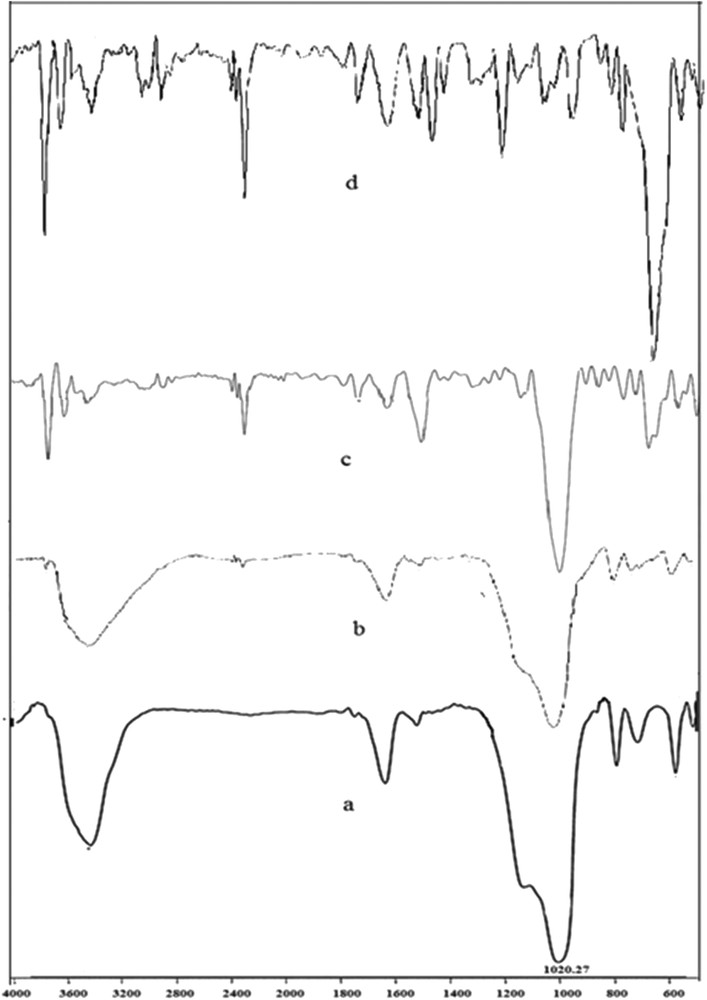
FT-IR spectra of a) DAZY, b) ZrY, c) ZrTPP–DAZY, and d) ZrTPP.
Field emission scanning electron microscopy (FESEM) of ZrTPP–DAZY indicated that the cubo-octahedral units which were improved during the formation of metallocomplexes into the zeolite cage remained unchanged. In addition, the absence of metallocomplexes on the zeolite surface indicated that the metallocomplexes have formed inside the nanocavity of zeolite, not on the external surface (Fig. 4).

FESEM micrograph of ZrTPP–DAZY.
The FESEM-EDX spectrum of ZrTPP–DAZY also confirmed the formation of metallocomplexes in the zeolite cages (Fig. 5).
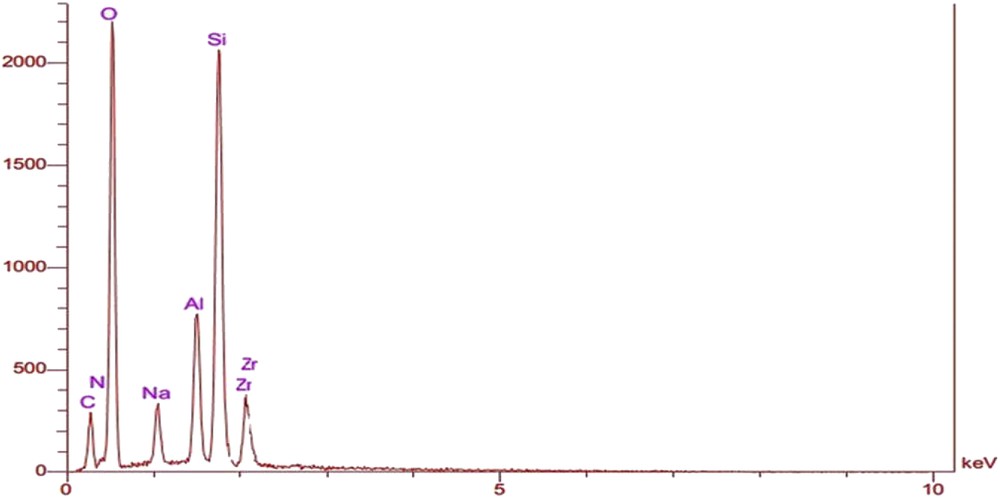
EDS analysis of ZrTPP–DAZY.
3.2 The effect of catalyst amount
For this purpose, the amount of catalyst was optimized in the oximation of butyraldehyde with hydroxylamine hydroxide. The results indicated that the best results were obtained in the presence of 0.6 g of the catalyst equal to 0.012 mmol ZrTPP (Table 2). In addition, the effect of temperature on the reaction was studied. The results indicated that the occurrence of these reactions requires moderate conditions rather than hard conditions (Table 3).
The effect of catalyst amount on oximation yield of butyraldehyde.
T(°C) | Time (h) | Yielda (%) |
r.t | 3 | 65 |
70 | 3 | 70 |
90 | 3 | 70 |
a Isolated yield.
The effect of temperature on oximation yield of butyraldehyde.
Catalyst amount | Time (h) | Yielda (%) |
0.2 | 3 | 25 |
0.4 | 3 | 45 |
0.6 | 3 | 70 |
0.8 | 3 | 70 |
a Isolated yield.
The control experiment was performed under different conditions including a free catalyst, pristine zeolite, neat ZrTPP and ZrTPP–DAZY. The results indicated that under free catalyst conditions, no progress was observed in the reaction. In the presence of Y zeolite, the yield of the reaction was only 35%; ZrTPP is a good catalyst system, however, after being used once, it becomes inactive because of the dimerization or disproportionation of porphyrin. Therefore, the heterogenous system is preferred due to high yield and recoverability (Table 4).
3.3 Oximation of aldehydes using ZrTPP–DAZY under mechanical stirring
Under the optimized reaction conditions, a wide range of aromatic and aliphatic aldehydes reacted with hydroxylamine hydrochloride in the presence of ZrTPP–DAZY under magnetic stirring at room temperature to attain the corresponding Z-aldoximes in 50–90% yields. The results (Table 5) indicated that the increase in length of hydrocarbon chain leads to lower oximation yield. It can be concluded that the zeolite structure is sensitive to chain length. It seems that as the length of chain increases, the mobility of aldehyde is reduced and therefore, the accessibility to the active site will be difficult in a way that the lowest yield belongs to heptanol with the longest chain and the highest yield belongs to acetaldehyde with a shorter chain length amongst these compounds. Furthermore, in the case of aromatic aldehydes, the nature of the substituents (electron-donating or electron-withdrawing groups) has no significant influence on the product yield (Table 5). In all of these cases, the Z-aldoxime was obtained without any by-product and was proved by comparing their melting point and 1H-chemical shift of the C(H)N group.
Oximation of aldehydes with NH2OH·HCl catalyzed by ZrTPP–DAZY under mechanical stirring conditions.a
Entry | Substrate | Time (min) | Yieldb (%) | M.P. (L)/(B.P) (°C) | 1H chemical shift | |
Observed | Reported | |||||
1 | 180 | 80 | 119 | 121 [28] | 8.16 | |
2 | 180 | 75 | 123 | 126–128 [28] | 8.21 | |
3 | 180 | 80 | 146 | 146 [30] | 8.11 | |
4 | 180 | 70 | 120 | 115–120 [38] | 6.84 | |
5 | 20 | 90 | 117 | 115 [39] | 6.92 | |
6 | 180 | 70 | 148 | 152 [39] | 6.73 | |
7 | 180 | 50 | 47 | 44–46 [40] | 6.72 |
a Reaction conditions: aldehyde (1 mmol), catalyst (0.6 g, 0.025 mmol) and NH2OH·HCl (1.2 mmol).
b Isolated yield.
The reusability of ZrTPP–DAZY was tested using 4-chloro benzaldehyde as a model substrate under mechanical stirring. At the end of the reaction, the catalyst was filtered, washed using ethanol, dried and activated at 110 °C for 3 h. The recovered catalyst was reused in the oximation of fresh 4-chloro benzaldehyde. The results indicated that after five runs, this catalytic system preserved the catalytic activity. This, in turn, can be attributed to the encapsulation of metallocomplexes into the nanocage of zeolite, which minimizes the leaching of metallocomplexes and therefore, the catalytic activity is maintained (Table 6).
The results attained in the reusability of ZrTPP–DAZY catalyst recovery in the oximation of 4-chlorobenzaldehyde.a
Run | Time (min) | Yieldb (%) |
1 | 180 | 80 |
2 | 180 | 75 |
3 | 180 | 75 |
4 | 180 | 70 |
5 | 180 | 67 |
a Reaction conditions: 4-chloro benzaldehyde (1 mmol), catalyst (0.6 g, 0.025 mmol) and NH2OH·HCl (1.2 mmol).
b Isolated yield.
4 Conclusion
In this article, ZrTPP was synthesized inside the nanocage of DAZY by a template synthesis method. NaY zeolite was dealuminated in order to inhibit zirconium hydrolysis in order to facilitate the formation of Zr porphyrin inside a zeolite nanocage. In addition, dealumination leads to the production of a mesoporous structure and therefore, facilitating the formation of the catalyst. The catalyst amount in the nanocage of zeolite was 0.0156 g/g support (2 mmol/100 g zeolite). This catalytic system was characterized using XRD, FT-IR, FESEM, EDS, DRS, UV–Vis and ICP techniques. The catalytic activity of ZrTPP–DAZY was examined in the oximation of aromatic and aliphatic aldehydes. The results indicated that in aliphatic aldehydes, when the chain length increases, the yield of the reaction is reduced. This effect can be attributed to the accessibility to the active site, in a way that with the increase in the chain length, the mobility is reduced and therefore, the oximation of aldehyde is decreased and the reaction yield is also reduced.
In this method, as compared with other methods, high loading of catalyst is attributed to the small size of metalloporphyrin. In other words, an increasing mesoporous structure diameter is related to the removal of aluminum species of an extra-framework from the zeolite nanocage. Zirconium tetraphenylporphyrin encapsulated into dealuminated Y Zeolite (ZrTPP–DAZY) was recycled several times and reused without losing its catalytic activity. This protocol can be considered as a green catalytic system for the efficient oximation of aliphatic and aromatic aldehydes under stirring conditions.