1 Introduction
Fumed silica (FSi) is a nanosized and highly dispersed synthetic silicon dioxide product [1]. It consists of very fine agglomerated aggregates, which are produced via the flame hydrolysis of chlorosilanes. The size of primary FSi particles in the aggregates is about 10 nm, whereas the agglomerated particles have sizes in the range of 100 nm–5 μm. From an industrial point of view, FSi is used as reinforcement of elastomers, thickening of liquids, antifoaming, paper coating, and adsorbent [2]. The density of silanol groups on FSi surface is low, but they are distributed over the surface, and thus, its surface is highly reactive to chemical reactions and has a hydrophilic character. Silylation of these silanol groups is the main reaction of FSi. The modified silica compounds are widely used as catalysts [3], optical materials [4], coating agents [5], chemosensors [6], proton exchange membranes [7], and organic light-emitting devices [8].
Sulfonamide compounds were discovered in 1935 [9], and nowadays are extensively used for the treatment of infections in human [10], aquaculture, livestock production [11], catalysis [12], and organic syntheses [13]. Regarding the importance of antibacterial surfaces and implants, antibiotic-loaded coatings are currently considered as the attractive materials in biomedical engineering [14]. So far, sulfonamide-loaded polymeric materials have been widely studied and used as membranes [15]. There are just a few publications on the modification of silica surface with sulfonamides; for example, Schick and Sun [16] just studied and characterized sulfonamide modified silica, whereas Piscopio and coworkers used it for solid phase synthesis [17]. In continuation of our previous research [18], herein, we tried to modify the FSi surface with sulfonamides by the use of silylating agents and then investigating their antibacterial properties.
2 Experimental section
2.1 Materials and instruments
3-(Chloropropyl)trimethoxysilane (ClPTMS), 3-(aminopropyl)trimethoxysilane (APTMS), and FSi were purchased from Sigma–Aldrich Company. All aforementioned materials were used without any further purification. The Fourier transform infrared (FT-IR) spectra of samples were recorded on NaCl disks by a FT-IR Bruker Tensor 27 instrument. The surface morphologies of samples were observed using a field emission scanning electron microscope (Hitachi S-4160 Japan). Thermogravimetric analysis (TGA) was carried out by a BӒHR Thermoanalyse, STA503 model from ambient temperature to 1000 °C with a ramp rate of 10 °C min−1 in air. Zeta potential was obtained using a Malvern Instrument. Escherichia coli and Staphylococcus aureus were prepared from Iranian Research Organization for Science and Technology and used as the antibacterium model.
2.2 General procedure for modification of FSi with aminopropyl (FSiPrNH2) and/or propylchloride (FSiPrCl) groups
FSi (10 g) was poured into a two-neck round-bottom flask and dried well under vacuum pressure at 100 °C for about 2 h. Afterward, when it reached to ambient temperature, dry toluene (150 mL) was added to it until a dilute mixture was obtained. The mixture was heated under reflux condition for about 30 min and then ClPTMS and/or APTMS (6 mL) was gradually added to it and the mixture was refluxed for about 72 h. Finally, toluene was evaporated under reduced pressure to obtain the crude functionalized FSi, which was washed well with CH2Cl2 by the use of a Soxhlet apparatus and then dried at ambient temperature overnight.
2.3 General procedure for the modification of FSiPrCl with diamine compounds
FSiPrCl (6 g) was poured into a two-neck round-bottom flask and dried under reduced pressure at 100 °C for removal of the adsorbed water. Then, N,N-dimethylformamide (50 mL) and piperazine or p-phenylenediamine (12 g) were added to it; subsequently, the mixture was heated under reflux condition for about 72 h. Afterward, the solvent was evaporated and the crude solid was washed well with EtOH using a Soxhlet apparatus and then dried at ambient temperature overnight. Finally, the modified FSi with piperazine (FSiPrPi) or p-phenylenediamine (FSiPrPhDA) was obtained.
2.4 General procedure for the preparation of sulfonamide-modified FSi
Two grams of FSiPrNH2, FSiPrPi, or FSiPrPhDA was dried under reduced pressure at 100 °C and then the corresponding sulfonyl chloride 2a–c (4 g), K2CO3 (0.5 g), and EtOH (15 mL) were added to it and stirred at room temperature for 24 h. Subsequently, the obtained solid was filtered, washed well with CH2Cl2 using a Soxhlet apparatus, and dried at room temperature.
2.5 Antibacterial tests
2.5.1 Culture conditions
Several colonies from an overnight culture on a nutrient agar plate were subcultured in 15 mL of Tryptone Soy Broth for 24 h at 37 °C Then, this overnight culture (500 μL) was cultured again in Tryptone Soy Broth (20 mL), and the cells were grown until mid-log phase (optical density at 600 nm, 0.5–0.6). Then, 15 mL of the mid-log-phase culture was centrifuged at 4000g for 18 min at 4 °C and the spent culture medium was discarded [19].
2.5.2 Microbial inhibitory concentration
Overnight cultures of E coli and S. aureus were diluted to obtain an optical density (OD600) of 0.2 (corresponding to 1 × 108 colony-forming units per milliliter). The inoculum (1 mL for each) was added to vials containing serial dilutions of a nanostructure solution with the concentration of 1 mg/mL (experiments performed in triplicate). A control group of bacteria were cultivated in the absence of nanostructure solution. After incubation for 24 h at 30 °C, bacterial growth was monitored by recording the OD600 using a UV–vis spectrophotometer [20]. The minimum inhibitory concentration (MIC) was defined as the lowest concentration of sulfonamide-modified FSi that could completely inhibit the bacteria growth. Nanostructure solution was prepared through the dispersion of nanostructure (0.01 g) in DMSO (10 mL) under ultrasonic irradiation (50 W power for about 20 min).
3 Results and discussion
3.1 Preparation and characterization of sulfonamide-modified FSi
In this study, for preparation of sulfonamide-modified FSi, the FSi surface was first functionalized with different amine. In this regard, FSi was treated with APTMS and/or ClPTMS to give FSiPrNH2 and/or FSiPrCl, respectively (Scheme 1). Then, FSiPrCl was reacted with piperazine 1a or p-phenylenediamine 1b to gain FSiPrPi and FSiPrPhDA, respectively (Scheme 2). Subsequently, the prepared amine-functionalized FSi compounds were reacted with sulfonyl chlorides 2a–c to achieve the target sulfonamide-modified FSi compounds (Scheme 3, Table 1), which then were characterized by varied techniques.
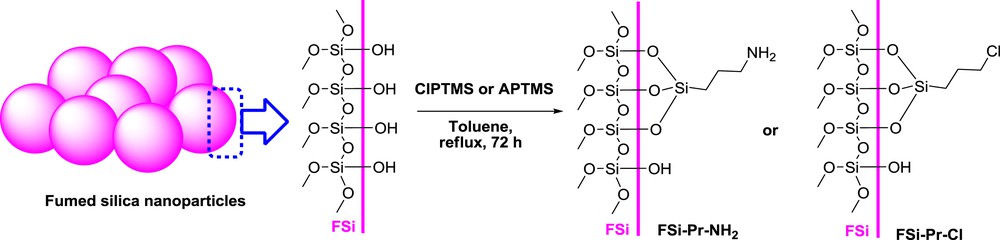
Modification of FSi surface with different amines.

The reaction of FSiPrCl with diamines.

The synthetic route for preparation of sulfonamide-modified FSi.
The obtained sulfonamide modified-FSi compounds.
Entry | Substrate | Sulfonyl chloride | Name |
1 | FSiPrNH2 | 2a | SNA1 |
2 | FSiPrNH2 | 2b | SNA2 |
3 | FSiPrNH2 | 2c | SNA3 |
4 | FSiPrPi | 2a | SNB1 |
5 | FSiPrPi | 2b | SNB2 |
6 | FSiPrPi | 2c | SNB3 |
7 | FSiPrPhDA | 2a | SNC1 |
8 | FSiPrPhDA | 2b | SNC2 |
9 | FSiPrPhDA | 2c | SNC3 |
3.2 FT-IR spectroscopy
Figs. 1–3 depict the FT-IR spectra of amino and sulfonamide-functionalized FSi. In general, nanosilica materials display three intense peaks at 1100, 960, and 800 cm−1, which are corresponded to the asymmetric stretching vibrations of SiOSi, symmetric stretching vibration of SOH, and symmetric stretching vibrations of SiO, respectively. The presence of organic moieties was established through the peaks at 2850–2960 cm−1 in all spectra, which are related to the symmetric and asymmetric stretching vibration of aliphatic CH. In Fig. 1, the absorbance bands at 3292 and 1544 cm−1 belong to the stretching and bending of NH2, respectively, which were disappeared in spectra of SNA1, SNA2, and SNA3 confirming formation of sulfonamide bond in these materials. Also, the absorbance bands at 1656, 1593, and 1527 cm−1, respectively, belong to the stretching of CO, out of plane vibration of NH, and CH3 bending vibrations which are for the acetamide moiety of SNA3, SNB3, and SNC3. The absorbance bands in the range of 675–686 cm−1 correspond to the out of plane vibration of CH in aromatic rings. As shown in Fig. 2, the absorbance bands at 2767 cm−1 are related to the CH2 stretching in piperazine ring, confirming the presence of piperazine moiety grafted on the surface of FSi. The peak at 1660 cm−1 is for the vibration of H2O molecules, which were absorbed by piperazine molecules through hydrogen; piperazine in its free form exists as a hexahydrated form. In addition, the CC stretching in piperazine ring was displayed at 1448 cm−1. In Fig. 3, the pointed peak at 1625 cm−1 is for in-plane bending of NH2 in p-phenylenediamine moiety, which was disappeared in the spectra of SNC1, SNC2, and SNC3. Unfortunately, the SiOSi vibration at 1100 cm−1 covers all vibration peaks of the formed sulfonamide, although the mentioned evidence confirmed the formation of sulfonamide bond in the corresponding materials.
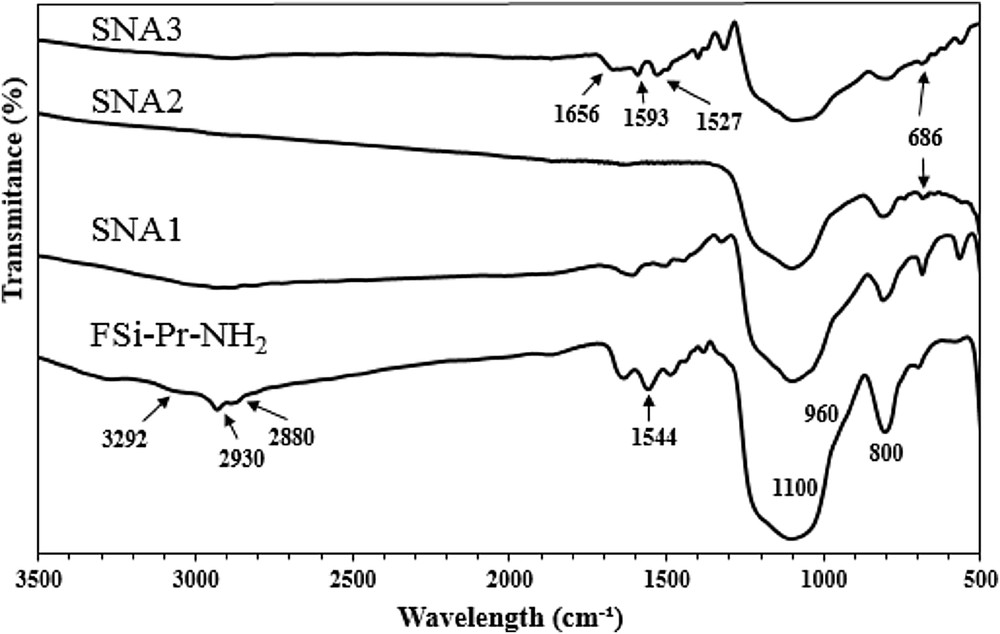
FT-IR spectra of FSiPrNH2 and its sulfonamides.

FT-IR spectra of FSiPrPi and its sulfonamides.
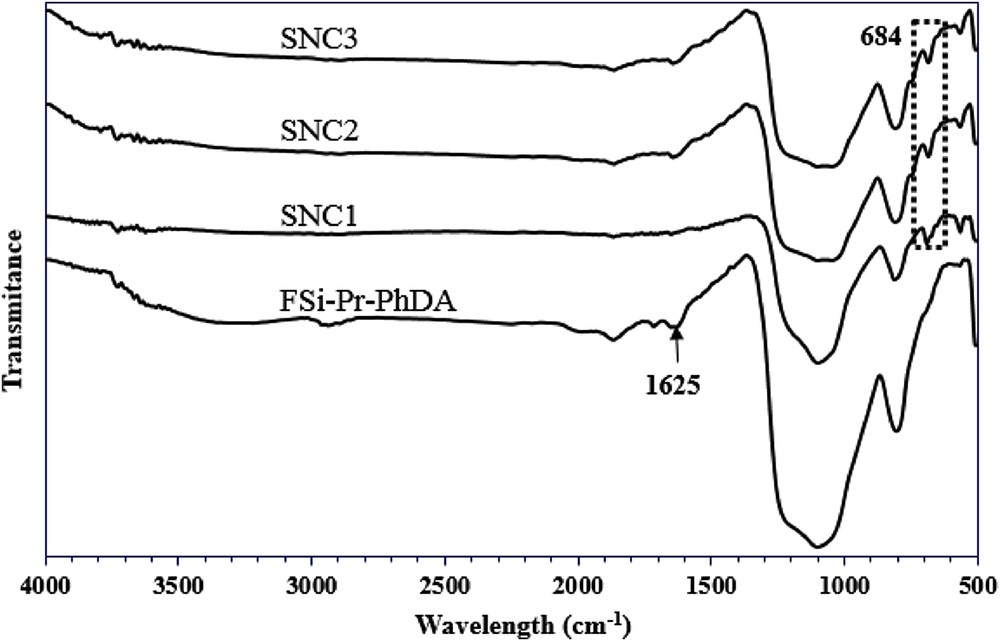
FT-IR spectra of FSiPrPhDA and its sulfonamides.
3.3 TGA and differential thermal analysis
The TGA was used to estimate the amount of grafted organic compounds on the surface of FSi. Figs. 4–6 provide the TGA–differential thermal analysis (DTA) curves of functionalized FSi compounds. According to Fig. 4, the first mass loss happened for FSiPrNH2 between 100 and 300 °C corresponds to an endothermic peak at 288 °C in the DTA plot. This mass loss is because of the removal of physically absorbed water molecules and used solvent in the synthetic procedure that they were not removed during drying process. Correspondingly, this mass loss is seen in the TGA curves of SNA1, SNA2, and SNA3, whereas their exothermic points display about 100 °C shift. However, SNA1 shows more weight loss in this range approving the more adsorbed water or solvent on its surface. Additional weight loss in FSiPrNH2, which occurs from the end of first mass loss with an exothermic peak till around 340 °C, is reflected by an endothermic peak at 298 °C, which might be attributed to the decomposition of the organic groups. This weight loss was also happened in SNA1-3 with a sharper rate confirming formation of sulfonamide bonds. The weak mass drop (onset at 640 °C) till around 675 °C is because of the condensation of silanol groups. Subsequently, the weight loss at the temperature range of 280–600 °C indicates that the amount of organic groups in FSiPrNH2, SNA1, SNA2, and SNA3 is 2.07, 1.41, 1.09, and 1.02 mmol/g, respectively. Consequently, some amine groups were not reacted with the corresponding sulfonyl chlorides. Therefore, both propylamine and sulfonamides are present on the modified surface of FSi compounds.

(Left) TGA and (Right) DTA curves of FSiPrNH2 and its sulfonamide materials.

(Left) TGA and (Right) DTA curves of FSiPrCl, FSiPrPi, and its sulfonamide materials.

(Left) TGA and (Right) DTA curves of FSiPrCl, FSiPrPhDA, and its sulfonamide materials.
The additional explanations for Figs. 5 and 6 are as follows: the TGA curve for FSiPrCl depicts initial exceeding of the weight from 100%, which is because of the buoyancy effect. The weight loss of FSiPrCl for organic moieties was calculated as 0.91 mmol/g. Similarly, the calculated organic weight loss for FSiPrPi and FSiPrPhDA was 0.94 and 0.2 mmol/g, respectively. This shows that probably all propylchloride moieties were completely treated with piperazine, whereas the organic moieties on the surface of FSiPrPhDA were leached because of the vigorous mechanical stirring. Also, the organic weight loss for SNB1, SNB2, SNB3, SNC1, SNC2, and SNC3 was, respectively, calculated as 0.36, 0.66, 0.68, 0.26, 0.18, and 0.58 mmol/g. It exhibits that the existing sulfonamide concentration on the surface is lower than the amine concentration in the parent substrate except that SNC3 in which the silanol groups of silica surface maybe reacted with the sulfonyl chlorides.
3.4 Scanning electron microscopy image and EDX
Scanning electron microscopy (SEM) images (Fig. 7) of FSiPrNH2 and SNA1 demonstrate nanosized particles in the range of 29–45 nm. Because the particle size was not changed after sulfonation of FSiPrNH2, therefore, the silica particles were not agglomerated after modification. Same results were obtained from the SEM images of the other modified FSi compounds. The EDX results of FSi, FSiPrNH2, and SNA1 are presented in Fig. 8, confirming the expected elements in each sample.

SEM images of (Left) FSiPrNH2 and (Right) SNA1.
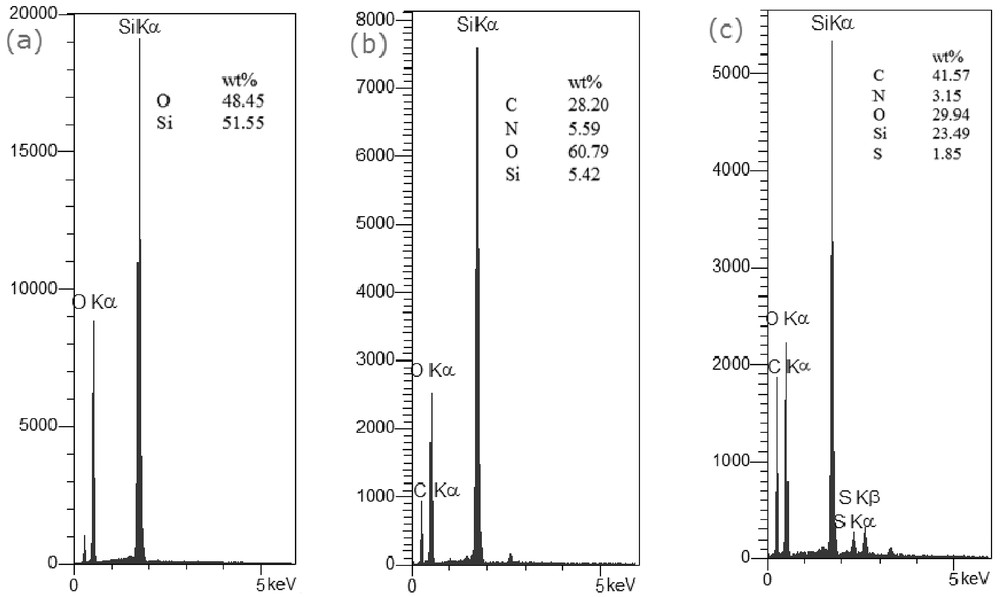
EDX analyses of (a) FSi, (b) FSiPrNH2, and (c) SNA1.
3.5 Inhibitory effect of sulfonamide-modified FSi against E. coli and S. aureus
MIC results of the modified FSi and three free sulfonamides (sulfaquinoxaline, sulfadiazine, and sulfadimidine), as models, against the Gram-negative bacteria, including E. coli, Pseudomonas aeruginosa, and Salmonella typhi, and Gram-positive bacteria, including S. aureus, Bacillus subtilis, and Enterococcus faecalis, are shown in Table 2. According to the results, FSiPrNH2 (SNA), SNA1, SNA2, and SNC3 show the minor effect against S. aureus as same as free sulfadiazine, whereas FSiPrPi (SNB), SNB1, and FSiPrPhDA (SNC) are more effective compounds against S. aureus. The antibacterial effect of SNB and SNC is probably because of the antibacterial properties of primary amines [21]. In addition, the obtained results from the antibacterial effect of SNA3, SNB2, SNB3, SNC1, and SNC2 against S. aureus are acceptable. S. aureus is commonly found in the respiratory tract, nose, and on the skin. It often causes skin and bloodstream infections in human and animal [22]. The modified FSi compounds showed minor effects against the other tested bacteria. It is essential to say that the effect of FSi compounds will increase by raising their concentration because the molarity of amine and grafted sulfonamides on the surfaces are not comparable with the concentration of free sulfonamides.
The MIC of nanostructures against bacteria (μg/mL).
Compound | E. coli | S. aureus | B. subtilis | P. aeruginosa | E. faecalis | S. typhi |
SNA | >1024 | 128 | >1024 | >1024 | >1024 | >1024 |
SNA1 | >1024 | 128 | >1024 | >1024 | >1024 | >1024 |
SNA2 | >1024 | 128 | >1024 | >1024 | >1024 | >1024 |
SNA3 | >1024 | 64 | >1024 | >1024 | >1024 | >1024 |
SNB | >1024 | 32 | >1024 | >1024 | >1024 | >1024 |
SNB1 | >1024 | 32 | >1024 | >1024 | >1024 | >1024 |
SNB2 | >1024 | 64 | >1024 | >1024 | >1024 | >1024 |
SNB3 | >1024 | 64 | >1024 | >1024 | >1024 | >1024 |
SNC | >1024 | 32 | >1024 | >1024 | >1024 | >1024 |
SNC1 | >1024 | 64 | >1024 | >1024 | >1024 | >1024 |
SNC2 | >1024 | 64 | >1024 | >1024 | >1024 | >1024 |
SNC3 | >1024 | 128 | >1024 | >1024 | >1024 | >1024 |
Sulfaquinoxaline | >1024 | 512 | 8 | >1024 | 16 | 256 |
Sulfadiazine | 512 | 128 | 16 | 512 | 64 | 32 |
Sulfadimidine | 512 | 512 | 32 | >1024 | 64 | 128 |
The first proposed mechanism for antibacterial properties of these nanostructures is based on the interaction between positively charged amine moieties of sulfonamides and negatively charged microbial cell membranes leading to the leakage of proteinaceous and other intracellular constituents. A second proposed mechanism is based on the binding of nanostructures with microbial ribosomes, which clearly requires entry of the nanostructures into the cell, and subsequently interferes with the messenger RNA and protein synthesis [23]. Accordingly, the zeta potential of SNA1 was obtained as +7.06 mV, which is related to the positively charged amines on the sulfonamide-modified surface and thus the first proposed mechanism is acceptable.
3.6 Application of the sulfonamide-modified FSi
Sulfonamide-based compounds are valuable materials for using in different fields of research. One of the most interesting reactions is Michael addition, which is performed in the presence of sulfonamides as a catalyst for the synthesis of nitroolefins [24]. Rani and Peddinti [25] used camphor-10-sulfonamide–based prolinamide as an organocatalyst for the Aldol reaction. In addition, sulfonamides are good ligands for chelating with metallic ions, for example, Han et al. synthesized varied l-leucine–derived chiral sulfonamide ligands and combined it with Ti(OiPr)4 to catalyze the addition of phenylacetylene to aldehydes [26]. Furthermore, asymmetric Mannich reaction of aldehydes and the R-iminoester catalyzed by the chiral amino sulfonamide were reported by Kano et al. [27]. Sulfonamide-based palladium complexes were also used for Suzuki, Heck, and Sonogashira coupling reactions [28]. Therefore, sulfonamide-modified FSi compounds can be used as catalysts and will be more efficient than those reported sulfonamide catalysts because of its heterogeneous nature.
On the other hand, so far, some sulfonamide-modified supports were used as Cu2+ detector [29], dye adsorbent [30], pH sensor [31], and drug delivery systems [32]. This shows the widespread use of such substrates. In addition to the mentioned plausible uses of sulfonamide-modified FSi compounds, these are useful compounds as additives for industrial silicone-based compounds (e.g., silicone glue and oil) because of their efficient antibacterial activity.
4 Conclusion
In this study, the surface of FSi was functionalized with different amine compounds, followed by treating with sulfonyl chlorides, and consequently, nine types of sulfonamide-modified FSi compounds were obtained. FT-IR spectra confirmed the presence of organic moieties on the surface of modified FSi materials. TGA and DTA curves demonstrated the amount of organic groups on the FSi surface, which was in the range between 0.18 and 2.07 mmol/g. SEM images exhibited the particle size of modified FSi as 29–45 nm. In addition, EDX analysis confirmed the presence of expected elements in the modified FSi compounds. Afterward, antibacterial tests using MIC protocol showed that SNB, SNB1, and SNC are the more effective antibiotics against S. aureus. Consequently, this research provided new silica-based antibiotics, which are applicable in catalysis and also producing antibacterial silicone-based compounds (e.g., silicone glue and oil) as well.
Acknowledgments
The authors are thankful for the financial support from the Research Council of Alzahra University and the University of Tehran.