1 Introduction
Nowadays, the production of chemicals avoiding waste through nonpolluting processes is still a challenge for researchers [1]. Unlike the pharmaceutical sector, involving low quantity chemical fabrication, the chemical industry, widely based on selective oxidation processes, implies large-scale production of chemicals; for example, 25 Mtones of terephthalic acid (TPA) is produced yearly from oxidation of p-xylene (PX) [2]. This process has a major industrial impact because TPA is the raw material for production of polyethylene terephthalate (starting material for bottles and textiles), polyester fibers, paint, and adhesives [3]. The commercial route implies the use of the acetic acid at 200 °C and 15–30 atm in the presence of the homogeneous catalytic system Co/Mn and Br as promoter. The process, known as AMOCO process, achieves 98% conversion of PX and 95 mol % yield of TPA, which is highly corrosive and polluting for the environment [4–9]. In addition, the crude TPA obtained at the end of reaction contains 3000 ppm 4-carboxybenzaldehyde (4-CBA) and 500 ppm p-toluic acid (TA) as major impurities [10].
Heterogeneous catalytic liquid phase oxidation of PX revealed the first encouraging results in 2001 [11] by Chavan et al. with a hybrid material μ3-oxo-bridged Co/Mn cluster complexes encapsulated in Y-zeolites. They have obtained a 99% selectivity of TPA for 100% conversion in only 2 h. Consequently, other heterogeneous systems were developed for PX oxidation, such as manganese oxide modified with Co or Cu [3] or Pd/Sb/MoTiO2 catalytic system [12]. Unfortunately, they suffer a few drawbacks deriving from the use of NaBr as coactivator, acetic acid as solvent, and harsh reaction conditions (high temperatures or pressures). Several studies have focused on the green aspect of the reaction and tried to develop systems that work in mild conditions even if this involves obtaining intermediate oxidation products, such as TA. Usually, AuPd alloy nanoparticles on different supports are able to oxidize the o-, p- and m-xylene to tolualdehyde, toluyl alcohol, and toluic acid in mild conditions [13–17]. Recently, different phases of CeO2 nanoparticles demonstrate their ability to oxidize with moderate yield the PX to TA (25%–35%) with the advantage of using very friendly reaction conditions [18,19]. Nevertheless, the data obtained in heterogeneous catalysis are not outstanding and further studies are needed.
This study addresses the problem of corrosive selective oxidation, by substituting the homogeneous system with a heterogeneous system containing mixed oxides of Mn and Fe, and replacing the harsh reaction conditions with more gentle ones. The advantages of this system are the ease in the separation of the catalyst and the reaction products, by filtration and the low waste, eco-friendly reaction conditions. This way the catalyst can be effectively recovered and reused. Manganese oxides are able to promote several oxidation reactions [20,21]. Our recent studies on Mn/Fe oxides emphasize their capabilities to convert the aromatic hydrocarbons in aromatic carboxylic acids [22–24]. Moreover, to achieve materials with specific characteristics, the preparation method should be carefully chosen; therefore, during this work the influence of the preparation method on the properties of the heterogeneous catalysts of Mn/Fe/O was investigated. For this purpose, the materials were prepared by hydrothermal treatment and citrate methods, characterized by several techniques and tested in the oxidation of PX using hydrogen peroxide, molecular oxygen, and tert-butyl hydroperoxide (TBHP) as oxidation agents.
2 Experimental section
2.1 Material synthesis
All reagents have analytical purity: Mn(NO3)2·6H2O (EMSURE, for analysis), Fe(NO3)3·9H2O (Scharlau, extra pure), polyethylene glycol (PEG, Sigma Aldrich), C6H8O7 (Sigma Aldrich).
2.1.1 Synthesis of MnFe mixed oxide by hydrothermal treatment
To prepare MnFe mixed oxide (molar ratio Mn/Fe = 1:2), stoichiometric molar amounts of manganese nitrate (2.5 g) [Mn(NO3)2·6H2O] and iron nitrate [Fe(NO3)3·9H2O] (7 g) were dissolved in 10 mL of water to form a clear solution. While stirring, 10 mL of PEG (a nonionic, polar polymer) was added to the solution to serve as a surfactant to avoid particle agglomeration. The pH was adjusted to 11 by adding 2 M of NaOH dropwise. The mixture was stirred for 1 h to obtain a homogeneous solution, then transferred into an autoclave and kept at 150 °C for 12 h, under autogenous pressure. The product was separated by filtration and washed several times with water, absolute ethanol, and acetone, until pH = 7. The resulted powder was dried at 70 °C overnight and calcined in air at 700 °C for 5 h. This material was denoted as Mn/Fe/O_HT.
2.1.2 Synthesis of MnFe mixed oxide by citrate method
MnFe mixed oxide with the same molar ratio (Mn/Fe = 1:2) was synthesized by complexation with citric acid. Stoichiometric amounts of manganese nitrate (2.5 g) [Mn(NO3)2·6H2O] and iron nitrate [Fe(NO3)3·9H2O] (7 g) were dissolved in 50 mL of deionized water at 60 °C to form a clear solution. Citric acid was added to this mixture with 20% excess. The solution was stirred for 1 h at 60 °C and then evaporated under vacuum until a gel was formed. The gel was dried under vacuum for 5 h at 60 °C and overnight at 120 °C in air. The obtained powders were calcined in air at 700 °C for 5 h. The material prepared by this method was denoted as Mn/Fe/O_CIT.
2.2 Textural and structural characterization
Dried synthesized materials were characterized using differential thermal analysis (DTA) and thermogravimetric (TG) analysis using a TG-DTA analyzer Shimadzu DTA-60 instrument. The experiments were performed in air in a temperature range of room temperature to 900 °C with a heating rate of 10 °C/min, using alumina as reference and 5–10 mg of a sample.
Powder X-ray diffraction (XRD) patterns were recorded using a Shimadzu XRD-7000 diffractometer with Cu K⍺ radiation (λ = 1.5418 Å, 40 kV, 40 mA) at a scanning speed of 0.10° min−1, in the 10°–80° 2θ range. XRD served to estimate the crystallite size of catalyst particles. This was calculated using Scherrer's formula (D = kλ/β cos θ, where D is the average crystallite size, k a constant equal to 0.89, λ the wavelength of X-rays, and β is the corrected half width).
The textural characterization of the calcined samples was performed by N2 adsorption at −196 °C. The surface area was calculated using the Brunauer–Emmett–Teller (BET) method from the data obtained at p/p0 between 0.01 and 0.35. Before the determination of the surface area, all powders were degassed at 150 °C for 5 h. The pore size distribution of each sample was determined from the desorption branch of the N2 isotherm using Barrett–Joyner–Halenda's (BJH) analysis, using a Micrometrics instrument (ASAP 2010).
2.3 Catalytic tests
The catalytic reactions were performed in a batch reactor, under thermal treatment while stirring, using a reflux system. In a typical liquid phase oxidation reaction, 50 mg of catalyst, 2 mL of acetonitrile, and 2.5 mmol of substrate (PX) were used. Different parameters were monitored to determine the best formulation of the catalyst: the influence of an oxidizing agent (OA; hydrogen peroxide and TBHP), the molar ratio between the substrate (S) and the OA, S/OA = 1:4, 1:8, 1:12, and the reaction temperature (60, 80, and 100 °C).
A series of catalytic tests with molecular oxygen were performed in a stainless steel autoclave (HEL Instruments) with 2.5 mmol of substrate (PX), 2 mL of acetonitrile, 0.05 g of catalyst, 20 bars of O2, 100 °C, and different molar ratios of S/OA (1:4, 1:8, 1:12).
After the reaction, the catalyst and the reaction mixture were separated by filtration and the reaction mixture was thermally treated for further analysis. The organic phase was extracted three times with dichloromethane before being analyzed by gas chromatography [(GC-2010) Shimadzu apparatus equipped with a DB-5MS capillary column (0.20 mm × 50 m × 0.33 μm) and flame ionization detector]. The remaining aqueous phase was precipitated with HCl to recover the possible acid compounds solubilized in this phase.
3 Results and discussion
3.1 Materials characterization
3.1.1 Thermogravimetric analysis–DTA
Fig. 1 represents TG-DTA profiles of the dried samples. The studied samples show different overall weight losses (TG curves) as a function of temperature. For Mn/Fe/O_HT sample, we observe a total weight loss of 10 wt % upon heating in one step, while for Mn/Fe/O_CIT sample a total weight loss of 70 wt % in two steps was recorded upon heating. Both materials, irrespective of the preparation method, are thermally stable over 400 °C. Therefore, the proper annealing temperature must be higher than 400 °C.
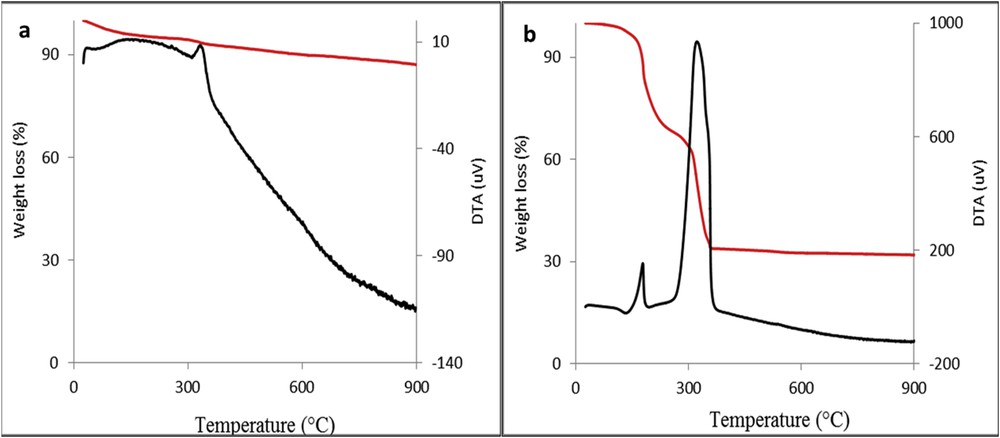
TG-DTA curves of the dried Mn/Fe/O_HT (a) and Mn/Fe/O_CIT (b) materials.
The weight loss for Mn/Fe/O_HT is accompanied by two enthalpic changes as a function of temperature: an endothermic effect at 100 °C associated with the elimination of species such as adsorbed water, surface OH groups and nitrate compounds, and an exothermic effect around 320 °C attributed to the surfactant decomposition (Fig. 1a). Indeed, as literature revealed the PEG decomposes thermally around 350 °C [25]. After this temperature no other changes were recorded on the DTA profile.
Concerning the DTA curve of the Mn/Fe/O_CIT, it presents two exothermic effects, associated with the two steps of the weight loss from TG curve: one around 170 °C because of the decomposition of pure citric acid that was used in excess (almost 20% weight loss) and the other one around 320 °C corresponding to the decomposition of citrate complex (Fig. 1b), revealing a weight loss of approximately 50%. Our study is consistent with the work of Prasad et al. [26] on the thermal decomposition of the citrate precursor for cobalt ferrite. It is also interesting to note that for this sample there is no weight loss associated with the adsorbed water and nitrate compounds at 100 °C as observed for Mn/Fe/O_HT. This is probably because the sample was dried at 120 °C overnight.
3.1.2 X-ray diffraction
The XRD patterns confirmed the presence of bixbyite-Mn2O3 phase (PDF card 00-041-1442), hematite (Fe2O3) (PDF card 00-033-0664), and of a mixed manganese iron oxide (Mn0.9Fe0.1)2O3 (PDF card 04-007-1252) in both materials Mn/Fe/O_CIT and Mn/Fe/O_HT (Fig. 2). Comparing the XRD patterns of the two calcined materials, it can be observed that in the case of Mn/Fe/O_HT the diffraction intensity lines from Fe2O3 became stronger than the ones obtained with Mn/Fe/O_CIT, indicating a higher degree of crystallinity. This may be because the hydrothermal treatment is a crystal growth method in hot water, which could favor the formation of crystalline phases during preparation. Also, from XRD patterns different crystallite sizes were observed for the two materials. The more crystalline phase leads to the formation of larger crystallite, of about 51 nm in the case of Mn/Fe/O_HT as compared with the one formed during the citrate method, of about 33 nm for the Mn/Fe/O_CIT material (Table 1).
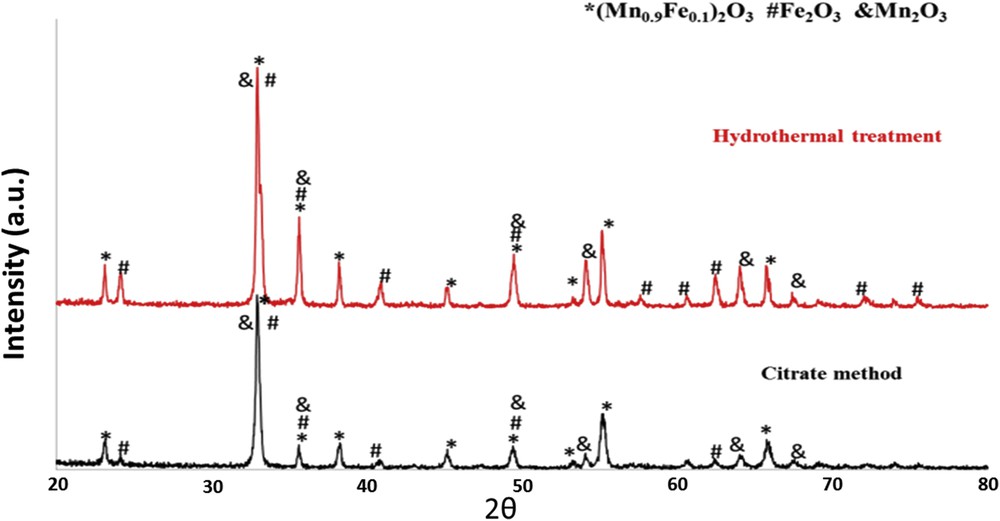
XRD patterns for Mn/Fe/O_CIT and Mn/Fe/O_HT.
BET surface area and pore volume of the Mn/Fe/O mixed oxides.
Material | Surface area (m2/g) | BJH pore volume (cm³/g) | Crystallite size (nm) |
Mn/Fe/O_CIT | 14 | 0.106 | 34 |
Mn/Fe/O_HT | 14 | 0.089 | 51 |
3.1.3 Adsorption–desorption isotherms
Textural properties of the studied materials were determined by adsorption–desorption isotherms, recorded at liquid nitrogen temperature (−196 °C), using BET formalism [27] and are presented in Fig. 3. Surface area, pore volume, and crystallite size of all samples are summarized in Table 1.

Adsorbtion–desorbtion isotherms for Mn/Fe/O_HT (left) and Mn/Fe/O_CIT (right).
The samples present type III isotherms, characteristic for weak adsorbate–adsorbent interactions, commonly associated with nonporous materials. This is explained by the sintering effect, which can appear at high calcination temperatures and cause the damage of the mesoporous structure of the material.
From Table 1, it can be observed that the surface area of the Mn/Fe/O materials is the same, although we expected different values, as the preparation method was different. In fact, the idea of using PEG was to prevent the particle agglomeration and to avoid sinterization during the thermal treatment of the Mn/Fe/O_HT sample. It seems that the calcination step at 700 °C leads to similar textural properties, irrespective of the preparation method.
3.2 Catalytic tests
3.2.1 Influence of the OA
The main reaction product from PX oxidation obtained during this study is TA that coexists with small amounts of p-methylbenzyl alcohol (4-MetOH), p-tolualdehyde (p-TALD), cresol, or 4-CBA.
We studied also the influence of the OA during the selective oxidation reaction of PX catalyzed by Mn/Fe/O materials. With this aim, we used molecular O2, hydrogen peroxide (H2O2), and TBHP as OAs. Results are depicted in Table 2.
Influence of the OA on the catalytic activity.
Entry | Catalyst | OA | C (%) | S (%) | Yield (%) TA | |||
4-MetOH | p-TALD | TA | Others | |||||
1 | Mn/Fe/O_CIT | O2 | 0 | – | – | – | – | – |
2 | H2O2 | 2 | 42 | 58 | – | 0 | 0 | |
3 | TBHP | 85 | 4 | 9 | 67 | 20 | 57 | |
4 | Mn/Fe/O_HT | O2 | 4 | – | – | – | – | – |
5 | H2O2 | 3 | 43 | 50 | – | 7 | 2 | |
6 | TBHP | 77 | 3 | 15 | 74 | 8 | 57 |
The use of different OAs influences the behavior of Mn/Fe/O catalysts during catalytic selective oxidation of PX. Irrespective of the method used for the preparation of Mn/Fe/O materials, the best OA remains the TBHP as compared with the hydrogen peroxide or molecular oxygen. Good conversion (up to 85%) and a preferential selectivity toward TA have been obtained with TBHP (Table 1, entry 3), in the detriment of p-MetOH and p-TALD (see Fig. 4). Low conversion was observed when H2O2 or molecular oxygen was used for both catalysts (Table 2, entries 1 and 2). The use of H2O2 in the oxidation of PX resulted in products with less oxidative states (4-MetOH and p-TALD).

Reaction products for the selective catalytic oxidation of PX.
The high reactivity of Mn/Fe/O catalysts in the presence of TBHP may result from the contribution of three features: (1) TBHP forms radical species more stable than the H2O2 homologues, (2) TBHP is more stable than H2O2, therefore, H2O2 decomposition occurs faster, and (3) hydrogen peroxide is inefficiently used in the oxidation of PX in the presence of acetonitrile. The fast reaction of H2O2 with the acetonitrile is known as the Radziszewski reaction [28].
Lack of reactivity in the presence of molecular oxygen can be explained by the lowest oxidizing character in comparison to the other two peroxides. Also, O2 is a very stable molecule according to the energetic diagram, having a bond order equal to 2, whereas the oxygen from peroxides, O22−, presents a lower bond order (1), which means that the bond is easier to break as compared with O2, reflecting a higher reactivity [29].
Mn/Fe/O synthesized by the citrate method exhibits a better catalytic activity as compared with the one obtained by hydrothermal treatment, under the same reaction conditions (Table 2, entries 3 and 6).
3.2.2 Influence of reaction temperature
The influence of temperature on the selective oxidation of PX with Mn/Fe mixed oxides was studied performing the reaction at three different temperatures: 60, 80, and 100 °C. The experimental outcomes are depicted in Table 3.
Influence of the reaction temperature on the catalytic activity.
Entry | Catalyst | Temperature (°C) | C (%) | S (%) | Yield (%) TA | |||
4-MetOH | p-TALD | TA | Others | |||||
1 | Mn/Fe/O_CIT | 60 | 57 | 2 | 31 | 55 | 12 | 31 |
2 | 80 | 85 | 1 | 9 | 82 | 8 | 72 | |
3 | 100 | 85 | 4 | 9 | 67 | 20 | 57 | |
4 | Mn/Fe/O_HT | 60 | 54 | 2 | 35 | 50 | 13 | 27 |
5 | 80 | 68 | 2 | 22 | 67 | 9 | 45 | |
6 | 100 | 77 | 3 | 15 | 74 | 8 | 57 |
Increasing the reaction temperature, an increase in the conversion yield occurs, as expected; however, the two mixed oxides behave differently. In the case of Mn/Fe/O_CIT, increasing the temperature from 60 to 80 °C resulted in an increase in the conversion with 28%, whereas a further increase from 80 to 100 °C produces no change in the catalytic activity (Table 3, entry 1–3). The highest yield in TA was achieved at 80 °C (72%) (Table 3, entry 2). The increase in temperature to 100 °C caused a decrease in approximately 15% in yield of TA because a higher temperature leads to further oxidation of TA to 4-CBA detected among “other” compounds. Concerning the Mn/Fe/O_HT catalyst, the activity is lower as compared to its homologue prepared by the citrate method but also presents an increase in the conversion along with the increase in temperature (Table 3, entries 4–6). The selectivity of TA follows the same trend as the conversion increases by increasing the temperature, but in this case no advanced oxidation of TA was observed. This behavior demonstrates that Mn/Fe/O_HT does not possess enough accessible sites that can favor the advanced oxidation reaction.
Summarizing, as already mentioned previously, the Mn/Fe/O synthesized by the citrate method exhibits a better catalytic activity as compared with the one obtained by hydrothermal treatment, under the same reaction conditions (Table 3). Such a behavior can be explained from the structure of the catalytic materials. From XRD patterns (Fig. 2) of the Mn/Fe/O_CIT, the Fe2O3 phase has a lower crystallinity degree and presents smaller dimension particle size as compared with Mn/Fe/O_HT, which can be assimilated with a higher amount of active sites. Also, different contributions of the three compounds that constitute the catalyst, Mn oxide, Fe oxide, and Mn/Fe oxide, could play an important role. All these lead to an enhancement of the catalytic activity of the Mn/Fe mixed oxide prepared by the citrate method. However, the yield in TA is similar for both catalysts (Table 3, entries 3 and 6), demonstrating that the composition of the catalysts (identical for both preparation methods) is related to the product selectivity, whereas the number of active sites is responsible for the activity of the catalysts and can be tuned by the preparation method.
3.2.3 Influence of molar ratio between the substrate and OA
Going further in our study, we investigated the influence of a molar ratio between the substrate and OA on the catalytic activity. For this purpose, we used tert-butyl hydroperoxide as an OA, because it proved to be the most efficient. Different molar ratios as 1:4, 1:8, and 1:12 for PX to tert-butyl hydroperoxide were used. Results are presented in Table 4.
Influence of the molar ratio between the substrate and OA on the catalytic activity.
Entry | Catalyst | Molar ratio PX/TBHP | C (%) | S (%) | Yield (%) TA | |||
4-MetOH | p-TALD | TA | Others | |||||
1 | Mn/Fe/O_CIT | 1:4 | 98 | – | 2 | 95 | 3 | 93 |
2 | 1:8 | 94 | 1 | 9 | 96 | 2 | 90 | |
3 | 1:12 | 85 | 4 | 9 | 67 | 20 | 57 | |
4 | 1:4a | 70 | 6 | 12 | 81 | 1 | 57 | |
5 | Mn/Fe/O_HT | 1:4 | 93 | – | – | 99 | – | 92 |
6 | 1:8 | 94 | 1 | 2 | 96 | 2 | 90 | |
7 | 1:12 | 77 | 3 | 15 | 74 | 8 | 57 | |
8 | 1:4a | 54 | 5 | 20 | 73 | 2 | 40 |
a TBA (tert-butyl alcohol) was added to the mixture; molar ratio PX/TBPH/TBA 1:4:8.
As a general trend, a decrease in the conversion by increasing the amount of an OA is observed. During the oxidation process, side products are generated in the reaction media, like TBA (Scheme 1 (1)) and water (Scheme 1 (2)). By increasing the amount of an OA in the reaction media, the amount of side products generated by TBHP (TBA and water) will increase, which can compete for the active sites of the catalyst and cause their blocking. Therefore, an optimal molar ratio PX to OA is 1:4, for which the conversion is 98%. However, a higher amount of TBHP in reaction media affords further oxidation of TA, but in the detriment of PX conversion (Table 4, entries 3 and 7).
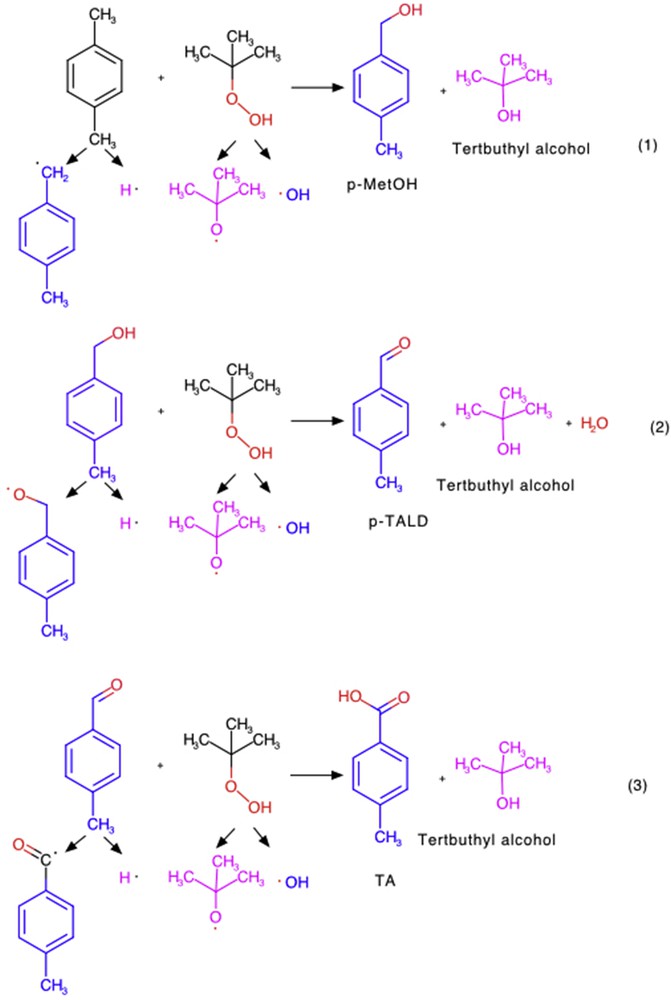
Possible pathway for the oxidation process of PX in the presence of the Mn/Fe/O.
Additional experiments were conducted to understand the role of TBA in the PX oxidation. In addition, TBA was added to the mixture in a molar ratio PX/TBPH/TBA 1:4:8 (Table 4, entries 4 and 8). A decrease in the conversion of PX and in TA selectivity can be observed by adding TBA, indicating that TBA can both compete with PX and products for the active sites or can act as a radical trapper.
At the best reaction conditions (PX/TBHP = 1:4, 100 °C, 24 h) the main product TA coexists with small amounts of p-MetOH, p-TALD, cresol, or 4-CBA. Scheme 1 presents the possible pathways for the oxidation process when Mn/Fe/O is used as a heterogeneous catalyst and TBHP as an OA: during the first step (reaction 1) the formation of p-MetOH occurs and also the formation of TBA as a side product takes place; going further (reaction 2) the alcohol is oxidized with the formation of aldehyde (TALD) accompanied by the side products (TBA and water). At the third step (reaction 3) further oxidation of the aldehyde to the corresponding carboxylic acid, TA, occurs together with TBA as side products.
3.3 Recyclability tests
The catalyst stability and recyclability were established by performing two successive reaction tests. The oxidation of PX in the presence of Mn/Fe/O_CIT was selected as our benchmark reaction. After the reaction, the catalyst was separated via centrifugation, washed with water, and dried at 60 °C for 2 h. The recycled supported catalysts exhibit a loss of activity of only 13% after the first cycle. Only a slight decrease in the TA yield was observed after two runs (Table 5).
Influence of the catalyst stability and recyclability on the catalytic activity.
Run | C (%) | S (%) | Yield (%) TA | |||
4-MetOH | p-TALD | TA | Others | |||
1 | 98 | – | 2 | 95 | 3 | 93 |
2 | 85 | – | 7 | 94 | 2 | 80 |
4 Conclusions
In this study, PX was successfully oxidized to obtain p-toluic acid in the presence of Mn/Fe/O prepared by two different methods, citrate method and hydrothermal treatment. Several OAs were used and TBHP favored a high conversion and yield in TA, owing to its stability and reactivity, as compared with hydrogen peroxide or molecular oxygen. The Mn/Fe/O prepared by the citrate method show better catalytic performance (98% conversion and 93% yield) because of smaller crystallite size formed during preparation with a lower degree of crystallinity, which can be responsible for the catalytic activity.
Acknowledgments
The authors acknowledge the Romanian Ministry of Research and Innovation (PN-II-PT-PCCA-2011-3.2-1683 and PN-III-16-48-03 core program) for financial support.