1 Introduction
Surfactants are the group of organic compounds that continue to attract great interest from researchers because of their wide range of applications as laundry detergents, emulsifiers, corrosion inhibitors, oil recovery and pharmaceuticals. These are the most representative chemical products to be consumed in major quantities daily and globally and have in the past led to adverse effects on the aquatic environment. Many studies have previously revealed the adverse impact of widespread use of conventional surface active agents on the environment [1]. As such nontoxicity, biodegradability and biocompatibility of surfactants have become almost equally important for the consumers as their functional performance.
Biosurfactants are a class of green and sustainable surface active agents naturally synthesized from microorganisms such as bacteria, fungi and yeast or excreted extracellularly. Synthetic equivalents to biosurfactants can therefore be prepared by designing molecules that imitate natural amphiphilic structures such as phospholipids, alkyl glucosides and acyl amino acids. Amino acid surfactants (AASs) are one such type of surfactant that can generally be originated from animal or agricultural-derived feedstocks. AASs have been gaining great interest of scientists over the last two decades as novel surfactants because they can be synthesized using renewable sources and their ease of degradability and harmless byproduct make them safer for our environment [2].
AASs can be defined as a group of surfactants made up of amino acids comprising amino acid group (HO2C-CHR-NH2) or its residue (HO2C-CHR-NH2). These two functional regions of amino acids give the possibility to derive an extensive range of surfactants. There are total of 20 standard proteinogenic amino acids known in nature, which are responsible for growth and all physiological reactions of living kingdom. They differ from each other only on the basis of the residue, R [3] (Fig. 1). Some are nonpolar and hydrophobic, others are polar and hydrophilic, some are basic and some are acidic. As amino acids are renewable compounds, surfactants synthesized from amino acids also have great potential as sustainable and eco-friendly substances [4]. Simple and natural structure, low toxicity and fast biodegradation often make them superior to their conventional counterparts. Their production can be via different biotechnological and chemical routes using renewable raw materials such as amino acids and vegetable oils.
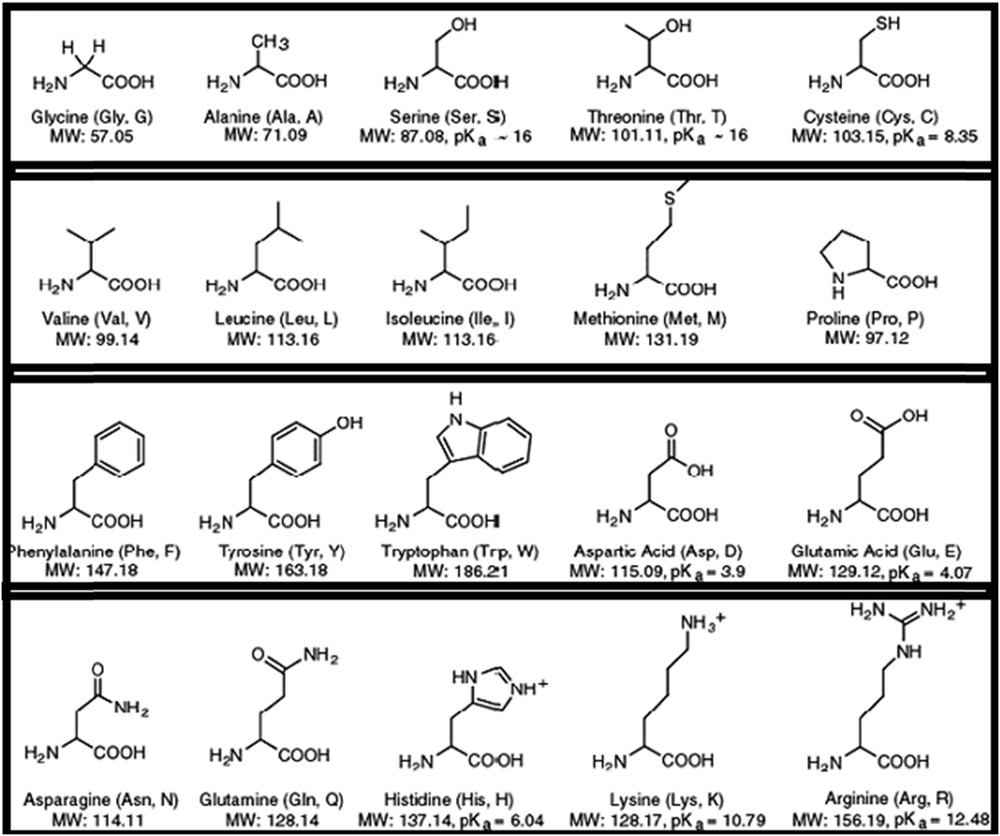
Twenty standard amino acids. pKa is the negative base-10 logarithm of the acid dissociation constant (Ka) of a solution. pKa = −log10 Ka.
Amino acids were first discovered as a substrate for surfactants in the early 20th century [5]. Primarily they were used as preservatives in pharmaceuticals and cosmetic formulations. Furthermore, they were found to be biologically active against a variety of disease-causing bacteria, tumors and viruses [6]. In 1988, availability of AASs at low cost increased the interest of researchers to study their surface activity [7]. Nowadays, along with the growth of biotechnology, a few amino acids are also able to be synthesized commercially at a large scale by yeasts, thus justifying their production to be more environmental friendly [8].
2 History
The value of the structures of naturally occurring amino acids as raw materials for preparing amphiphiles was predicted as soon as they were discovered early in the 19th century. The first research on AAS synthesis was reported in 1909 by Bondi [9]. In that study, N-acylglycine and N-acylalamine as hydrophilic moieties of surfactants have been introduced. Subsequent work involved the synthesis of lipoamino acid using glycine and alanine [10]. Hentrich et al. [11] published a series of findings and filed the first patent on the applications of acyl sarcosinate and acyl aspartate as surfactants in household cleaning products such as shampoos, detergents and toothpastes. Subsequently, the synthesis and physicochemical properties of acyl amino acids have been studied by many researchers [12,13,14]. To date a significant number of research publications have been published on the synthesis [12,13,15–19,20], properties [21–26], industrial applications [27–29] and biodegradability [17,30–33] of AASs.
3 Structural properties
Nonpolar hydrophobic fatty acid chains of AASs may vary in their structure, length and number. Structural variety and high surface activities of AASs explain their wide compositional diversity and broad range of physicochemical and biological properties. The difference in the amino acid or peptide head group of AASs determines the adsorption, aggregation and biological activity of these surfactants. Their types in terms of cationic, anionic, nonionic and amphoteric depend on the functional groups present in them. The combination of hydrophilic polar amino acids and nonpolar long-chain hydrophobic moiety for building up the amphiphilic structure has created molecules with high surface activity [4,34,35]. In addition asymmetric carbons present in the molecule help in the formation of chiral molecules [36,37].
4 Chemistry
All peptides and polypeptides are the polymerization products of 20 or so proteinogenic α-amino acids. All 20 α-amino acids comprise a carboxylic acid (–COOH) functional group and an amino (–NH2) functional group attached to the same tetrahedral α-carbon atom [5]. Amino acids differ from each other on the basis of distinct R-groups attached to the α-carbon (except for glycine where the R-group is hydrogen). R-groups may differ from each other in structure, size and electric charge (acidity/basicity). These differences also determine the solubility of amino acids in water.
The presence of four different substituents attached to α-carbon makes amino acids chiral in nature (except glycine) and hence optically active. Amino acids have two possible configurations; both are nonsuperimposable mirror images of each other, although in nature the l-stereoisomers are significantly more abundant. The presence of aromatic R-groups in some amino acids (phenylalanine, tyrosine and tryptophan) results in the absorption of ultraviolet light with an absorbance maximum at 280 nm [36]. Both, the acidic α-COOH and basic α-NH2 groups present in amino acids are able to ionize and create ionic equilibrium as follows:
Amino acids contain at least two weakly acidic groups; however, the carboxyl group is a far stronger acid than the amino group. At pH 7.4, the carboxyl group is unprotonated and the amino group is protonated. An amino acid with no ionizable R-group would be electrically neutral at this pH and forms zwitterions [38].
5 Classification
Four parameters are taken into consideration to classify AASs, these are sequentially addressed below.
5.1 On the basis of origin
On the basis of their origin, AASs can be categorized into two following classes.
5.1.1 Natural class
Some naturally occurring amino acid–containing compounds also possesses the ability to reduce surface and interfacial tension, some even surpassing the efficacy of glycolipids [39,40]. These types of AASs are also known as lipopeptides. Lipopeptides are low molecular weight compounds, which are generally produced by Bacillus species. This class of AASs is further classified into three subclasses: surfactin, iturin and fengycin (Fig. 2).
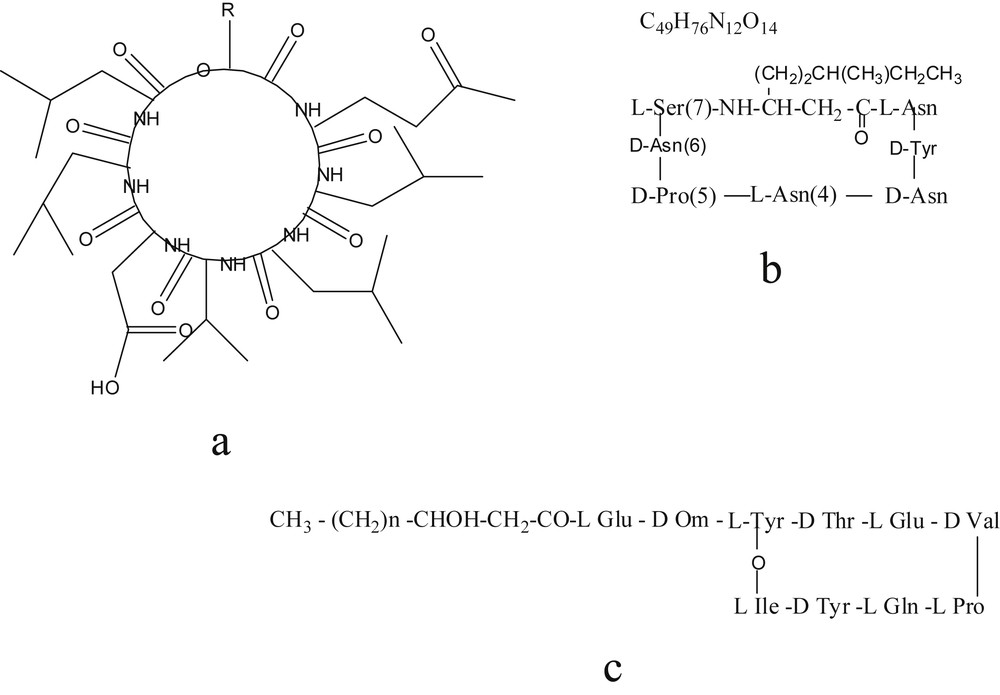
a) Surfactin; (b) iturin A3 and (c) fengycin B.
The surfactin [41,42] family covers the heptapeptide variants of the esperin, pumilacidin, lichenysin and surfactin groups. In such types of surfactants, the peptide moiety is linked to a C12–C16 unsaturated linear, iso or anteiso β-hydroxyl fatty acid chain. Surfactins are the macrolactone rings, in which ring closure is catalyzed in between the β-hydroxyl fatty acid and the C-terminal peptide.
In the iturin [43] subclass, there are mainly six variants, that is, iturin A and C, mycosubtilin and bacillomycin D, F and L. In all the cases, the heptapeptide is attached to a varied C14–C17 chain of β-amino fatty acids. In iturins, because of its β-amino nature, an amide bond is formed with the C-terminal group and thus forms a macrolactame structure.
The fengycins [44–46] subclass encompasses fengycins A and B, which are also known as plipastatins when Tyr9 is d-configured. These decapeptides are also linked to a C14–C18, saturated or unsaturated linear, iso or anteiso-β-hydroxyl fatty acid chain. Structurally, fengycins are also macrolactone rings that include a Tyr side chain at third position in the peptide sequence and form an ester bond with the C-terminal residue, thus forming an internal ring-like structure in many Pseudomonas lipopeptides.
5.1.2 Synthetic class
AASs can also be synthesized experimentally by use of any of the acidic, basic and neutral amino acids [47]. Common amino acids used for their synthesis are glutamic acid, serine, proline, aspartic acid, glucine, arginine, alanine, leucine and protein hydrolysates. Surfactants of this subclass can be prepared chemically, enzymatically and chemoenzymatically; however, chemical synthesis has been found more economically feasible for their production. Common examples of this include N-lauroyl-l-glutamic acid and N-palmitoyl-l-glutamic acid.
5.2 On the basis of the substitution of aliphatic chain
On the basis of the substitution of aliphatic chain, amino acid–based surfactants can be classified into two types [20].
5.2.1 On the basis of substitution site
In this type, there are three different categories as described in the following sections.
5.2.1.1 N-substituted AASs
In N-substituted compounds, an amino group is substituted either with a lipophilic moiety or a carboxylic group, resulting in the loss of alkalinity. The simplest examples of N-substituted AASs are N-acyl amino acids, which are anionic in nature. N-substituted AASs have an amide linkage formed in between the hydrophobic moiety and the hydrophilic moiety. The ability of amide linkage to form hydrogen bond eases the degradation of such surfactants under acidic environment and hence makes them biodegradable.
5.2.1.2 C-substituted AASs
In C-substituted compounds, substitution takes place on the carboxylic group through an amide or an ester bond. C-substituted compounds are typically cationic in nature such as esters or amides.
5.2.1.3 N- and C-substituted AASs
In the second type of surfactants, both the amino and the carboxylic groups are present as part of the hydrophilic moiety. These types are amphoteric in nature.
5.3 On the basis of the number of hydrophobic tail
On the basis of the number of hydrophobic tail and head groups, AASs are classified into four groups [5]. Single or linear chain AASs, dimeric or gemini AASs, glycerolipid-type AASs and bolaamphiphile AASs. Linear chain surfactants are the surfactants comprising amino acids with the minimum one hydrophobic tail (Fig. 3). Dimeric AASs have two amino acid polar head groups and two hydrophobic tails per molecule (Fig. 4). In this class, two linear AASs are joined together via spacer and hence also called dimerics. Glycerolipid-type AASs contain two hydrophobic tails attached to a one common amino acid head group (Fig. 5). These surfactants can be considered as an analogue of monoglycerides, diglycerides and phospholipids. Bolaamphiphile AASs are a group of surfactants that has two polar amino acid heads that are joined together via a single hydrophobic tail (Fig. 6).
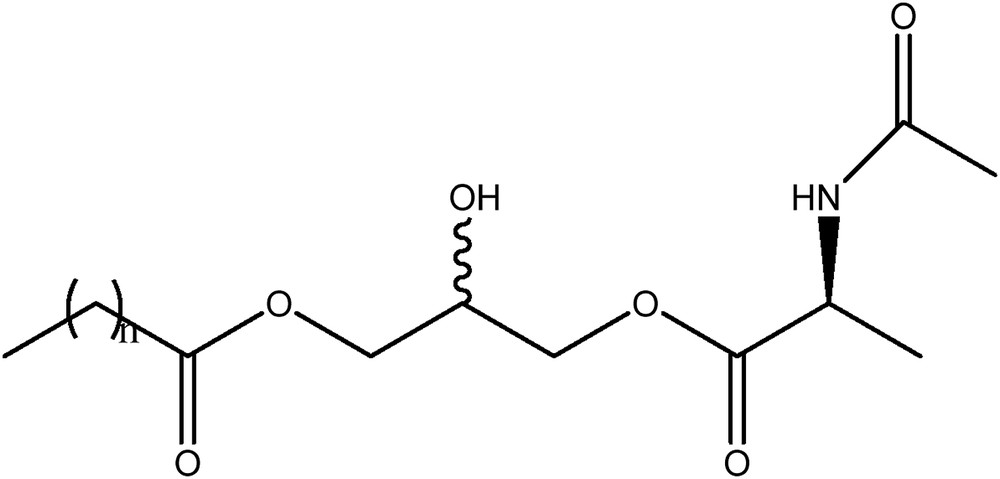
Example of linear AAS.
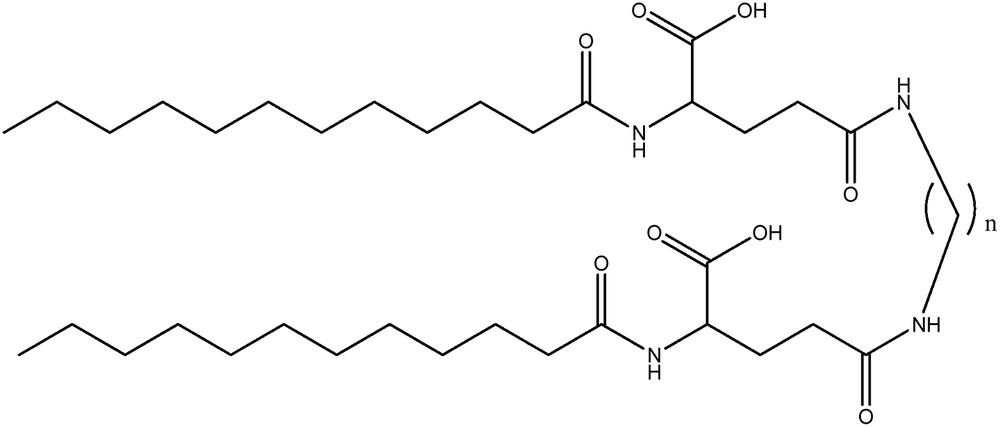
Example of gemini AAS (s = 2, 5 and 8).

Example of glycerolipid-type AAS.
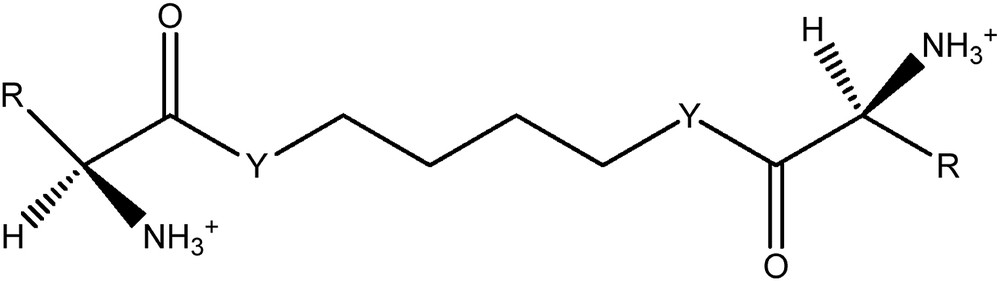
Example of bolaamphiphile AAS.
5.4 On the basis of head group type
On the basis of polar head group amino acid–based surfactants are further classified into cationic, anionic, zwitterionic and nonionic AASs.
5.4.1 Cationic AASs
In this class of surfactants, the head group bears positive charge. The first commercially available cationic AAS is cocoyl arginine ethyl ester as a pyrrolidone carboxylic acid salt. This surfactant has unique and varied properties that make it useful in disinfectants, antimicrobial agents, antistatic agents, hair conditioner and it is also very mild to eyes and skin and easily biodegradable [47]. Singare and Mhatre [48] have synthesized arginine-based cationic AASs and evaluated them on the basis of their physicochemical properties. In this study, they claimed the good yield of product using Schotten–Baumann reaction conditions. Increase in the surface activity and decrease in the critical micelle concentration (cmc) of surfactants were found with the increase in alkyl chain length and the hydrophobicity. Quaternary acyl protein is another known example of this class, which is generally used as a conditioner in hair care products [44].
5.4.2 Anionic AASs
In anionic surfactants, negative charge is present on the polar head group of surfactants. Sarcosinate surfactants are an example of anionic AASs. Sarcosine (CH3–NH–CH2–COOH, N-methyl glycine) is an amino acid normally found in sea urchins and starfish and is chemically related to glycine (NH2–CH2–COOH), a basic amino acid found in the cells of mammals. Lauric acid, myristic acid and oleic acid and their halides and esters are generally used to synthesize sarcosinate surfactants. Sarcosinates are mild in nature, due to which they are generally used in mouthwashes, shampoos, aerosol shaving lathers, sunscreens, skin cleansers and other cosmetics [49].
Other commercial anionic AASs include Amisoft CS-22 and Amilite GCK-12, which are the trade names of sodium N-cocoyl-l-glutamate and potassium N-cocoyl glycinate, respectively. Amilite is generally used as foaming agent, detergent, solubilizer, emulsifier and as a dispersing agent and has numerous applications in cosmetics as shampoo and bath soap, body wash, toothpaste, face wash, facial soap, contact lens cleaners and household tensides [50]. Amisoft is used as mild skin and hair cleansers and predominantly used in face and body cleansers, synthetic detergent bars, body care hair shampoos and other skin care products [51].
5.4.3 Zwitterionic (amphoteric) AASs
Amphoteric surfactants contain both acidic and basic sites and thus change charge by varying pH. They behave like anionics in alkaline medium, cationics in acidic environment and amphoterics in neutral medium. Lauroyl lysine (LL) and alkoxy (2-hydroxypropyl) arginine are the only known amino acid–based amphoteric surfactants. LL is a condensation product of lysine and lauric acid. Due to its amphoteric structure, LL is insoluble in almost all types of solvents except highly alkaline or acidic solvents. Excellent adhesive property towards hydrophilic surfaces and low friction coefficient of LL as organic powder imparts excellent lubricating ability in this surfactant. LL is widely used in skin creams and hair conditioners or as a lubricant [52].
5.4.4 Nonionic AASs
Nonionic surfactants are characterized by polar head groups that carry no formal charge. Al-Sabagh et al. [53] prepared eight novel ethoxylated nonionic surfactants using oil soluble α-amino acids. In this process, esterification of l-phenylalanine (LEP) and l-leucine followed by amidation with cetyl alcohol and palmitic acid, respectively, yielded two amides and two esters of α-amino acids. The ethylene oxide was then condensed with the amides and esters prepared and produced three different polyethylene oxide units of 40, 60 and 100 as phenylalanine derivatives. These nonionic-type AASs were found to have good detergency and foaming properties [53].
6 Synthesis
6.1 Fundamental synthesis paths
In AASs, the hydrophobic group may be attached at the amine moiety, the carboxylic acid moiety, or through the side chain of the amino acid. On the basis of this approach, four fundamental synthesis paths are available to researchers [54] as given in Fig. 7.
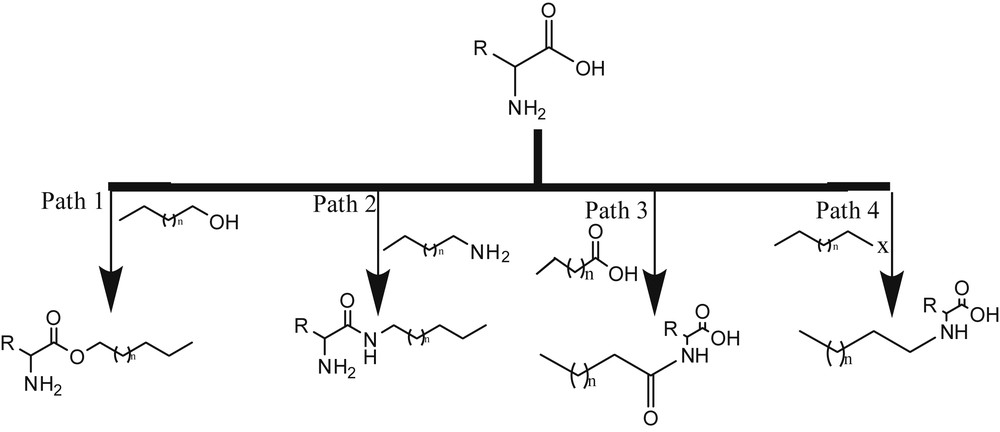
Fundamental synthesis paths of amino acid–based surfactants.
Path 1 shows the production of amphiphilic ester amine through esterification reactions, in which surfactant synthesis is typically achieved by refluxing fatty alcohols and amino acid in the presence of the dehydrating agent and acidic catalyst. In some reactions, sulfuric acid plays the role of both the catalyst and the dehydrating agent.
Path 2 shows the synthesis of amphiphilic amidoamine by creating the amide bond through the reaction of an activated amino acid and alkyl amine to create the desired amide bond.
Path 3 shows the production of amido acid through the reaction of the amine group of amino acid with fatty acid.
Path 4 shows the synthesis of long-chain alkyl amino acids via the reaction of the amine group with alkyl halogen.
Path 5 involves the coupling of the specific function of the side group of the amino acid. In this type of path, generally the carboxylic group of aspartic acid and glutamic acid reacts with fatty alcohol to form anhydride (Fig. 8).
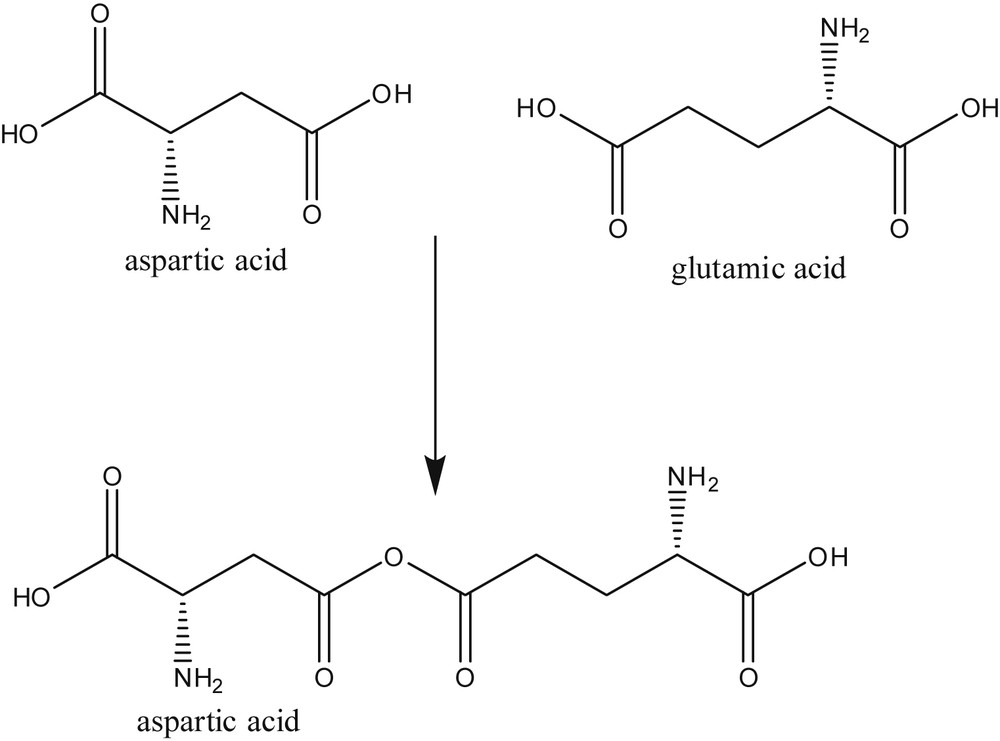
Path 5 for synthesis of AASs.
6.2 Development in the synthesis/production
6.2.1 Synthesis of single-chain amino acid/peptide surfactants
Synthesis of N-acyl and O-acyl amino acids [54] or peptides can be achieved by the enzyme-catalyzed acylation reaction of amine or alcohol groups with fatty acids. The first description of solvent-free lipase-catalyzed synthesis of amino acid amide or methyl ester derivatives was reported by using Candida antarctica with the yield in the range of 25–90% depending on the target amino acid [55]. In some reactions, ethyl methyl ketone has also been used as a solvent. Vonderhagen et al. [56] also illustrated the lipase and proteases catalyzed N-acylation of amino acids, protein hydrolysates and/or their derivatives using the mixture of water and organic solvents such as dimethylformamide/water and butyl methyl ketone. In these earlier examples, the major issue with enzymatic synthesis of AASs was typically very low yields. Valivety et al. [57] reported yields of just 2–10% of N-myristoyl amino acid derivatives even after many days under incubation at 70 °C by using different lipases. Problems associated with the low yields of amino acid were also supported by Montet et al. [58] during the synthesis of N-acyllysines using fatty acids and vegetable oils. They reported a maximum 19% yield of the product under both the solvent-free conditions and organic solvents. Valivety et al. [59] also supported the same issue while synthesizing N-Cbz-l-lysine or N-Cbz-lysine methyl ester derivatives. In this study, they claimed 80% yield of 3-O-myristoyl-l-serine using N-protected serine as a substrate and Novozyme 435 as a catalyst under a melted solvent-free environment. Nagao and Kito [60] studied the results of O-acylation of l-serine, l-homoserine, l-threonine and l-tyrosine (LET) using lipases obtained by Candida cylindracea and Rhizopus delemar in an aqueous-buffered medium and reported the acylation of l-homoserine and l-serine up to some extent with low yield, whereas no acylation takes place on l-threonine and LET. Various researchers supported the use of cheap and easily available substrate to synthesize cost effective AASs. Soo et al. [61] claimed the best results of palm oil–based surfactants using immobilized lipoenzyme. They stated that although reactions with palm oil fractions required long reaction duration of 6 days but resulted better yields of products. Gerova et al. [62] studied the synthesis and surface active properties of several optically active and racemic mixtures chiral of N-palmitoyl AASs based on methionine, proline, leucine, threonine, phenylalanine and phenylglycine. Pang and Chu [63] described the copolycondensation of amino acid–based monomers and dicarboxylic acid–based monomers to synthesize a series of biodegradable functional amino acid–based polyesteramides in solution.
Cantacuzene and Guerreiro reported the esterification of carboxylic acid group of Boc-Ala-OH and Boc-Asp-OH using long-chain aliphatic alcohols and diols using dichloromethane and Sepharose 4B as a solvent and a catalyst, respectively. In this study, good yields (51%) of Boc-Ala-OH were obtained with fatty alcohols up to 16 carbon atoms whereas six and 12 carbon atoms were found better and gave 63% of Boc-Asp-OH [64]. Clapes et al. [31] claimed 58–76% yield and 99.9% purity of N-arginine alkyl amide derivatives obtained through the formation of amide and ester bonds between Cbz-Arg-OMe and various long-chain alkyl amines and fatty alcohols in the presence of Papain from Carica papaya latex as a catalyst.
6.2.2 Synthesis of gemini amino acid/peptide surfactants
Amino acid–based gemini surfactants comprised two linear AAS molecules joined together head to head via a spacer [65]. Two possible schemes have been derived for the chemoenzymatic synthesis of gemini-type amino acid–based surfactants (Schemes 1 and 2). In Scheme 1, two amino acid derivatives react with the spacer followed by the introduction of the two hydrophobic groups. In Scheme 2, two linear structures are joined directly through a bifunctional spacer chain [66]. Valivety et al. [57] were the first to develop the enzymatic synthesis of gemini lipoamino acids. Yoshimura et al. [65] studied the synthesis, adsorption, and aggregation properties of an amino acid–based gemini surfactant based on cystine and n-alkyl bromide. Comparisons of the synthesized surfactants have also been performed against their corresponding monomeric counterparts. Faustino et al. [67] described the synthesis of anionic urea–based monomeric AASs and their corresponding geminis based on l-cystine, d-cystine and dl-cystine, as well as derived from l-cysteine, l-methionine and l-cysteic acid, and their characterization was achieved in terms of electrical conductivity, equilibrium surface tension and steady-state fluorescence spectroscopy techniques. Comparison studies between monomeric forms and geminis stated that geminis have lower cmc values.
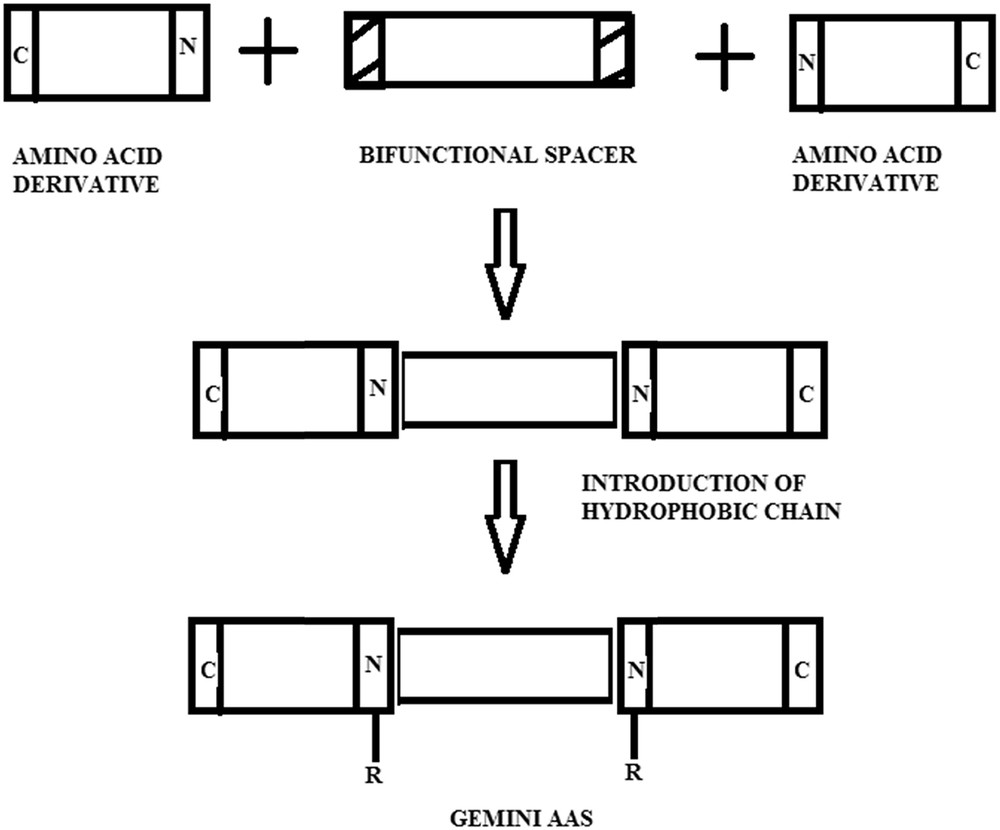
Synthesis of gemini AAS using AA derivatives and spacer, followed by insertion of the hydrophobic group.
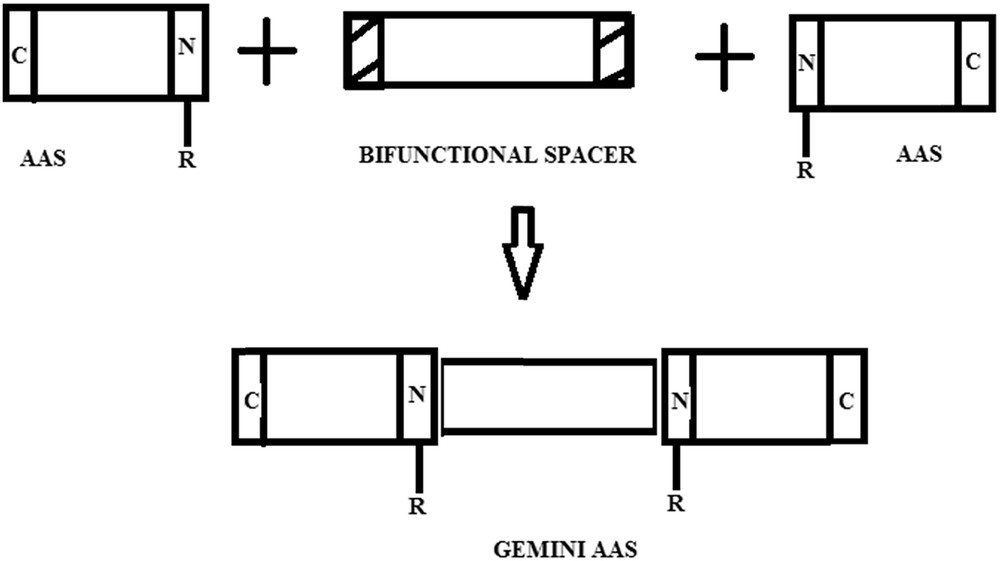
Synthesis of Gemini AASs using bifunctional spacer and AAS.
6.2.3 Synthesis of glycerolipid amino acid/peptide surfactants
Glycerolipid amino acid/peptide surfactants comprise a novel class of lipoamino acids, which are the structural analogues of mono and diacylglycerides and phospholipids as one or two aliphatic chains and one amino acid joined together via ester bonds in the glycerol backbone. Synthesis of such types of surfactants starts with the preparation of glyceryl esters of amino acid in the presence of an acid catalyst such as BF3 at elevated temperatures [57]. Enzymatic synthesis using hydrolases, proteases and lipases as catalysts was also found to be good alternative (Scheme 3) [68]. Enzymatic synthesis of dilauroylated arginine glyceride conjugate with papain enzyme has also been reported. Synthesis of diacylglycerolipid conjugates from acetylarginine and evaluation of their physicochemical properties have also been reported [69–71].
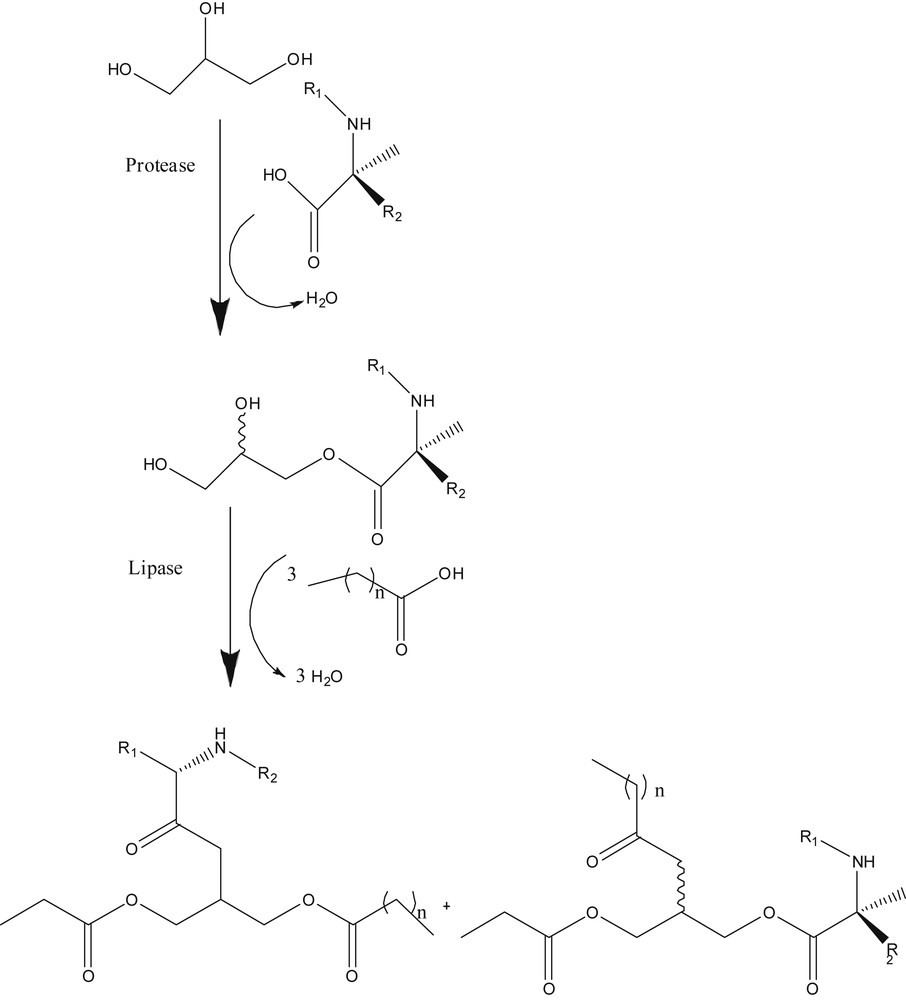
Synthesis of mono and diacylglycerol amino acid conjugates.
6.2.4 Synthesis of bolaamphiphile amino acid/peptide surfactants
Amino acid–based bolaamphiphiles contain two amino acids connected with a hydrophobic linker. Franceschi et al. [72] described the synthesis of bolaamphiphiles with two amino acids (d- or l-alanine or l-histidine) and an alkyl chain of varying length and studied their surface active properties. They discussed the synthesis and aggregation of novel bolaamphiphiles bearing amino acid moieties using unusual β-amino acids or an alcohol and spacers with C12–C20. The unusual β-amino acids used may be sugar amino acid, an azidothymin (AZT)-derived amino acid, a norbornene amino acid, and an AZT-derived amino alcohol (Scheme 4). Polidori et al. [73] has also described synthesis of symmetric bolaamphiphiles derived from tris(hydroxymethyl)aminomethane (Tris) (Scheme 4).
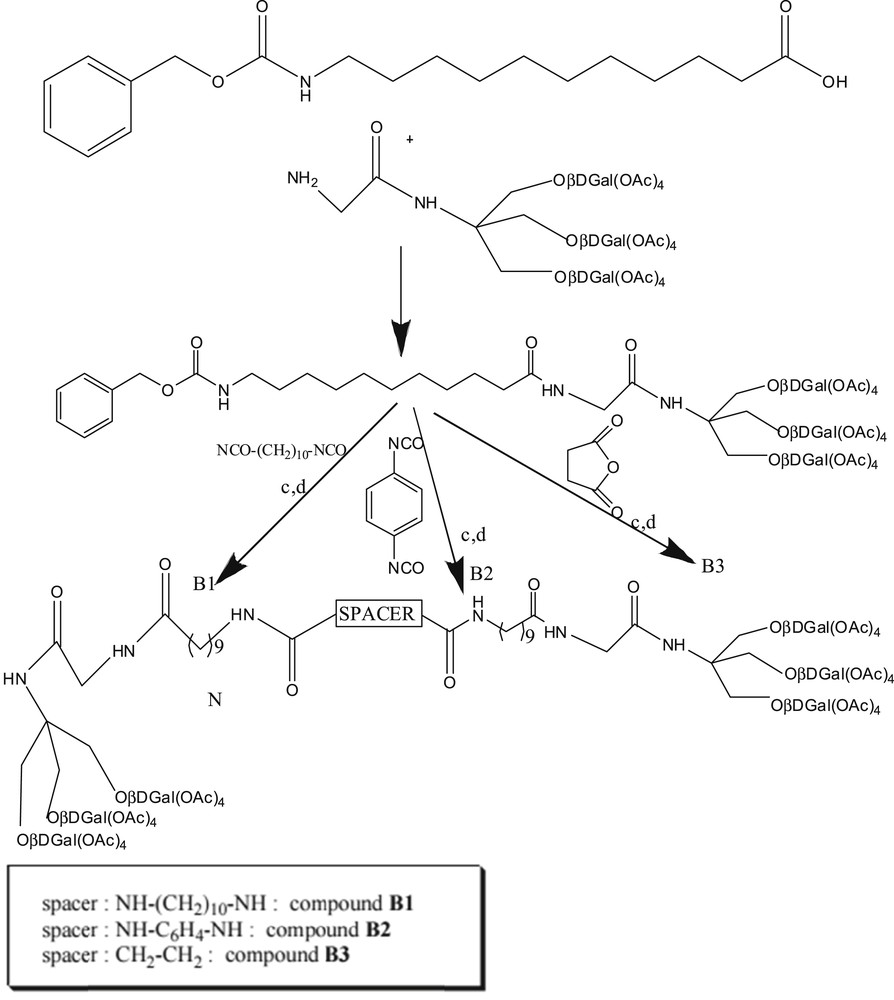
Synthesis of symmetric bolaamphiphiles derived from tris(hydroxymethyl)aminomethane (Tris).
7 Physiochemical properties
Amino acid–based surfactants are known to have a wide range of desirable properties that increase their applicability in varied applications such as good solubilization, good emulsifying properties, high efficiency, high surface activity and good lime resistance (calcium tolerance).
A variety of surface active properties of amino acid–based surfactants like surface tension, cmc, phase behaviour and Krafft temperature have been studied by many scientists and it was concluded that AASs have superior surface activity than their conventional counterparts. Properties of AASs are discussed below.
7.1 Critical micelle concentration
The cmc is an important phenomenon of surfactants as it governs several surface active properties such as solubilization, lytic action and their interaction with biological membranes and so on. Generally, increasing the chain length of the hydrocarbon tail (increasing hydrophobicity) resulted in decreased cmc values of a surfactant solution and hence increased their surface activity [74]. When compared to conventional surfactants, amino acid–based surfactants generally have lower cmc values [70,71].
Infante et al. [29] synthesized three arginine-based AASs by varying the combination of head group and hydrophobic tail (monocationic amide, dicationic amide and dicationic amidoester) and studied their cmc and γcmc values (surface tension at the cmc) and the results showed a decrease in cmc and γcmc values by increasing the length of a hydrophobic tail. In a different study, Singare and Mhatre [48] also confirmed the cmc decreases with an increase in the number of carbon atoms in a hydrophobic tail of Nα-acyl arginine surfactants (Table 1).
cmc and surface tension of Nα-acyl arginine surfactants.
Properties | CAM | NAE | LAE | MAE |
CME (mg L−1) | >1500 | 820 ± 50 | 410 ± 10 | 350 ± 30 |
γ (mN m−1) | 27.0 ± 0.5 | 26.1 ± 0.5 | 25.5 ± 0.5 | 24.0 ± 0.5 |
Yoshimura et al. [65] investigated the cmc values of amino acid–based gemini surfactants derived from cysteine and showed the decreasing pattern in cmc values by increasing the carbon length from 10 to 12 within the hydrophobic chain. Further increases in the carbon chain length up to 14 resulted in an increase in cmc values, confirming the low aggregation tendency of gemini surfactants.
Faustino et al. [75] reported the formation of mixed micelles in aqueous solutions of cystine-based anionic gemini surfactants. The comparison has also been made between the gemini surfactant and its conventional monomeric counterpart, C8Cys. The values of cmc for lipid–surfactant mixtures were reported to be lower than that of the pure surfactant. The gemini surfactant and 1,2-diheptanoyl-sn-glycero-3-phosphocholine, a water-soluble, micelle-forming phospholipid was shown to have cmc values in the millimolar range.
Shrestha and Aramaki [76] studied the viscoelastic wormlike micelles formation in the aqueous systems of mixed type amino acid–based anionic and nonionic surfactants in the absence of salt. In this investigation, N-dodecyl glutamic acid was found to have higher Krafft temperature; however, when neutralized with alkaline amino acid l-lysine, it generated the micelles and the solution started behaving like a Newtonian fluid at 25 °C.
7.2 Good water solubility
Good water solubility of AASs is due to the presence of additional CO–NH linkage [5]. This ability makes them readily biodegradable and environmentally friendly in comparison to their conventional counterparts. The presence of two carboxylic groups leads to even better solubility of N-acyl-l-glutamic acid in water. The presence of two ionic arginine groups in one molecule of Cn(CA)2 also exhibits good water solubility, thus resulting in more effective adsorption and diffusion on the cell interface and showing effective antimicrobial action even at lower concentrations.
7.3 Krafft temperature and Krafft point
Krafft temperature can be understood as the unusual solubility behaviour of surfactants and rapid increase in their solubilities above specific temperature [77]. Ionic surfactants have the affinity to produce solid hydrates through precipitation from the aqueous medium. The solubility of the surfactants will commonly be observed to undergo a sharp and discontinuous increase at some distinctive temperature, which is referred to as the Krafft temperature. Krafft point of ionic surfactants is the Krafft temperature at their cmc [78].
This solubility characteristic is generally observed in ionic type surfactants and can be explained on the basis of limited solubility of nonassociated surfactants below Krafft temperature and the solubility increasing gradually until the Krafft point is reached, which was due to micelle formation. To achieve complete solubility it is necessary to prepare surfactant formulations above their Krafft point [79].
Krafft temperature of AASs has been studied by various researchers, and comparisons were made with the Krafft temperature of conventional synthetic surfactants. Shrestha and Aramaki [76] studied the Krafft temperature of arginine-based AASs, which were found to exhibit premicellar aggregation cmc values above 2–5 × 10−6 M followed by the normal micellization at 3–6 × 10−4. Ohta et al. [80] synthesized six different types of N-hexadecanoyl AASs and discussed their relationship between the Krafft temperature and the amino acid residue.
In an experiment, an increase in the Krafft temperature of the N-hexadecanoyl AAS was found with decrease in the size of the amino acid residue (phenylalanine is an exceptional case), whereas an increase in the enthalpy of solution was found endothermic with decrease in the size of the amino acid residue (except for glycine and phenylalanine). From these observations, it was concluded that d–l interaction was superior to the l–l interaction in solid state of the N-hexadecanoyl AAS salt in both the alanine system and the phenylalanine system.
Brito et al. [81] also determined the Krafft temperatures of three series of novel amino acid–based surfactants using differential scanning microcalorimetry and found the change from trifluoroacetate ion to iodide, which causes a relatively large increase (≈6 °C) in the Krafft temperature from 47 to 53 °C. The presence of a cis-double bond and unsaturation present in long chain Ser-derivative significantly decreases the Krafft temperature. N-Dodecyl glutamic acid was reported to have higher Krafft temperature. However, neutralization with alkaline amino acid l-lysine resulted in micelle formation in the solution, which behaves like a Newtonian fluid at 25 °C.
7.4 Surface tension
Surface tension of surfactants increased with increasing chain length of the hydrophobic moiety. Zhang et al. [82] determined the surface tension values of sodium cocoyl glycinate by the Wilhelmy plate method using a DCAT 11 Tensiometer at 25 ± 0.2 °C and obtained surface tension values of 33 mN m−1 at the cmc of 0.21 mmol L−1. Yoshimura et al. [83] determined the surface tension of some 2CnCys type amino acid–based surfactants. The surface tension at the cmc was found to decrease when increasing the chain length up to n = 8, whereas the trend reversed for surfactants with n = 12 or longer chain lengths.
The effect of CaCl2 on surface tension of dicarboxylic amino acid–type surfactants has also been studied [84,85]. In these investigations, addition of CaCl2 in aqueous solutions of three dicarboxylic amino acid–based surfactants (C12MalNa2, C12AspNa2 and C12GluNa2) was carried out. Surface tensions were found to be lower at very low CaCl2 as compared to that at the plateau value beyond the cmc. This was due to the effect of calcium ions on the packing of the anionic surfactants at the air–water interface. Surface tension of the salts of N-dodecyl aminomalonic acid and N-dodecyl aspartic acid remained almost constant up to a 10 mM concentration of CaCl2. Above 10 mM, a rapid increase in surface tension was observed, which was due to the formation of calcium salt precipitate of the surfactant. In case of disodium salt of N-dodecyl glutamic acid, a considerable decrease in surface tension was observed by moderate addition of CaCl2 that remained almost constant over a broad range of CaCl2 concentrations.
For the determination of adsorption kinetics at air–water interface for gemini AASs, dynamic surface tension measurements were carried out via the maximum bubble pressure method. Results revealed no change in the dynamic surface tension for 2C12Cys for the longest measurement time. Reduction in the dynamic surface tension only depends on the length of hydrocarbon tail, number of hydrocarbon tails and the concentration. Faster decay was observed by increasing the concentration and decreasing the chain length and chain numbers of the surfactants. Results obtained at higher concentrations of CnCys (n = 8–12) were found very close to the γcmc obtained through Wilhelmy surface tension.
In another study [86], the dynamic surface tension of sodium dilauroyl cystine (SDLC) and sodium didecamino cystine was determined using Khan DCA-315 Tensiometer through the Wilhelmy plate technique, and the equilibrium surface tension of their aqueous solution was measured by the drop volume method. Further studies on the reaction of the disulfide bond were also performed by other methods. Addition of mercaptoethanol to 0.1 mmol L−1 SDLC solution resulted in the rapid increase in surface tension from 34 to 53 mN m−1. As NaClO can oxidize the disulfide groups of SDLC to sulfonic groups, when NaClO (5 mmol L−1) was added into 0.1 mM L−1 SDLC solution, no aggregate was observed. Transmission electron microscopy and differential light scattering results showed no aggregate formation in the solution. The surface tension of SDLC was found to increase from 34 to 60 mN m−1over the duration of 20 min.
7.5 Binary surface interaction
The vibrant properties of the mixture of cationic-type AASs (diacyl-glycerol-arginine–based surfactant) and phospholipids at the air–water interface for life sciences have been reported by many research groups, and it was finally concluded this nonideal characteristic is an asset to the pervasiveness of electrostatic interactions [87,88].
7.6 Aggregation properties
Differential light scattering measurements are commonly used to determine the aggregation properties of amino acid–based monomeric and gemini surfactants at concentrations higher than the cmc, and the diffusion coefficient so obtained is converted into the apparent hydrodynamic diameter DH (=2RH). When compared to the other surfactants, CnCys and 2CnCys formed relatively large aggregates with a wide size distribution. Surfactants other than 2C12Cys generally form aggregates of about 10 nm. The micelle size for gemini surfactants was found significantly larger than their monomeric counterparts [67]. An increase in the hydrocarbon chain length also results in the increase in the micellular size [89,90]. Ohta et al. [91] described the aggregation properties of aqueous solutions of three different stereoisomers of tetramethyl ammonium N-dodecyl-phenyl-alanyl-phenyl-alaninate and revealed that the diastereomers have same critical aggregation concentrations in aqueous solutions. Iwahashi et al. [92] investigated the formation of chiral aggregates of optically active N-lauroyl-l-glutamic acid, N-lauroyl-l-valine and its methyl ester in different solvents like tetrahydrofuran, acetonitrile, 1,4-dioxane and 1,2-dichloroethane through the measurement of circular dichroism, NMR chemical shift of N–H proton and vapour pressure osmometry.
7.7 Interfacial adsorption
Amino acid–based surfactants have also been characterized in terms of their interfacial adsorption and compared with their conventional counterparts. Dodecyl ester of aromatic amino acids obtained from LET and LEP was subjected to assess their interfacial adsorption characteristics [93]. Results revealed that LET and LEP exhibit lower interfacial area at the air/solution and in water/hexane interface, respectively.
Bordes et al. [84,85] investigated the solution behaviour and adsorption of three dicarboxylic amino acid–based surfactants, the disodium salts of dodecyl glutamic acid, dodecyl aspartic acid and dodecyl aminomalonic acid with three, two and one carbon atoms, respectively, between the carboxyl groups at the air–water interface. It was reported that cmc of dicarboxylic surfactants was four to five times higher than monocarboxyl group containing dodecyl glycinate. This was attributed to the presence of hydrogen bonding between adjacent molecules through the amide groups in the dicarboxylic surfactants.
7.8 Phase behaviour
Phase behaviour of the surfactants can be determined using sealed ampoules containing requisite quantities of reagents, which were mixed at higher temperatures and get homogenized with a vortex mixer through repeated centrifugation. After mixing properly, the samples are kept in a thermostatically controlled water bath at 25 °C for a few days to ensure equilibrium. The equilibrated phases were acknowledged by visual observations using normal and crossed polarizer. At a high concentration of the surfactant, the isotropic discontinuous cubic phase was observed. A large hydrophilic head group of the surfactant molecule favoured the small and discrete aggregates of positive curvature [94]. Marques et al. [95] studied the phase behaviour of 12 Lys12/12 and eight Lys8/16 Sersystems and stated that the 12 Lys12/12 Sersystems had phase separation between the micellar and vesicle solution. Small micelles were found to coexist in between the vesicles in the former, whereas eight Lys8/16 Sersystems showed a continuous transition process (where the elongated micelles were found to be present in between the small micelles and vesicles).
7.9 Emulsification
Kouchi et al. [96] examined the emulsification potency of N-[3-lauryl-2-hydroxypropyl]-l-arginine, l-glutamate, along with other AASs, in terms of interfacial tension, dispersability and viscosity. Comparison of synthesized surfactants has also been made with their conventional nonionic and amphoteric counterparts and results revealed that the AASs have greater emulsification potency than conventional surfactants.
Baczko et al. [97] synthesized novel amino acid–based anionic surfactants and investigated their usability as chiral oriented NMR spectroscopy solvents. A series of sulfonated amphiphilic l-Phe or l-Ala derivatives with pentyl to tetradecyl tails were synthesized by reacting the corresponding amino acid esters with o-sulfobenzoic anhydride. Wu et al. [98] synthesized the sodium N-fatty acyl AAS using pupa oil and obtained pupa protein hydrolysates as a waste byproduct of the silk industry. Studies on the emulsifying power of pupa protein hydrolysates and sodium N-fatty acyl amino acid in an oil/water emulsion revealed that these surfactants were superior with ethyl acetate as the oil phase over n-hexane.
7.10 Lime tolerance
Lime tolerance can be understood as the resistance of the surfactant to precipitate as lime soap in the presence of calcium- and magnesium-like ions present in hard water. Surfactants with high tolerance against water hardness were found useful for detergent formulations and personal care products. Determination of the lime tolerance can be achieved by calculating the solubility and the surface activity change in surfactants in the presence of calcium ions [84,85]. Another method used to determine lime tolerance involves the calculation of percentage or the number of grams of the surfactant required for the dispersion of lime soap formed from 100 g sodium oleate in water [99]. In hard water areas, high concentrations of calcium and magnesium ions and mineral content can lead to difficulties in some practical applications. Sodium ions are generally used as the counterions in the synthesis of anionic surfactants. By virtue of being bivalent calcium ions bind to two surfactant molecules resulting in the easier surfactant precipitation from solution and thus reducing detergency. Studies on lime tolerance of AASs showed that ‘acid and lime tolerance’ is vividly augmented by an additional carboxyl group, and they further increase with increase in the length of the spacer group between two carboxyl groups. The order of acid and lime tolerance found was C12 Glycinate < C12 Aspartate < C12 Glutamate. Comparison of dicarboxylamido linkage surfactants with dicarboxyl amino linkage surfactants revealed that dicarboxyl amino linkage surfactants have wider pH range, in which surface activity increases with moderate addition of acid. Dicarboxyl N-alkyl amino acids have a chelating effect in the presence of calcium ions and C12 Aspartate forms white gel. C12 Glutamate shows high surface activity at a high concentration of Ca2+ and has the potential to get used in seawater desalination [100].
7.11 Dispersability
Dispersability is the ability of surfactants to inhibit the agglomeration and settling down of surfactants in solution. Dispersability is an important property of surfactants that make them suitable for detergents, cosmetics and pharmaceuticals [101]. Dispersing agents must contain ester, ether, amido or amino linkage between the hydrophobic group and the terminal hydrophilic group, as well as a straight-chain hydrophobic group [102]. Generally, the anionic surfactants like sulfated alkanolamide and zwitterionic surfactants like amidosulfobetaine are found to be very effective as lime soap dispersing agents [103–106].
Various studies have been made to determine the dispersability of AASs, in which N-lauroyl lysine was found to have poor compatibility with water and was found difficult to make cosmetic formulations. In this series, N-acyl basic amino acid was found to have superior dispersability and is being used in cosmetic industries to improve formulations [107].
8 Toxicity
Conventional surfactants, especially cationic surfactants are intensely toxic against aquatic life, which includes algae, fish and molluscs. Their acute toxicity is because of their tendency to disrupt the integral membrane, which is due to the adsorption–ionic interaction phenomenon of the surfactant at the cell–water interface. Lowering the cmc of surfactants typically results in greater adsorption of surfactants onto interfaces, typically raising their acute toxicity. Increasing the length of a hydrophobic chain of the surfactant molecule also results in the increase of acute toxicity of the surfactant. Low toxicity or nontoxicity of most AASs to human and the environment, specifically to marine organisms, makes them suitable as food ingredients, medicines and cosmetics [107–113]. Various researchers have verified amino acid–based surfactants as mild and nonirritating to the skin [110]. Arginine-based surfactants are known to be less toxic than their conventional counterparts. Brito et al. [89,90] studied the physicochemical and toxicological properties of amino acid–based amphiphiles and their spontaneously formed cationic vesicles derived using tyrosine (Tyr), hydroxyproline (Hyp), serine (Ser) and lysine (Lys) and presented data for their acute toxicity to Daphnia magna (IC50). They synthesized cationic vesicles of dodecyltrimethylammonium bromide (DTAB)/Lys-derivative and or Ser-/Lys-derivative mixtures and tested their ecotoxicity and haemolytic potential and stated that all AASs along with their vesicle-containing mixtures showed lower ecotoxicity than the conventionally used surfactant DTAB. Rosa et al. [114] studied the association of DNA and stable cationic amino acid–based vesicles. As compared to conventionally used cationic surfactants that are generally toxic, the interaction of cationic amino acid–based surfactants appeared to be nontoxic. This cationic AAS based on arginine, ALA, gives spontaneously stable vesicles with some with anionic surfactants. Corrosion inhibitors based on amino acids were also reported to be nontoxic [115–120]. These surfactants were also found to be easy to synthesize with high purities (up to 99%), low in cost, readily biodegradable and were completely soluble in aqueous media. In various studies, sulfur containing amino acid–based surfactants were found to be more competent corrosion inhibitors [115,121–125].
Perinelli et al. [126] in their latest investigation reported the favourable toxicological profile of rhamnolipids over conventional synthetic surfactants. Rhamnolipids were already known as permeability enhancers. They have also reported the impact of rhamnolipids on the epithelial permeability of macromolecular drugs.
9 Antimicrobial activity
Antimicrobial activity of surfactants can be calculated on the basis of minimal inhibitory concentration [29]. Arginine-based surfactants have been studied in detail for their antimicrobial properties [23,28,30,31,47,65]. The Gram-negative bacteria were found to be more resistant to arginine-based surfactants than Gram-positive bacteria. Antimicrobial activity of surfactants typically increases by the presence of hydroxyl group, cyclopropane or unsaturation within the acyl chain. Castillo et al. [127] stated that the length of acyl chains and the positive charge that determine the HLB (hydrophile lipophile balance) of the molecule do affect their membrane disrupting property. Nα-acyl arginine methyl ester is another important class of cationic surfactants that have broad-spectrum antimicrobial activity with easy biodegradability and less or no toxicity [17,128]. Studies on the interaction of Nα-acyl arginine methyl ester–based surfactants with 1,2-dipalmitoyl-sn-glycero-3-phosphocoline and 1,2-dimiristoyl-sn-glycero-3-phosphocoline, model membranes, and with living organisms with or without external barriers also revealed their good antimicrobial activity [48].
10 Biodegradability
Kamimura [128], Shida et al. [129,130] and Kubo et al. [131] extensively studied the biodegradability of amino acid–based surfactants and found that N-acyl amino acids easily biodegrade through decomposition into amino acid and fatty acids.
Single hydrophobic chain containing surfactants are comparatively more biodegradable than their branched counterparts such as bis(Args). Typically, the more hydrophobic the surfactant, the poorer will be their biodegradability. Akinari et al. [132] synthesized the AASs based on fatty acids and studied their physicochemical properties and biodegradability. Biodegradation studies of these surfactants showed their microbial degradation between 57% and 73% over 14 days. Zhang et al. [133] prepared supramolecular hydrogel mixtures of the biosurfactants based on sodium deoxycholate and amino acids such as glycine (Gly), alanine (Ala), lysine (Lys) and arginine (Arg) using different buffered solutions and claimed their unique sensitivity towards multistimuli environments, their facile biodegradability and pH-sensitiveness makes them promising and versatile vehicles for dye (or drug) delivery.
11 Haemolytic activity
Nogueira et al. [134] investigated the five anionic lysine-based AASs that differ on the basis of their counterion and examined their ability to disrupt the cell membrane under varied pH range, concentrations and incubation period. For this purpose, they used a standard haemolysis assay as a model for endosomal membranes. Results confirmed the pH sensitive haemolytic activity and better kinetics of these surfactants at the endosomal pH range.
Surfactants are known to have the capability to interact with lipid bilayer of cell membranes. Red blood cell is one of the most frequently used cellular membranes as a reference model to investigate the mechanisms of fundamental surfactant-induced osmotic cell resistance. Pérez et al. [15] studied the mechanisms of surfactant membrane interaction by monitoring the action of three arginine-based cationic AASs and five lysine-based anionic AASs on hypotonic haemolysis. Results revealed the dissimilar antihaemolytic behaviours among amino acid–based surfactants, both linked to the maximal protective concentration. Physicochemical and structural properties of these compounds dictated the protection against hypotonic haemolysis. A good correlation was found in between the cmc and the concentrations of cationic surfactants that resulted in maximum protection against hypotonic haemolysis. In contrast, no correlation was observed for the anionic surfactants. Lysine-based surfactants differ only in their counterions, this difference being responsible for their antihaemolytic potency and the haemolytic activities.
Toxicological study revealed that the capability of arginine-based monomeric and gemini surfactants to disrupt the erythrocyte membranes depends on size and hydrophobicity [135].
Pinheiro and Faustino [136] discussed erythrocyte interaction with Nα, Nε-dioctyl lysinate salts with different counterions (Li+, Na+, K+, Lys+ and Tris+). Surfactants interacted with erythrocyte membranes in a biphasic way, at low concentrations through protecting against hypotonic haemolysis and at high concentrations by inducing haemolysis.
12 Rheological properties
Rheological properties of surfactants play a very important role to decide/predict their applications in different industries such as food, pharmaceuticals, oil extraction and in personal/home care products [137–139]. The relation of viscoelastic characteristics and cmc of amino acid–based surfactants have been discussed in many studies [76,140,141].
13 Industrial applications
Special structural characteristics, nontoxicity and biodegradability of the AAS make them suitable for various industrial applications.
13.1 In agriculture
AAS can be used as insecticides, herbicides and plant growth inhibitors for agricultural means. Betaine ester surfactants are a class of cationic surfactants that can be used as ‘temporary biocides’ and can be hydrolyzed very easily into harmless components [36,38,129].
A lawn pesticide was also reported in a US patent that was synthesized using the mixture of refined oils extracted from the plants of Cupressaceae family and an amino acid–derived surfactant solution, in which amino acid–derived surfactant contributed 20–50% by weight of the said solution. Herbicidal action of the nonionic-type AAS has also been reported [130].
13.2 In laundry detergents
Nowadays, the demand for amino acid–based detergent formulations is increasing globally. AASs are known to have better cleaning ability, foaming ability and fabric softening properties that make them suitable to be used in household detergents, shampoos, body wash and so on. An AAS derived from aspartic acid is reported as an effective detergent with ampholytic and chelating characteristics. Less skin irritating effects were observed by using detergent compositions made up of N-alkyl-β-amino-ethoxyacids [142]. Liquid detergent compositions made up of N-coco-β-aminopropionate were claimed to be an effective cleaning agent for grease mark on metallic surfaces [143,144]. An aminocarboxylic surfactant, C14CHOHCH2NHCH2COONa, was also proved to have better detergency and used to clean textiles, carpets, hair, glass and so on. [145]. 2-Hydroxy-3-aminopropionic acid-N,N-diacetic acid derivatives were also known to incorporate stability in bleaching agents with good complexing ability.
Detergent composition based on N-(N′-long-chain acyl-β-alanyl)-β-alanine was reported to have better ability and stability, foam breakage and good softening properties [145]. Keigo and Tatsuya [146] prepared and patented acyl amino acid–based detergent compositions. Kao formulated N-acyl-1-N-hydroxy-β-alanine–based detergent compositions and claimed their low skin irritation property, high water resistance and high detergency [147]. Ajinomoto Company used low toxic and easily degradable, l-glutamic acid, l-arginine and l-lysine–based AASs as the principal ingredients in shampoos, detergents and cosmetics (Fig. 9) [148]. Ability of enzymatic additives in detergent compositions to remove protein-based soilings has also been documented [149]. N-acyl AASs derived from glutamic acid, alanine, methyl glycine, serine and aspartic acid were reported as good liquid cleaning agents in aqueous solutions. These surfactants did not show any increase in viscosity even at very low temperatures and can easily transfer from foamer container, thus providing uniform foam [150].
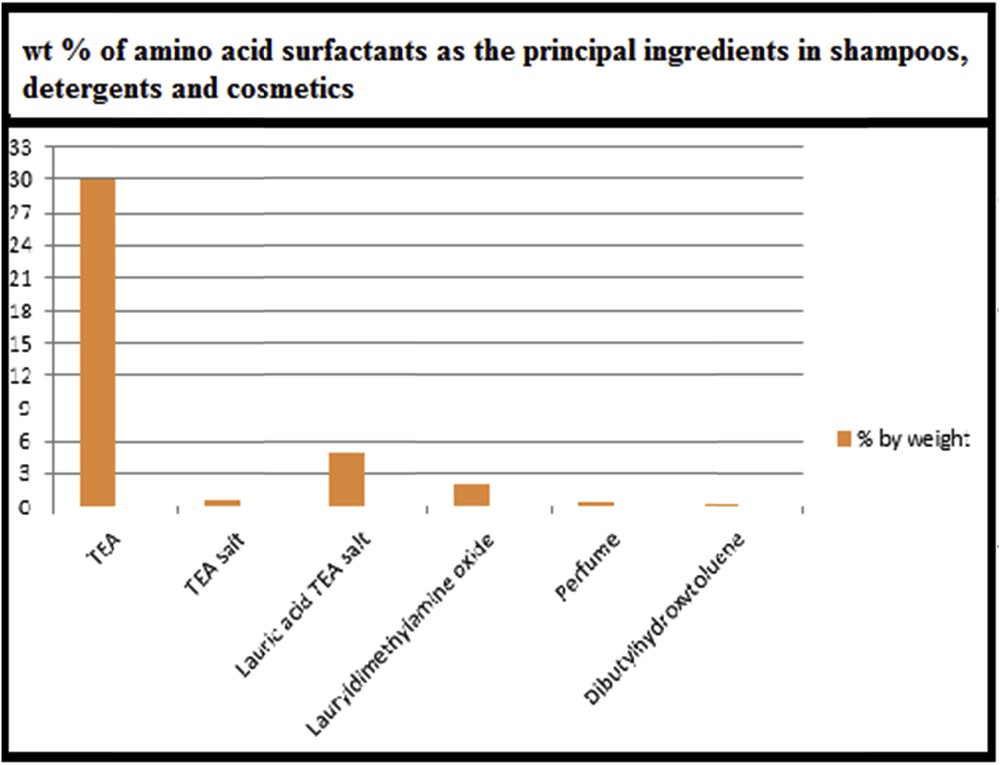
Weight percent of AASs as the principal ingredients in shampoos, detergents and cosmetics.
13.3 Lubricants
Amphoteric-type AASs are generally used as lubricants. Having excellent adhesive property for hydrophilic surfaces and low friction coefficient make them ideally suited to be lubricants. Various researchers prepared and studied the lubricating properties of AAS, specifically, glutamic acid [151–153].
13.4 In medicine
13.4.1 Drug carriers and preparation of functional liposomes
In the recent years, various researchers have claimed the ability of synthetic acyl amino acid/peptide as a drug carrier and for preparing functional liposomes with lipopeptide ligands. As compared to the conventional liposomes of lecithin, vesicles of long aliphatic chain Nα-acyl amino acids also showed encapsulation efficiencies for solutes [154,155].
13.4.2 Gene therapy and DNA transfection
Gene therapy is a very important technique currently used in life science to safely introduce selected gene into living cells. Gemini surfactants have been found to have potential to be used as a carrier for the transportation of bioactive molecules. Lysine and 2,4-diaminobutyric acid–based polycationic gemini surfactants can be easily synthesized using standard peptide chemistry [156].
McGregor et al. [157] prepared a new class of amino acid–based gemini surfactants as a carrier for gene delivery into cells. Preliminary results revealed that combining these amino acid–based gemini surfactants with dioleoylphosphatidylethanolamine (DOPE) allowed the synthesis of liposomes of different sizes and lipid compositions. Investigation revealed that suspensions of DOPE/surfactants mixtures in water lead to a mixture of lipid vesicles with more complex structures corresponding to particles e500 nm in diameter. Different molar ratios of DOPE and surfactants (50/50, 60/40 and 70/30) were found to effect luciferase expression in Chinese hamster ovary (CHO) cells at comparable levels. The size of colloid and the molecular composition of the gemini surfactants matter in delivering optimum gene expression in living models.
DNA transfection efficiency of cysteine-based AASs with low molecular weight and its corresponding gemini were evaluated by Peña et al. [158]. These surfactants showed no cytotoxicity and had greater efficacy than their commercially available counterparts to transfect CHO-K1 (Chinese hamster ovary) cells.
13.4.3 Antiviral agents
Lipoamino acids are attracting attention to researchers due to their significant antiviral activity. Some acyl amino acid derivatives have also been reported to inhibit influenza neuraminidase [159]. Several Nα-palmitoylated amino acids/peptides when integrated into model membranes affected the transition temperature between the surface tension at their cmc.
AAS-derived cationic surfactants obtained by condensation of fatty acids such as lauric acid and esterified dibasic amino acids such as arginine may be exploited for the protection against the microorganism and these cationic surfactants were also found to be effective against viral infections. Furthermore, the addition of ethyl-N-α-lauroyl-l-arginate into the cultures of Herpes virus type 1 Vaccinia virus and bovine parainfluenze 3 virus leads to almost complete diminution of the virus organisms in these cultures, such effects being observed from 5 to 60 min [160].
13.5 In food processing industry
AASs are widely used as emulsifiers in the food sectors such as in margarine, dairy products, low-calorie spreads and dressings [161]. In addition, new insights into eating habits and health aspects need the changes in the food formulations time to time in terms of calories, fat content, vitamins or minerals that lead to a constant demand of optimizing product formulations. These surfactants also include optimal selection of raw materials. Formerly, industries were giving great attention to the different glycerides, most commonly to monoglyceride derivatives but due to health consciousness, arginine-based AASs with antimicrobial activity and wide antibacterial spectra are receiving great interest as a promising alternative to commercially available pure glycerides in food formulations. These surfactants additionally show considerable antimicrobial activity against Escherichia coli and Salmonella, which are the most common food borne pathogens and create a serious health hazard due to increased drug resistance [162].
13.6 In cosmetic industry
AASs are used in the formulation of various personal care products. Potassium N-cocoyl glycinate was found to be mild to skin and applied and used in face cleansers to remove soil and makeup. N-acyl-l-glutamic acid has two carboxylic groups, which lead to better water solubility. Among these, AASs based upon C12 fatty acid are extensively used in face cleansers to clean soil and makeup. AASs with C18 have found to be used as emulsifiers in skin care cosmetics. N-dodecanoylalaninate is known to have ability to create nonirritating creamy foam for skin, and therefore used to formulate baby care products. N-lauroyl–based AAS used in toothpaste shows good detergency similar to soap and strong enzyme-inhibiting efficacy [102].
In the past few decades, in the selection of surfactants in cosmetics, personal care products and pharmaceuticals, less toxicity, mildness, gentleness and safety are becoming the major concerns. Consumers of these products are genuinely conscious for the potential irritation, toxicity and environmental factors. Nowadays, AASs are used to formulate many shampoos, rinses, and body soaps because of the numerous merits over their conventional counterparts for use in cosmetics and personal care products. Protein-based surfactants possess desirable properties that a personal care product must have [103]. Some AASs are known to have film forming ability, whereas others have good foaming ability. Amino acids are important naturally occurring moisturizing factors present in the stratum corneum. When epidermal cells die they become a part of the stratum corneum, and the proteins present in the cells gradually degrade into amino acids. These amino acids are then transported further into the stratum corneum, absorb the fat or fatlike substances into horny layer and thus improve the elasticity of the skin surface. About 50% of the naturally occurring moisturizing factors present in the skin are made up of amino acids and pyrrolidone [163]. Collagen, a common cosmetic ingredient also comprises amino acids, which keep the skin supple. Various skin problems such as roughness and dark colouration of the skin are largely due to the deficiency of amino acids. A case study revealed that mixing of an amino acid with ointment gives relief to skin burn and the affected area returns to the normal state and does not turn into a keloid [164].
Amino acids are also found very useful for the care of damaged cuticles. Lacklustre and dry hair can indicate a decrease in amino acid concentration in heavily damaged cuticles. Amino acids have the ability to penetrate through the cuticle into hair shaft and drawing in moisture from the skin. This capability of amino acid–based surfactants makes them useful in shampoos, rinses, hair softeners, hair treatment agents, and the presence of amino acids makes hair less breakable [23].
13.7 In microbial enhanced oil recovery
AASs are also found useful in microbial enhanced oil recovery. A bacterium strain, Brevibacterium aureum MSA13, synthesized an AAS that has potential for use in microbial oil recovery. This surfactant has octadecanoic acid as a hydrophobic moiety and a hydrophilic group comprises a tetrapeptide, a short sequence of four amino acids pro-leu-gly-gly. Another example involved in microbial enhanced oil recovery in marine surroundings is an actinobacterium, MSA13 produced surfactant [165].
13.8 In nanomaterials
Amino acid–based polymerizable surfactants were also found to be useful in the synthesis of chiral nanoparticles. Preiss et al. [166] reported the synthesis of amino acid–based chiral surfactants with polymerizable moieties, which were then exploited to prepare nanoparticles with a chiral surface functionality. They also checked their potential as nucleating agents in the enantioselective crystallization of amino acid conglomerate systems, taking a model system of rac-asparagine. Comparisons were made in the particles synthesized from chiral surfactants having different tail groups and results revealed that only the chiral nanoparticles made up of polymerizable surfactants were found to have ability to act efficiently as a nucleation agent in the enantioselective crystallization.
13.9 Other applications
AASs are also known for use in forming PEDOT [poly(3,4 ethylenedioxythiophene)] films [167–176]. PEDOT films through direct anodic oxidation of EDOT (3,4-ethylenedioxythiophene) in aqueous solution comprising sodium N-lauroyl sarcosinate, an eco-friendly AAS, were also prepared. In addition to the above-mentioned applications, AASs are also used in the optimization of dry-cleaning process with carbon dioxide and as chiral solvents [177–180].
Van Roosmalen et al. [179] have optimized the dry-cleaning process using carbon dioxide along with the AASs and studied the impact of various reaction parameters on the cleaning. The reaction parameters studied were the cleaning time, temperature and power involved in mechanical action. In addition, the influence of the quantity of each substance used such as CO2, isopropyl alcohol, water and surfactant was also investigated.
Self-assembly of AASs resulted into micelles with a chiral surface. Such a property of AAS micelles of being supramolecularly chiral makes them suitable for asymmetric organic synthesis [181]. The chiral micelles can also impart chirality in mesoporous materials prepared through surfactant templating route and make their pores chiral. Dicarboxylic-based AASs can also behave like surface active chelating agents. It has been observed that the interaction of dicarboxylic AASs with divalent ion-like calcium is reliant on the distance between the two carboxyl groups. For example, N-acyl glutamate that has three –CH2 groups between the carboxyl groups does not form an intramolecular chelate with calcium and is not able to precipitate in water with high concentrations of calcium. The binding property of chelating surfactants can also be used in mineral ore flotation. Calcium-containing minerals such as calcite and apatite can be separated by means of a flotation reagent with the right distance between the carboxyl groups. N-alkyl AASs are true amphoteric-type surfactants that can provide exceptionally low values of surface tension at the cmc, which is due to the formation of self-assemblies consisting of alternating anionic and cationic species. This represents the micellization-driven protonation of surfactants.
AASs can also be used to design switchable surfactants. Cysteine derivatives are the best-known examples of this class that can readily covert into cystine derivatives via reversible processes. For example, long-chain N-acylcystine, a gemini surfactant, is highly surface active and can be transformed into a cysteine derivative. Dithiothreitol, a poor surface active agent, can also revert back to the gemini surfactant via an oxidation. The exchange from one state to the other can also be achieved through electrochemical means [182].
14 Current challenges for AASs
The economic feasibility of large-scale production of AASs is a major issue to be surmounted. Biotechnological processes involved in their production are not easily cost effective especially for the processes where bulk use of surfactants is required such as petroleum and environmental application. Purification of substances is another problem linked to these surfactants, which is needed for pharmaceutical, cosmetic and food applications. Along with these disadvantages, denaturation and dissociation of AASs into their subunits and their activity are largely affected by salt solutions.
Various researchers have suggested remedies to overcome these problems. The overall cost cutting in their production can be achieved through the use of a waste substrate after combating their polluting effect. The development of efficient bioprocesses and their successful optimization is also required, which include the optimization of the culture conditions and cost-effective recovery processes for the maximum production and recovery [183–185].
15 Conclusions
Although conceptually simple the process of combining amino acids with a hydrophobic tail can by its very nature offer a variety of synthetic routes, this in turn leading to many potential structures. Since the first studies on the synthesis of simple AASs in 1909 research has extended into the production of cationic, anionic, nonionic and amphoteric molecules, with detailed characterization and assessment of physiochemical properties performed throughout. AASs have been shown to have wide reaching applications over different industrial sectors, and the diversity in properties borne out of their potential for structural variety will in the future allow for this range of applications to grow further. In addition, facile biodegradability and nontoxicity of AASs can make them superior to their conventional synthetic counterparts when selected for the right application. Examples even exist whereby the chirality of AASs has been exploited in use, such as imparting chirality into the surface of micelle template mesoporous materials. AASs have been reported to have a wide range of applications in different industrial sectors. Facile biodegradability and nontoxicity of AASs can make them superior to their conventional synthetic counterparts when selected for the right application. The major challenges associated with AASs are their high production cost and their challenging isolation to high purity. Various researchers are working to minimize these problems through selecting suitable renewable alternatives as a substrate along with designing cost effective and scalable procedures. AASs have the potential to be widely accepted commercially in various industrial sectors in near future, especially so when the diversity of their structure and physiochemical properties are considered.
Acknowledgements
D.B.T. acknowledges the Department of Science and Technology, Government of India, for financial support through grant no. SR/WOS-A/CS-1056/2014 (G).