1 Introduction
Synthetic metalloporphyrins are regarded as an ideal platform for catalysis with peroxidase-like activity and draw much interest because of their versatile applications such as epoxidation of alkenes, oxidative degradation of xenobiotics, biosensors, and so on [1–6]. Among the biomimetic metalloporphyrins, iron (Fe) and manganese (Mn) stick out, due to their availability and importance of redox cofactors. In sharp contrast to Fe, analogous Mn porphyrin–catalyzed oxidations remained undeveloped. Mn reconstituting heme proteins such as horseradish peroxidase (MnIII HRP) [7], microperoxidase-8 (MnIIIMP-8) [8], cytochrome c peroxidase (MnIIICcP) [9], and myoglobin (MnIIIMb) [10] have been prepared, but showed much lower reactivity toward hydrogen peroxide (H2O2).
Efforts were made to improve their activities by introducing amino acid residues with imidazole functional groups [11–13]. Similar works have been done for synthetic Mn porphyrins. The additives containing nitrogen such as imidazole and its derivatives [6,14,15] and pyridine derivatives [16] are most applied because of the axial ligand effect due to the formation of hydrogen bond between nitrogen and H2O2 coordinated at the Mn center. This effect is generally more pronounced in the reactions catalyzed by MnIII porphyrins [17]. Other methods reported with higher catalytic activation of H2O2 include the choice of Mn porphyrin nanocomposite with the tail of carboxylic groups [6,14], the addition of a carboxylic acid [16], and the immobilization of Mn porphyrin to supports (aminofunctionalized montmorillonite [17], chlorinated graphene oxide [18], modified multiwall carbon nanotube [19], and so on).
Because most synthetic models are designed to function in generally organic homogeneous solution rather than the environmentally benign aqueous media [5], water-soluble mimics come into notice. The biocompatible polymer with imidazole groups and carboxymethylated imidazolium groups was used to support Mn porphyrin in the preparation of the water-soluble mimics [20].
Murakami and Konishi [21] found the remarkable cocatalytic effect of gold nanoclusters on olefin oxidation catalyzed by the Mn porphyrin nanocomposite. Au nanoparticles (AuNPs) have enormous applications due to their unique physicochemical features such as biocompatibility, amphilicity, larger surface carrier capabilities, and gold electronic conductivity [22–24]. However, their tendency of agglomeration needs to be prevented for effective use. To synthesize well-dispersed AuNPs, various nanoreactor systems have been used; among them, polyethyleneimine (PEI) displays the reduction of AuCl4− to AuNPs under mild conditions and steric stabilization for AuNPs [25–28]. In our previous study, PEI–AuNPs–hemin nanocomposite was proved to have a much-improved peroxidase-like catalysis for decomposing H2O2 [28], which indicated that the PEI–AuNPs can enhance the catalytic activity of metalloporphyrins.
In this study, we designed and synthesized a nanocomposite of MnIII protoporphyrin IX chloride (MnIIIPPIX) associated with PEI–AuNPs, which is in a stable colloidal state in water. The oxidative degradation of azo dyes is often used to test the efficiency of peroxidase and its mimic system [29–36]. We herein used PEI–AuNP–MnIIIPPIX nanocomposite-catalyzed oxidative degradation of acid orange 7 (AO7), a kind of azo dye, as the model reaction and reported the notable peroxidase-like catalytic ability of this nanocomposite. The possible mechanisms are also proposed.
2 Experimental section
2.1 Materials and apparatus
All the reagents used here were of analytical grade. In particular, branched PEI (average molecular weight, Mw = 25 kDa) was purchased from J&K Scientific Ltd. (Shanghai, China). Hydrogen tetrachloroaurate(III) tetrahydrate (HAuCl4·4H2O) was purchased from Sigma–Aldrich (St. Louis, MO) and MnIIIPPIX from Frontier Scientific Inc. AO7 (C.I. 15510) was purchased from Beijing Chemical Reagents Co.
UV–vis spectra were recorded using a UV-3600 UV–VIS–NIR spectrophotometer (Shimadzu, Japan). Fourier transform infrared (FTIR) spectrum analysis was performed using an FTIR-8400S spectrophotometer (Shimadzu, Japan). Transmission electron microscopy (TEM) image was obtained using a JEOL JEM-2100 transmission electron microscope at an acceleration voltage of 200 kV.
2.2 Preparation and characterization of PEI–AuNPs–MnIIIPPIX
PEI–AuNPs were synthesized as described in our previous report [28]. Then, the PEI–AuNPs were added into a 0.1 mM MnIIIPPIX solution (in 0.1 M phosphate-buffered saline [pH 9.0]) in a stoichiometric ratio of 1:0.12. The mixture was stirred at 25°C. After 1 h, the mixture was centrifuged at 14,000 rpm for 60 min, and then the precipitate was washed and centrifuged again in ultrapure water for three times. So prepared PEI-AuNPs-MnIIIPPIX was dispersed in water with a final concentration of 0.5 mg/mL and stored at 4°C in the dark for further use.
2.3 Catalytic experiments of PEI–AuNPs–MnIIIPPIX
AO7 stocking solution (1000 mg/L) was prepared by dissolving it in 0.1 M phosphate-buffered saline at various pHs between 1.0 and 9.0. The AO7 oxidative degradation was carried out at 25°C in the dark for 120 min as follows: certain amounts of the catalyst were added into the 24 mg/L AO7 solution and the reaction was initiated by adding precalculated amount of H2O2. The residual AO7 was quantified by spectrophotometric assay at 484 nm, the maximum peak of AO7. Control reactions in different systems were carried out.
3 Results and discussion
3.1 Preparation and characterization of the catalyst, PEI–AuNPs–MnIIIPPIX
Scheme 1 shows the preparation route for PEI–AuNPs–MnIIIPPIX. PEI was used as a reducing and protective agent to prepare the PEI–AuNPs by one-step thermal process synthesis [28]. Next, PEI–AuNPs–MnIIIPPIX was prepared by an amidation reaction between carboxyl groups of porphyrin and the amino groups of PEI–AuNPs. Thus, MnIIIPPIX is heterogenized and protected from forming dimer, which was supposed to be one of the most important deactivation reasons for metal porphyrin [18].

Preparation route of the PEI–AuNPs–MnIIIPPIX nanocomposite.
The prepared catalyst was in a stable colloidal state in water and was characterized using UV–vis spectroscopy and FTIR (Fig. 1).
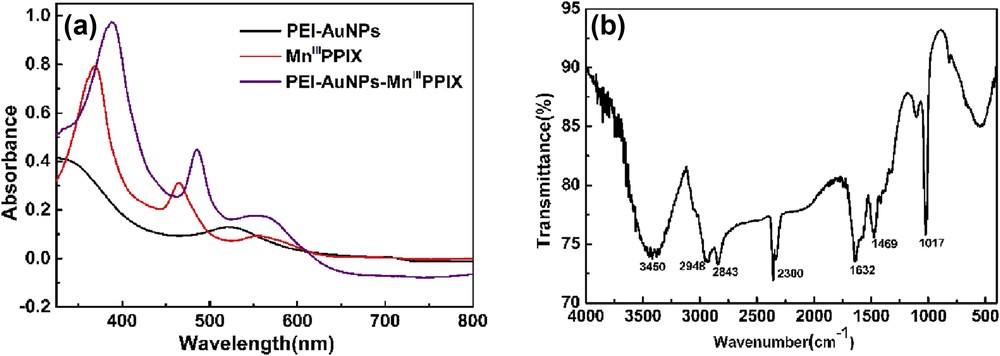
(a) UV–vis absorption spectra of PEI–AuNPs, MnIIIPPIX, and PEI–AuNPs–MnIIIPPIX nanocomposites; (b) FTIR spectrum of the PEI–AuNPs–MnIIIPPIX nanocomposite.
The UV–vis spectrum of MnIIIPPIX shows three absorption peaks at 370, 464, and 554 nm, respectively. Compared to the spectra of PEI–AuNPs and MnIIIPPIX, all three peaks were red shifted, which suggests that the conjugation is formed in the PEI–AuNPs–MnIIIPPIX nanocomposite [37,38]. The calculated diameter of AuNPs was 9.6 nm according to Haiss's theory [39]. The FTIR spectrum of the PEI–AuNPs–MnIIIPPIX features several characteristic bands. The strong and broad band near 3450 cm−1 belongs to stretching vibration of NH from PEI. There are absorption peaks of stretching vibration of NH2+ at 2948, 2843, and 2300 cm−1. The peak at 1632 cm−1 can mainly be assigned to a CO stretching in carboxyl or amide groups. The peaks at 1469 and 1017 cm−1 are attributed to the NH deformation vibration and CN stretching vibration. The informative spectroscopic data confirmed the covalent anchoring of MnIIIPPIX and PEI.
The morphology of PEI–AuNPs–MnIIIPPIX was further characterized by TEM, and Fig. 2 presents its representative TEM image and the size distribution.

(a) TEM image of the PEI–AuNPs–MnIIIPPIX nanocomposite. (b) The size distribution histogram of the immobilized AuNPs.
As shown in Fig. 2, the PEI–AuNPs–MnIIIPPIX had an approximately spherical morphology and dispersed uniformly with the average diameter of 9.4 nm (200 particles counted), and this matched well with the calculated results mentioned previously.
3.2 Peroxidase-like activities
To examine the catalytic activity of as-prepared PEI–AuNPs–MnIIIPPIX, we chose AO7, a typical azo dye as a probe of organic pollutants, which can be degraded by native peroxidase [40,41]. Fig. 3 shows the spectral changes of AO7 in different systems.
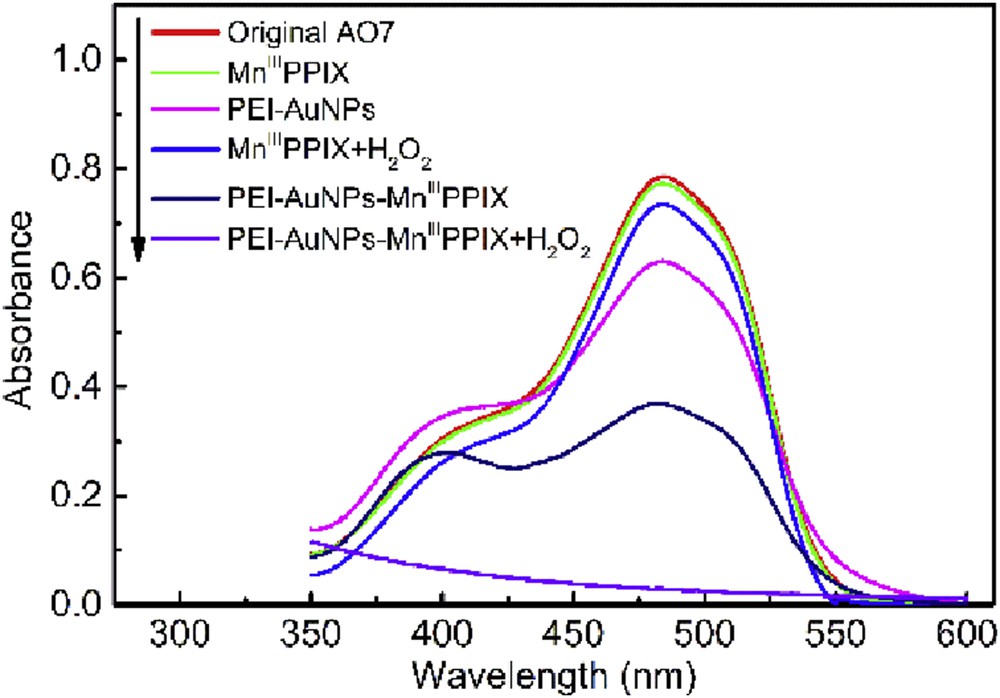
UV–vis spectra of AO7 and its change in different systems.
No obvious change of AO7 spectra is observed when MnIIIPPIX is present. The presence of PEI–AuNPs and PEI–AuNPs–MnIIIPPIX induced a decrease in the absorbance at 485 nm, which could be due to the adsorption of AO7 on the nanocomposite. It is interesting that there was an obvious decrease when PEI–AuNPs–MnIIIPPIX was added, indicating that the combination of MnIIIPPIX and PEI–AuNPs played a crucial role. The presence of H2O2 enhanced the AO7 degradation catalyzed by MnIIIPPIX and PEI–AuNPs–MnIIIPPIX and the most thorough degradation of AO7 achieved in the PEI–AuNPs–MnIIIPPIX/H2O2 system. The results prove the remarkable activity of the novel PEI–AuNPs–MnIIIPPIX nanocomposite on the activation of H2O2.
3.3 Factors affecting the degradation of AO7 in the PEI–AuNPs–MnIIIPPIX/H2O2 system
3.3.1 Catalyst dosage
Fig. 4 presents the degradation profile at different catalyst dosages ranging from 0.8 to 4.0 mg/L. As shown in Fig. 4a, the profile of normalized concentrations with time follows an exponential pattern, which may describe the degradation of AO7 according to the pseudo-first-order kinetic reaction:
(1) |
(2) |

(a) Effect of catalyst dosage on the degradation profile of AO7 and (b) the pseudo-first-order rate constant (AO 24 mg/L, pH 2.0, H2O2 1.5 mM).
The kobs increased significantly as a function of the dosage from 0.8 to 2.4 mg/L (Fig. 4b). Such enhancement in degradation was ascribed to the increased number of active catalytic sites, which are responsible for the formation of active radicals. Further increase in dosage (>3.2 mg/L) does not increase the kobs obviously, which may be limited by the rate-limiting step of the cleavage of the OO or OH bond in the intermediate where H2O2 is coordinated to the catalyst [4].
3.3.2 pH value
pH is another important parameter in the peroxidase-like catalytic reaction. Fig. 5 shows the degradation profile of AO7 and kobs between pH 1.0 and 9.0 and the maximum kobs was achieved at pH 2.0. pH value affects the structure of azo dyes, and hydrozone is the main form in the acidic medium with more susceptibility to oxidation [42]. On the other hand, pH plays an important role in the transformation of H2O2 coordinated to the metal porphyrin, which is consequently converted to an active intermediate [12,43].

(a) Effect of pH on the degradation profile of AO7 and (b) the pseudo-first-order reaction rate constant (AO 24 mg/L, PEI–AuNPs–MnIIIPPIX 2.4 mg/L, H2O2 1.5 mM).
3.3.3 H2O2 concentration
Fig. 6 depicts the degradation profile of AO7 and kobs at different H2O2 concentrations.
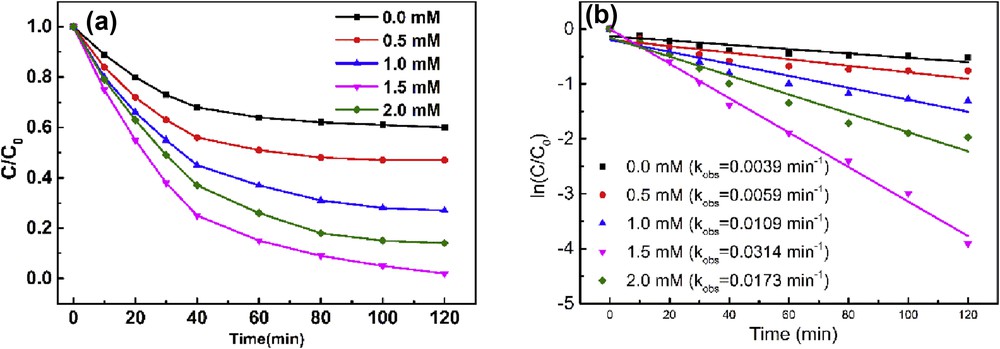
(a) Effect of H2O2 concentration on the degradation profile of AO7 and (b) pseudo-first-order rate constant (AO 24 mg/L, PEI–AuNPs–MnIIIPPIX 2.4 mg/L, pH 2.0).
As the H2O2 concentration increased to 1.5 mM, nearly complete degradation of AO7 was achieved within 120 min. However, the degradation ratio and reaction rate decreased when the H2O2 concentration increased to 2.0 mM. Suppose AO7 is completely mineralized according to the reaction:
C16H11N2O4S− + 42H2O2 → 16CO2 + 2NO3− + SO42− + 3H+ + 46H2O |
3.4 Possible mechanism
In Mn porphyrin–catalyzed system, the homolysis of OO in Mn peroxide with generation of OH radicals [4,8,10,12,13,45] supposed as to the main pathway (see Scheme 2(A)). In the present study, tert-butyl alcohol (TBA) [46] and methyl alcohol (MeOH) [47] are selected as the scavengers. Fig. 7 proves the obvious role of OH radicals during the degradation of AO7. The degradation ratios decreased from 97% to 33% and 45%, respectively, in the presence of TBA and MeOH and the kobs decreased 10.2% and 15.0% correspondingly. The results show that degradation of AO7 associated with the generation of OH radicals catalyzed by PEI–AuNPs–MnIIIPPIX is one important possible pathway. By comparing the inhibition effects of OH scavengers on the degradation of azo dyes to that in typical OH radicals' driving process [47], it is concluded that degradation of AO7 in the PEI–AuNPs–MnIIIPPIX/H2O2 system is not merely driven by OH radicals.

Possible reaction pathways in the activation of H2O2 by PEI–AuNPs–MnIIIPPIX.

(a) Effect of scavengers of OH radicals on the degradation profile of AO7 and (b) pseudo-first-order rate constant (AO 24 mg/L, PEI–AuNPs–MnIIIPPIX 2.4 mg/L, pH 2.0, H2O2 1.5 mM).
The homolysis of OO with generation of OH radicals was thought to cause the lower reactivity of Mn porphyrin [13]. Many efforts have been made to enhance the activity of MnIII porphyrin by adjusting the microenvironment of MnIII in porphyrin [2,13,48–50]. Here, PEI–AuNPs are induced into the system to improve the catalytic activity. Concerning the catalytic system and the results of scavenging OH radicals, we proposed the enhancing mechanisms of MnIII porphyrin activity in the PEI–AuNPs–MnIIIPPIX/H2O2 system. The possible reaction pathways for the activation of H2O2 by PEI–AuNPs–MnIIIPPIX can be described as presented in Scheme 2 (B) and (C).
First, the presence of the carboxyl group in MnIIIPPIX affords a suitable tail for the coordination and activation of H2O2 due to the formation of an acyperoxy-MnIII intermediate, in which the heterolytic cleavage of the OO bond is favored by the good leaving of the carboxylate [6,14]. Thus, more active high-valent metal-oxo species are produced, which are responsible for oxygen transfer to the substrate, AO7 (see Scheme 2(B)).
Second, the rich nitrogen atoms in PEI backbone, the nitrogen base, have cocatalytic effects. The similar cocatalytic effects of nitrogen bases have been reported in the catalytic reaction by MnIII porphyrins [4,15,17,50]. We refer that nitrogen atoms in PET could form the hydrogen bond with H2O2, which may facilitate the activation of H2O2 as a base to promote the OO bond cleavage, thus favoring the formation of the active catalytic species (see Scheme 2(B)). At the same time, the high density of amino groups in PEI renders the hydrophilic character of the nanocomposite. This hydrolytic stability will enhance the similarity with the natural environment of proteins and may lead to the use of practical heterogeneous catalyst in aqueous media [5].
Among the active species, MnIV species is less active and can revert to the active forms by the action of appropriate additives that induce the redox perturbation such as reduction or disproportionation [2,21,48,49], and the gold surface has been reported to have inducibility [21,49]. In PEI–AuNPs–MnIIIPPIX/H2O2 system, H2O2 can be activated through weak Au⋯O interaction with the gold surface. For MnIIIPPIX, the linkage between Mn–oxo to the AuNPs surface is formed and the reactivation of MnIV species to MnV species is facilitated by the linked AuNPs because of the more efficient route for electron transfer at the AuNPs surfaces (see Scheme 2(C)).
In this proposed mechanism, the excess H2O2 reacts with the Mn–oxo nanocomposite to produce the molecule of oxygen and water as shown in Scheme 2(D). The excess H2O2 also reacts with OH radicals to produce OOH with lower oxidation potential than that of OH:
H2O2 + OH → H2O + OOH |
This is why H2O2 concentration required is rather low in the PEI–AuNPs–MnIIIPPIX/H2O2 system, which is proved by the experiments as shown in Fig. 7.
4 Conclusion
In this work, the novel PEI–AuNPs–MnIIIPPIX nanocomposite with remarkable peroxidase-like activity in aqueous media was prepared under mild conditions. The nanocomposite showed high hydrolytic stability and the degradation of azo dye AO7 experiments demonstrated that the nanocomposite expresses high peroxidase-like activity in the presence of low concentration of H2O2. Our results in this study are one step forward in the development of a new trinary of peroxidase mimics with high activity in water. We proposed the coupling mechanism on the nature of MnIIIPPIX, PEI, and AuNPs involving the formation of active radicals: (1) the typical homolysis of OO in Mn peroxide catalyzed by Mn porphyrin with generation of OH radicals; (2) the carboxyl group in MnIIIPPIX affords a suitable tail for the coordination and activation of H2O2 due to the formation of an acyperoxy-MnIII intermediate, favoring the heterolytic cleavage of the OO bond; (3) the nitrogen atom in PEI backbone served as a base to promote the OO bond cleavage by forming a hydrogen bond with H2O2; and (4) AuNPs facilitate the reactivation of MnIV species to MnV species because of the more efficient route for electron transfer at the AuNPs surfaces. The construction of the novel catalyst PEI–AuNPs–MnIIIPPIX offers a new insight into the application of Mn porphyrin upon activation of H2O2, which can induce many further reactions.
Acknowledgments
This work was supported by the National Natural Science Foundation of China (21575066).