1 Introduction
This presentation is an overview of 40 years of research on a special class of materials that have been developed because they present a high optical transparency in the mid-infrared (mid-IR) range. These materials are glasses, a very unusual state of matter. This research has led to the creation of four companies, still alive.
First of all, we have never brought our interest to the family of oxide glasses such as silica. They dominate the world of optics because of their unique and outstanding properties. They cover the spectral domain of the visible light from the UV to the red, where our retina is sensitive. In addition, they are an excellent glass former leading to strong materials resistant to crystallization and corrosion.
SiO2 glasses have only one intrinsic disadvantage, they are opaque beyond 3 μm. This limit of transparency is because of the high vibration mode of the SiO chemical bond.
To develop optics transmitting light beyond 3 μm it is necessary to invent new compositions, which exhibit weaker chemical bond and are made of heavier atoms. Only two families of materials have been successfully developed giving rise to practical applications: fluoride and chalcogenide glasses.
1.1 What are the criteria to obtain a material in a vitreous state?
First of all, it must have a polymeric character, with an easy change in the bonds angle, to melt in giving a viscous liquid. This association of atoms by covalent bonding to form a giant molecule could by realized by combining dominant glass-forming atoms together with some others having the same electronegativity.
In many cases, the polymeric specie could be also a giant anion. The negative charge is compensated by some large cations distributed statistically in the viscous liquid. These large cations are called glass modifiers.
1.2 How to form a glass with a combination of atoms?
It is very instructive to look first into the crystal chemistry of these atoms. Let us take the case of silica, which exists in many polymorphic forms, from cristobalite to quartz. They only differ by the value and the variety of the SiOSi angle and are formed by corner-sharing SiO4 tetrahedra. It is not surprising that when silica melts, the atoms do not choose for a given orientation and keep the disordered situation of the liquid state. The situation is almost the same when playing with more exotic materials such as the fluoride glasses or the chalcogen-based glasses. All of us have learnt at school that when you cool down a liquid, at a certain temperature, it transform into a crystalline solid. This is true for the large majority of the liquids. Therefore, some of them refuse to follow this thermodynamic law; on the contrary, they become more and more viscous until the viscosity becomes infinite. The solid obtained is a frozen liquid, in other word, a glass. In case of the exotic compositions, the cooling needs to be fast enough to avoid any arrangement of atoms, which will nucleate a crystallite. We must admit that this temperature quenching procedure is very empirical and is one of the secrets of the glassmaker.
At the end, the glass obtained is a frozen liquid or a liquid with an infinite viscosity. In this sense it shows a continuous homogeneous development of the chemical bond and is an exceptional material for transmitting light. Nothing will interrupt the propagation of light, except in the UV–visible, the intrinsic absorption due to interaction with the bonding electrons and in the IR region the interactions with the phonon of the matrix.
The piece of glass obtained possesses unique properties compared to other solids. It can be heated until a special temperature called glass transition temperature (Tg). Above Tg the glass remembers that previously it was a liquid and becomes a plastic solid whose viscosity changes more or less rapidly with T.
This change in the viscosity regime is unique and extraordinary and allows the shaping of the glass by moulding eventually with the assistance of pressure.
The other most important property is the fibreing of a preform of glass, which permits its transformation into flexible fibres that can carry light according to the transparency domain of the vitreous material.
Because the glass is an out-of-equilibrium solid, there is some risk of nanocrystal nucleation, which phase separates from the glass matrix. A piece of glass heated above Tg, is then a solid in danger, and crystallization even partial could be detrimental for the propagation of photons. Fig. 1 represents the energy landscape of a glass. This disordered out-of-equilibrium solid is easily transformed into an ordered crystal.
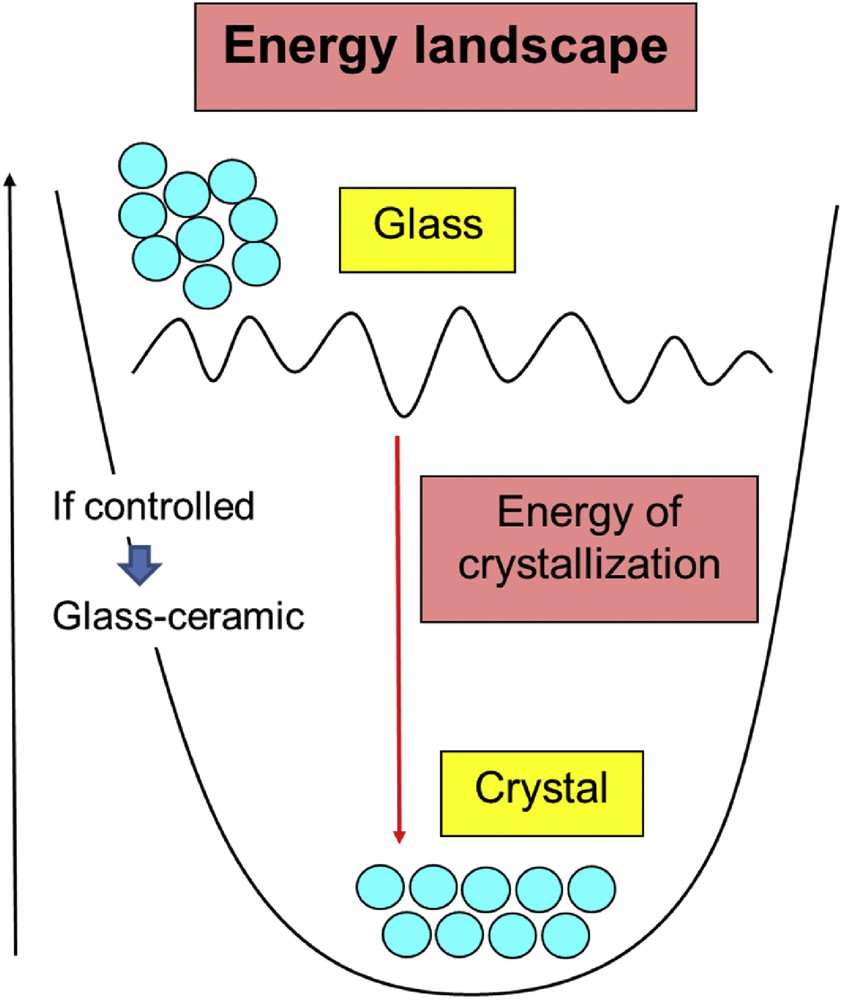
Energy landscape of a glass.
This phenomenon is the most critical and can be detected by differential scanning calorimetry, in measuring Tx the crystallization temperature. The difference Tx − Tg is a good criterion to estimate the shaping of a glass. It must be noticed that the growth of the nanocrystals can be controlled. If they keep a submicron size they are not scattering light in the IR and are not an obstacle to the transmission of IR photons. They lead to a new family of composite materials, the IR glass ceramics.
2 Search of ultratransparent glasses
This research was very active in the 1980s, and the main players were the optical fibre telecommunication companies. The idea was to find a substitute to silicate glasses for carrying information into an optical network over a longer distance. The glass SiO2 has indeed an intrinsic limitation in transparency, mainly due to two factors, which limit the choice of the photon wavelength. If the light is too close to the UV, it will be absorbed by the bonding electrons. On the other end an IR light will be absorbed by the vibration mode of the SiO network.
The result is a V-shaped attenuation curve giving the exact value of the optical loss versus wavelength. This is illustrated in Fig. 2, which shows that the minimum attenuation is 0.16 dB/km at λ = 1.55 μm for SiO2 fibres. This means that if such an optical signal is injected into the fibre it will keep about 20% of its strength after travelling 80 km into the fibre.
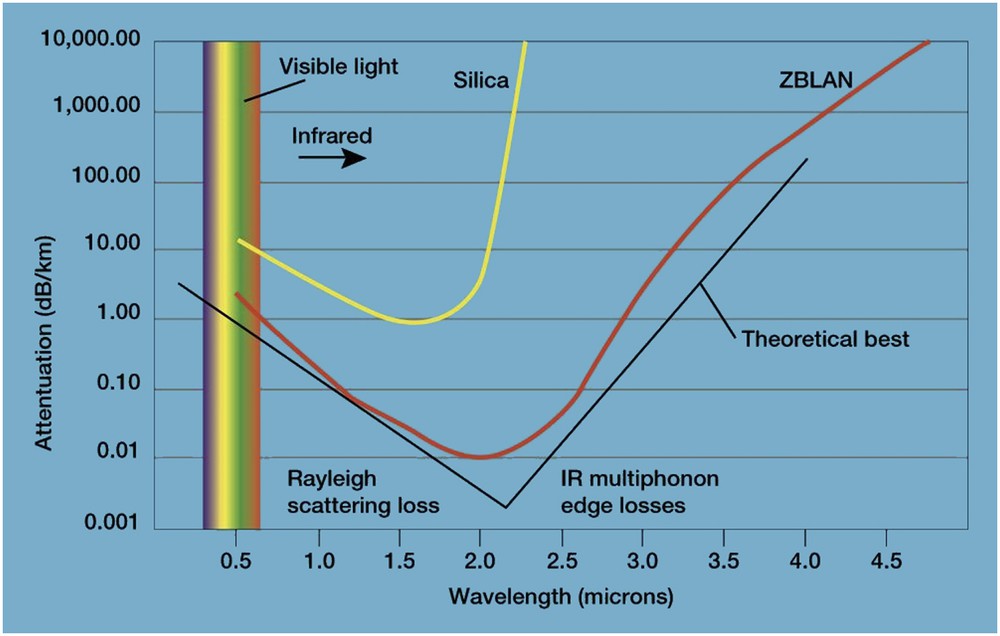
Silica and fluoride glass fibre attenuation curves versus wavelength.
An alternative to silica fibre was the fluoride glasses based on the glass forming properties of ZrF4 [1]. The situation is almost the same in the UV part, but is quite different on the IR side. The vibration mode of ZrF is much lower than that of SiO, and it results in the V-shaped curve for the complex ZrF4 glasses called ZBLAN, which is much deeper and correspond to a minimum loss of about hundred times lower than with SiO2 [2]. This ultratransparency occurs at a longer wavelength λ = 2.5 μm. This means that repeaterless optical fibre communication was possible over several hundred of kilometres.
This goal was never reached because impurities such as transition metals and OH even at a ppb level were conflicting with the intrinsic theoretical transparency.
But still the ultratransparency of fluoride remains. Good luck for the next generation of researchers in charge of ultrapure materials. In the same period, the SiO2 fibres made a tremendous progress because of the invention of the chemical vapour deposition process, which permits to reach industrially the ultimate transparency of silica.
Nevertheless, it remains a small business for fluoride glasses, and the company Le VerreFluoré, the first company created from the results of the laboratory, illustrated that transparent fibres from the UV to the mid-IR are always needed. There is some niche market in astronomy.
The complex composition of 60ZrF4, 20BaF2, 4LaF3, 6AlF3 and 10NaF, whose acronym is ZBLAN, is the most stable versus crystallization and is suitable for fibre drawing [2]. The glass-forming polyhedra are ZrF7, AlF6 and LaF8, whereas Ba2+ and Na+ play the role of modifiers. This kind of glass contains rare earth (RE) as natural constituent and then can be doped by all the RE elements. This has led to the development of optical amplifiers or fibre lasers showing more emission lines than silica glass [3].
3 Search of glasses transmitting as far as possible in the IR
The only elements that show potentiality for practical applications belong to the group VI. Indeed the chalcogens S, Se and Te associated with neighbouring atoms are heavy enough to transmit IR light [4]. They also exhibit some interesting glass-forming properties and good chemical durability.
The element Se is a good example explaining the formation of glass. On the six outer electrons two of them are bonding, forming infinite chains by strong σ covalence. The four others are nonbonding and form two lone pairs. The result is a formation of long flexible chains made of corner-sharing tetrahedra. Each tetrahedron is made of two localized lone pairs and two σ bonds. One can understand that free rotation around the σ bond is responsible for the flexibility of the structural model, and that when melted, Se can choose many equivalent possibilities of orientation. When cooling down Se vitrifies easily.
For making practical objects, glasses need to have suitable mechanical properties, which are related to the dimensionality of the framework. In particular, for practical reasons, Tg needs to be high enough. The way to improve the dimensionality is realized by cross-linking the chains, by trivalent atom such as As or Sb and using tetravalent atom such as Ge. The large glass rod in Fig. 3 has a typical Se/As/Ge composition. It transmits light from 1 to 15 μm.

Se/As/Ge glass rod.
The element Te, which has the same structure as Se, is not forming glass by itself. Indeed the lone pair electrons are, in this heavy element, very delocalized and form a metallic π bond along the chains. This situation avoids any free rotation. We succeeded to eliminate this π bonding in grafting halogens X = Cl, Br, I to the Te chains; the role of the halogen is to capture those free electrons. Then, were born the TeX glasses, which show a transparency towards 20 μm [5]. Almost the same results were obtained in connecting the Te chains with Ge atoms or better in adding to the melt lone pair deficient atoms such as Ga, which play the role of trapping the mobile electrons of Te.
The number of mixed compositions such as Te/Se/As, also called the TAS glass, has been optimized to develop optical fibres for medical and biological applications [6].
These chalcogen-based glasses despite their low phonon energy have never been candidates for telecommunications. Indeed they exhibit a poor transmission in the UV and visible light. Most of the Se or Te glasses are black. The absorption of the visible light is due to the nonbonding lone pair electrons, which made an intermediate level between the bonding and antibonding levels. The band gap of the glasses decreases from the S to Se and to Te. It must be noticed that some S-based glasses have partial transmission in the visible light. They are used as a matrix for RE elements, when a pumping level is possible and the emission is then collected in the IR [7].
Fig. 4 represents the transmission range of the different IR glasses.
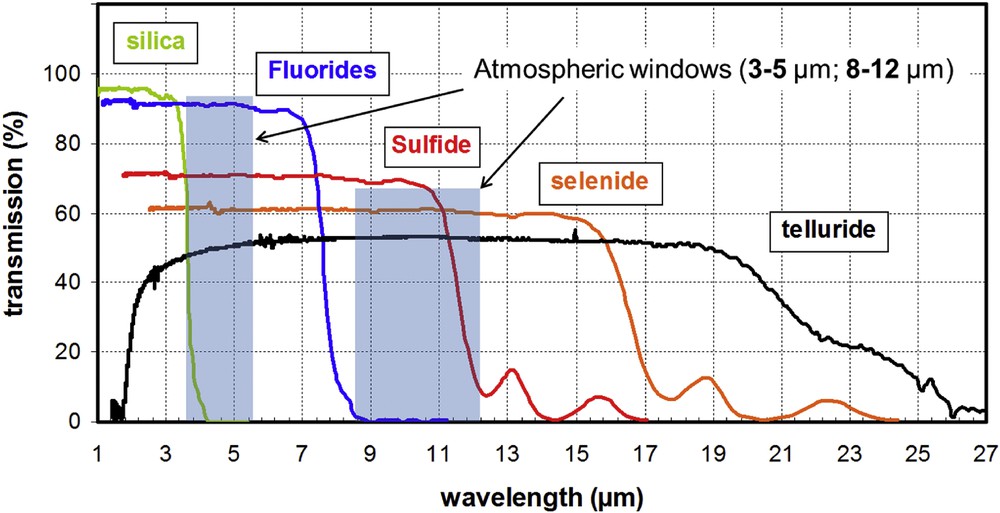
Transmission range of different IR glasses.
4 Moulded optics for night vision systems
As indicated in Fig. 4, the Se or Te glasses cover very important optical domains, which are called the atmospheric windows. Indeed the atmosphere that contains mainly H2O vapour and CO2 blocks the IR radiation, respectively, around 7 and 14 μm. The result is the existence of two windows called the 3–5 μm and the largest 8–12 μm, which are transparent enough to let the IR radiation go through.
Also, thermal objects emit IR radiation according to the black-body law. Fig. 5 represents the emission curve as a function of temperature. As represented in Fig. 5, an object (also humans at normal body temperature) at room temperature emits most of its energy around a wavelength of 10 μm. It must be noticed that the emission power is about 1 W/kg. This kind of radiation can be captured by an optical system made of Se glass.

Black-body spectral emission versus wavelength at different temperatures.
The huge advantage of Se glass compared to other IR crystalline materials, such as single crystal germanium, resides in their cost and the possibility of moulding above Tg. We first demonstrated the moulding capability of the chalcogen-based glasses [8]. This was at the origin of the company Vertex, which is now Umicore IR glass and located close to the laboratory. This company produces IR optics moulding glasses of different shapes and optical configurations. They provide lenses to the different IR camera producers. The low cost lenses have made the thermal imaging system affordable. Fig. 6 illustrates the moulding process and shows some lenses. Also shown is the first IR camera produced under defence contract by Thalès. It was manufactured more than 20 years ago and is equipped with moulding aspherical and asphero-diffractive moulded Se glass.

(a) Moulding process, (b) moulded chalcogenide lens, and (c) first IR camera equipped with chalcogenide glass optics (Thales ELVIR).
Now Umicore produces a great number of different optics from the large lenses for military applications to the small for thermal imaging and heat detectors.
In Fig. 7 are represented some domains of applications for IR cameras, from car driving assistance in the dark, medical imaging, to heat detection in building and all types of industries.
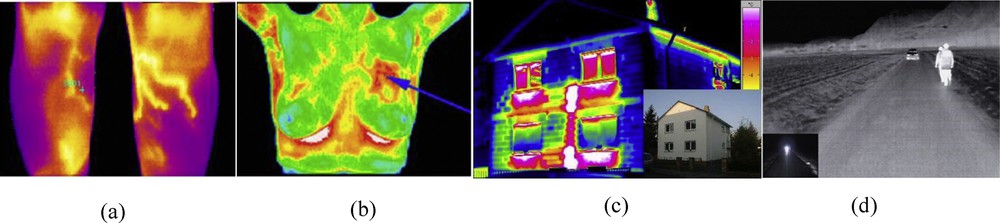
IR camera images: (a and b) medical imaging, (c) heat dissipation in house, and (d) night vision and car driving assistance.
5 IR fibres for the detection of molecules
The superiority of the glassy state resides on the possibility to draw optical fibre by heating a glass rod preform above Tg to reach the suitable viscosity and avoiding the nucleation of crystallites. In addition, the glass needs to have the maximum transparency. We have developed a special composition called TAS glass with the formula Te20As30Se50, which contains the maximum of Te. This is a good compromise for enlarging the IR transparency while keeping an excellent durability and having good mechanical properties.
These fibres transmit light from 2 to 12 μm and have an average diameter of 200 μm. They are used for the detection of molecules, according to the fibre-optic evanescent wave spectroscopy principle. When IR light is injected into a fibre, a part of the energy, because of multiple reflections on the surface of the fibre, escapes and propagates on the skin of the fibre. This evanescent light is more important when the fibre diameter decreases.
Any kind of molecule put into contact with the fibre will absorb the IR light according to its own vibration modes. The principle of measurement is represented in Fig. 8.
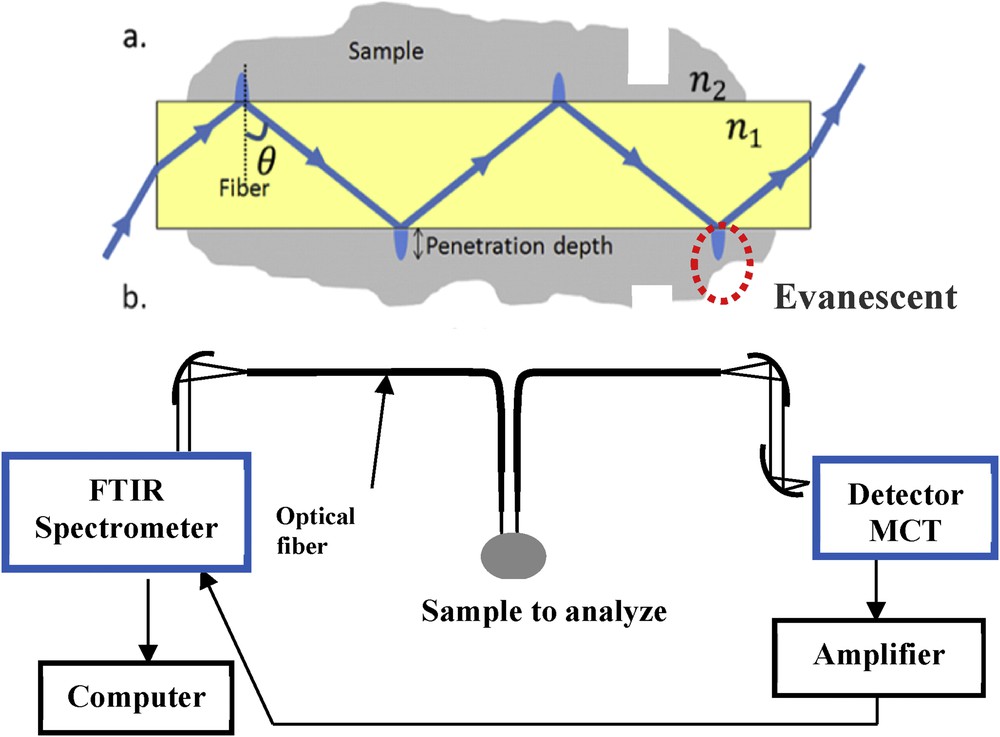
(a) Principle of fibre evanescent wave absorption and (b) experimental set-up.
This is an elegant way to realize in situ IR spectroscopy [9]. This method has been applied in many domains of biology and medicine. To develop a practical instrumentation we have created a company called Diafir (Diagnosis by IR fibre). This company put on the market a compact spectrometer equipped with a small cell containing a short IR fibre. Just one drop of liquid is enough to collect the IR spectrum of the liquid. Diafir is working with many hospitals and has been able to provide a fast and noninvasive method for analysing suspicious biological liquids.
In the laboratory, we have used the fibre evanescent wave spectroscopy technique in many domains. In medicine, we have worked on the detection of liver diseases (cirrhosis, hepatosiderosis, cancer, etc.) [10,11].
In the food industry, we have been able to detect and identify bacteria (staphylococcus, listeria, salmonella, etc.) in several food products (milk, cheese, meat, etc.)
In the industry and environment, we succeeded in monitoring the cooking of a resin for Airbus and the detection of phycotoxins in the sea.
Recently, the development of new optical fibres containing high amounts of Te has permitted to enlarge the domain of transmission towards the longer wavelengths. These sensors are able to detect the vibration bands of molecules considered as emerging pollutants, like the CCl band of chloroform [12]. Applications in the environment domain could be developed, especially the monitoring of water quality.
6 Microstructured fibres: a new way for guiding the IR light
The traditional way to guide the light into an optical fibre is to use a two-index configuration. The core of the guide is made of a glass having a refractive index slightly superior to the clad. By this way, the IR light due to reflection at the core/clad interface stay and propagate inside the core of the fibre.
An original way to obtain single-mode fibres is to design microstructured optical fibres (MOFs). These fibres present unique optical properties, thanks to the high degree of freedom for designing the geometrical structure. Since the primary works on silica MOFs [13], this new class of fibres has attracted much interest in the optical fibre community. In this case, the light propagation is obtained in the high refractive index core surrounded by the several crowns of low refractive index air holes. Ten years later, MOFs were realized with chalcogenide glasses [14].
A classical method to make MOFs is the stack and draw technique. However, this technique induces high optical losses (several dB/m) in chalcogenide glass MOF because of the poor quality of interfaces between chalcogenide capillaries. A new casting method to fabricate chalcogenide preforms has been developed [15]. The methodology for elaborating the fibre consists in moulding a rather fluid liquid chalcogenide glass into a silica vessel, which contains aligned silica capillaries (Fig. 9). After controlling that the liquid has filled the empty space, the silica ampoule is quenched to room temperature.
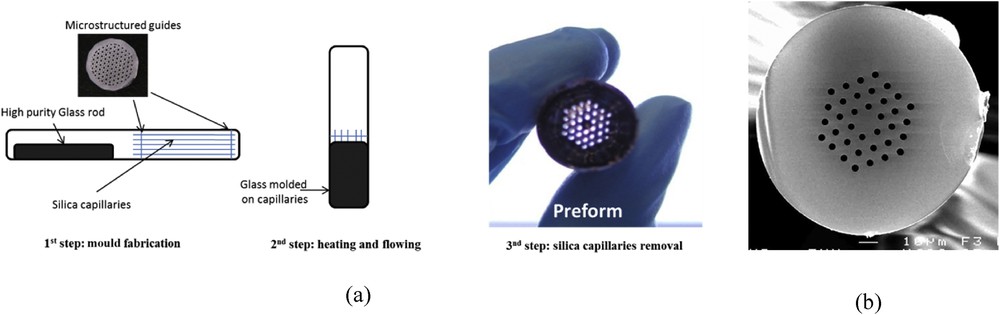
(a) Principle of the moulding method and (b) cross-section of a chalcogen-based MOF (courtesy of SelenOptics).
This operation is followed by the dissolution of the silica capillaries by a liquid HF treatment. After dissolution of the silica capillaries the resulting microstructured chalcogenide preform is drawn into fibre in a He controlled atmosphere. Typically, the fibre diameter can vary from 100 to 300 μm (Fig. 9). During the drawing step, the hole diameters are adjusted by applying a pressure into the preform holes. On the basis of this technology, we have created the SelenOptics Company and commercial chalcogenide MOFs are now available.
Low optical losses and innovative chalcogenide MOFs, such as small core fibres, present strong interest for wavelength conversion by using nonlinear effects such as four wave mixing, Raman and Brillouin scattering and supercontinuum generation [16]. These original types of fibres are also very suitable to observe evanescent spectroscopy in filling the hole by a gas to be analysed. They can be used for efficient mid-IR sensing [17].
7 Conclusions
This contribution is a sum of more than 40 years of research devoted to IR glasses. The results mentioned represent only those who have led to applications. Four companies Le VerreFluoré, Umicore IR Glass, Diafir and SelenOptics were born from this research. Those companies are leading in their domain and employ many students formed in the laboratory who kept close contact with it. All these companies were developed from pure fundamental discoveries made by our group. We were lucky to make a pioneering work in a field that just emerged about 40 years ago. The IR technology was, at the beginning, essentially developed for military applications, and thanks to the help of the French Defence we were able to develop IR lenses for dual technology.
Many others results have been obtained and published in domains that are more fundamental and concern, for instance, the structure of the glass and their thermal and mechanical properties. An important contribution was also made on the active properties of IR glasses. This part of the research concerns the RE–doped glasses, essentially the fluorides, which transmit in the pumping area of dopants. In the family of chalcogenides, only the S-doped glasses are enough transparent in the visible light to receive applications.