1 Introduction
Large amounts of agroindustrial wastes with no economic value are being produced every year. Vine shoots are an example of these byproducts usually left in the vineyard or used as a heating source [1,2]. Vine shoots are produced in large quantities because they consist of 93% of the total viticulture waste [3]. Many previous works aiming at the valorization of vine shoots proposed the production of paper pulp, of a lower quality, however, than the one produced from other agricultural residues such as wheat straw [4]. Energetic exploitation of vine shoots was also investigated by producing solid biofuels [2]. Nonetheless, the extraction of polyphenols, activated carbon, smoke flavors, and other beneficial compounds has also been widely studied [4,5]. Vine shoots are mainly composed of two fractions: 68% holocellulose (cellulose and hemicellulose) and 20% of lignin [1]. Cellulose is a regular glucose polymer consisting of d-glucose units connected by β (1 → 4) bonds. Hemicellulose is a heteropolymer consisting of five major monomeric sugars: l-arabinose, d-glucose, d-xylose, d-mannose, and d-galactose. Lignin is a high molecular weight polymer composed of monolignols (aromatic alcohols). The complex three-dimensional structure results from the copolymerization of three aromatic monomers: p-coumaryl alcohol, coniferyl alcohol, and sinapyl alcohol [6]. The extraction or degradation of cellulose, hemicelluloses, and lignin, which are the main polymeric fractions of lignocellulose, is a primordial step for an integral exploitation of this resource [7]. Acid treatment (H2SO4) converts hemicelluloses into reducing sugars that can be further fermented by microorganisms like Lactobacillus rhamnosus into ethanol, xylitol, lactic acid, and biosurfactants. Enzymatic hydrolysis also results in the production of fermentable sugars from insoluble cellulose or hemicellulose. This has the advantage of avoiding the degradation of the product or the formation of microbial inhibitors [7,8]. In addition, enzymatic hydrolysis was efficient in terms of polyphenol extraction from different matrices [9,10]. The delignification process through alkaline hydrolysis isolates lignin [7] that can be used in dyes, paints, emulsifiers, and so forth. [11,12]. Physical pretreatments, such as pulsed electric fields, ultrasounds, and high voltage electrical discharges (HVED) proved to increase the efficiency in terms of polyphenol extraction from vine shoots [13]. HVED has proven its efficiency in terms of enhancing the enzymatic hydrolysis yields and improving the delignification process of rapeseed straw [14]. However, the multistep extraction of many bioactive molecules, including polyphenols, was not conducted before. The recovery of polyphenols from vine shoots is very important because it is a rich source of resveratrol (R). The latter has shown many health benefits in oxidative stress, inflammation, obesity, diabetes, hepatic metabolism, cardiovascular effects, and so forth. [15]. The scientific strategy of this article meets the sustainable development concept, because (1) waste is reused to extract bioactive molecules that have many industrial applications and (2) an innovative green technology is used (HVED), reducing energy consumption, time, and solvent use [16].
To the best of our knowledge, no previous studies have suggested combining HVED with the enzymatic hydrolysis to improve the extraction yield. To this end, the valorization of vine shoots was achieved in the present study by the selective multistep extraction of polyphenols, reducing sugars, and lignin. This work highlights the importance of a multistage process to avoid the unspecific recovery of biomolecules observed after a grinding process. The effect of HVED together with that of enzymatic hydrolysis on the saccharification process and polyphenol extraction was studied. Experimental data about the influence of an enzymatic hydrolysis combined with HVED pretreatment on the subsequent alkaline hydrolysis for the delignification process were also reported.
2 Materials and methods
2.1 Raw material
Vine shoots (Vitis vinifera vine shoots var. Grenache Blanc) (200 kg/m3) were cut into cylinders of 1 cm height and a mean diameter of 0.5 cm. The dry matter (DM) content of vine shoots was 91% and was measured by drying the raw material to a constant weight at 105 °C.
2.2 Extraction process
Three extraction protocols were compared: enzymatic hydrolysis, HVED-assisted enzymatic hydrolysis, and alkaline hydrolysis. All the extraction processes were conducted in a round incubator (Infors HT Aerotron, France) for 4 h (total duration) with an agitation rate of 160 rpm, at 50 °C with a liquid to solid ratio of 20 (15 g of vine shoots and 300 g of solvent).
A first step of HVED followed by enzymatic hydrolysis in the best conditions permitted the extraction of polyphenols and soluble sugars, respectively. Obtained extracts were analyzed for the determination of total polyphenols, reducing sugars, and soluble lignin contents. The remaining exhausted vine shoots were then dried at room temperature and subjected to a subsequent step of alkaline hydrolysis for delignification. All extracts were filtered on a filter paper with a retention size of 5–13 μm (VWR, France) and were stored at −20 °C for no more than 2 weeks before analysis.
2.2.1 Enzymatic hydrolysis
Viscozyme L is a multienzyme complex containing arabanase, cellulase, β-glucanase, hemicellulase, and xylanase from Aspergillus sp. Four different enzyme concentrations were studied: 4, 8, 16, and 24 fungal β-glucanase units (FBGUs) per gram of vine shoots. One FBGU is the amount of enzyme required to reduce 1 μmol glucose/min according to the standard conditions. The diffusion process was conducted in the conditions stated in Section 2.2, with a pH of 4.5 (adjusted with HCl solution) given by the supplier as the optimal conditions for enzyme activity. The pH of the solution did not change during the whole duration of the extraction process. The best enzyme concentration for the hydrolysis was then selected to be conducted before (Viscozyme then HVED), during (HVED + Viscozyme), and after the HVED treatment (HVED then Viscozyme). The enzymatic reaction was stopped by incubating the extracts for 20 min at 90 °C to deactivate the enzyme [17].
2.2.2 High voltage electrical discharges
The high-voltage generator (40 kV, 10 kA) (Tomsk Polytechnic University, Russia) provides pulses (10 μs duration) with a frequency of 0.5 Hz and an average energy of 160 J/pulse. The generator is connected to a processing chamber (1 L capacity) comprising a needle stainless steel electrode (10 mm diameter) spaced 5 mm from the plane electrode (35 mm diameter). Vine shoots (15 g) and preheated solvent (300 g water or enzyme + water) at 50 °C were introduced between the electrodes. The electric treatment consisted of applying 200 discharges resulting in a total energy input of 101.6 kJ/kg. The temperature of the mixture was controlled every 50 pulses using a type-K thermocouple stainless steel beaded probe of a Traceable Type-K Thermometer (Fisher Scientific, Illkirch-Graffenstaden, France) ensuring a temperature elevation of less than 2 °C.
2.2.3 Alkaline hydrolysis
Alkaline hydrolysis was conducted with different NaOH concentrations (0.1, 0.2, 0.5, and 1 M) (pH ≈ 13) at 50 °C for 4 h under the conditions stated in Section 2.2. The NaOH was neutralized with the addition of HCl. The best alkaline hydrolysis conditions were then chosen to be used on exhausted vine shoots.
2.3 Alkaline hydrolysis on exhausted residues
After the determination of the optimal NaOH molarity (Fig. 2), alkaline hydrolysis was conducted on exhausted residues of vine shoots all subjected to different pretreatments (enzymatic hydrolysis, alkaline hydrolysis, and HVED-assisted enzymatic hydrolysis) (Fig. 1). The extracts were analyzed for the determination of total phenolic compounds, and the Klason lignin (KL) was quantified in the remaining solid.
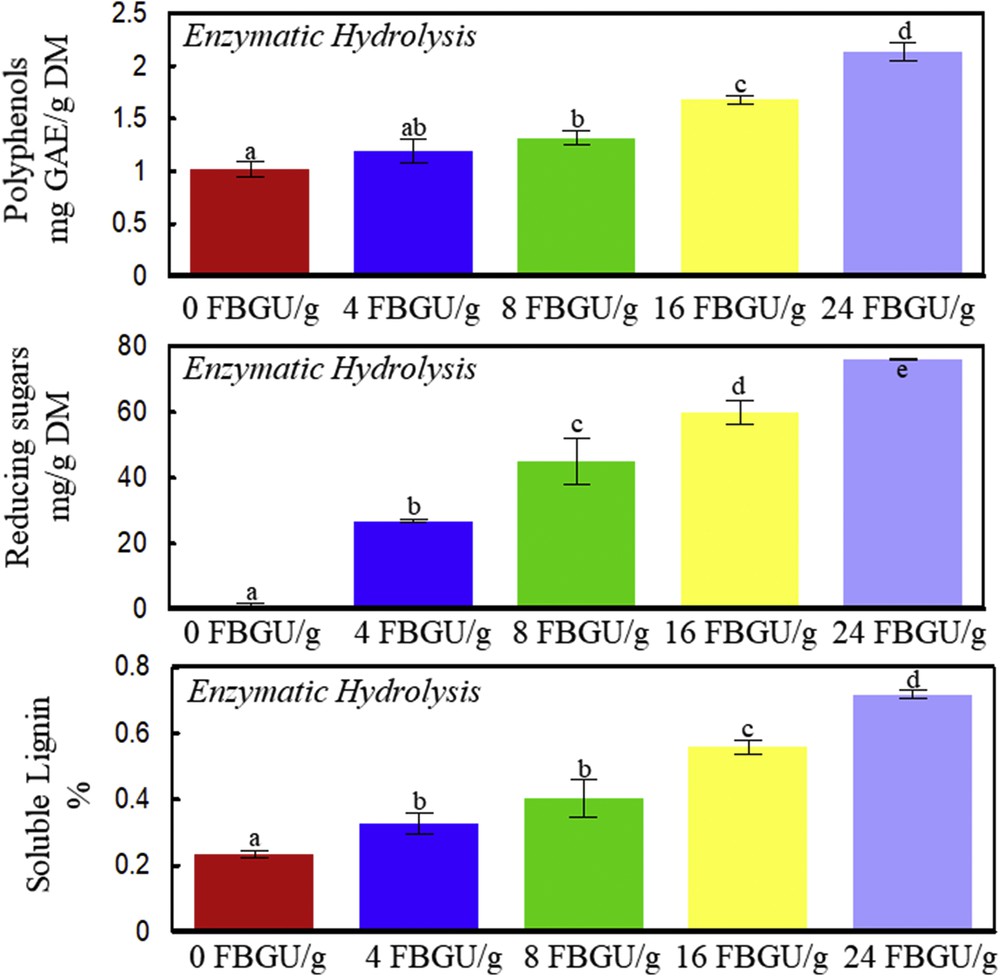
Enzymatic hydrolysis of vine shoots at 50 °C, pH 4.5, 160 rpm, and 4 h. Polyphenols, reducing sugars, and soluble lignin contents as a function of different Viscozyme L concentrations (0, 4, 8, 16, and 24 FBGUs/g vine shoots). A significant statistical difference between means is indicated by different letters (a, b, c, d, and e).

Multiple step process of polyphenol recovery, simple sugar extraction, and delignification.
2.4 Analysis
2.4.1 Total polyphenol content
On the basis of the oxidation/reduction reactions of phenols, the amount of total polyphenols was colorimetrically assayed by Folin–Ciocalteu [18]. A mixture of 0.2 mL of extract and 1 mL of Folin–Ciocalteu reagent (10-fold diluted) (Merck, Darmstadt, Germany) was prepared. After 5 min, 0.8 mL of Na2CO3 (75 g/L) (Prolabo, France) was added. The sample was incubated for 10 min at 60 °C and then cooled for 10 min at room temperature. The absorbance was measured at 750 nm using the UV–vis spectrophotometer (Thermo Spectronic Genesys 20, Thermo Electron Corporation, MA, USA). Gallic acid (Sigma–Aldrich, St-Quentin Fallavier, France) was used for the calibration curve. Results are expressed in milligrams of gallic acid equivalent per gram of DM (mg GAE/g DM).
2.4.2 Reducing sugar content
Reducing sugar amount was colorimetrically assayed by means of 3,5-dinitrosalicylic acid based on the oxidation of the aldehyde/ketone functional group present in sugars [19]. A mixture of 0.5 mL of extract and 1 mL of 3,5-dinitrosalicylic acid reagent (Sigma–Aldrich) was prepared. The sample was incubated for 5 min at 100 °C and then for 4 min at −20 °C. d-Glucose anhydrous (Fisher scientific) was used for the calibration curve. Results are expressed in mg/g DM.
2.4.3 Soluble lignin
The soluble lignin was assayed by measuring the absorbance in the UV range at 205 nm [20]. The concentration of soluble lignin (% SL) is calculated as follows:
(1) |
2.4.4 Klason lignin
KL content was determined according to TAPPI T222 om-11 [21]. Exhausted vine shoot samples (0.175 g) were mixed with 1.5 mL of sulfuric acid (72%) (Chem Lab nv, Zedelgem, Belgium) and incubated at 30 °C for an hour and 30 min. Then 42 mL of distilled water were added to dilute the samples. After that ,an autoclaving of 1 h at 122 °C was conducted. The masses of the solid residues after the drying process at 105 °C were those of the KL.
2.4.5 Relative proportion of polyphenols and reducing sugars toward one another
The measured total polyphenol content (TPC) and reducing sugar content (RSC) allowed the calculation of the relative proportions of polyphenols and reducing sugars toward each other. It was estimated as follows:
(2) |
The relative proportion was a criterion for the evaluation of the efficiency of the treatments.
2.4.6 Polyphenol identification and quantification by high-performance liquid chromatography
High-performance liquid chromatography (HPLC) analyses were performed to characterize and quantify phenolic compounds from vine shoot extracts [5,22]. The HPLC system (Waters Alliance, Milford, MA) was equipped with a quaternary Waters e2695 pump. Spectrophotometric detection was performed at 280 nm using a UV−vis photodiode array spectrophotometer Waters 2998 coupled with the control system and data collection Empower 3 software. Separations were achieved on a Discovery C18, 5 μm, 250 × 4.6 mm, column (Supleco, Bellefonte, PA) at 30 °C, with a C18, Supelguard Discovery 18, 20 × 4 mm, 5 μm, precolumn (Supelco). The mobile phase consisted of solvent A (0.1% (v/v) of aqueous formic acid) and solvent B (100% of acetonitrile). The solvent gradient was carried out as follows: 15–20% B for 5 min; 20–44.5% B for 45 min; 100% B for 1 min; maintained at 100% B for 9 min; and then 15% B for 5 min. The injection volume was 100 μL at a flow rate of 0.5 mL min−1.
Ferulic acid (FA), p-coumaric acid (CA), 4-hydroxybenzoic acid, and R were used for polyphenol quantification by the external standard method.
2.5 Statistical analyses
All experiments were conducted in triplicate. The means and error bars were represented in all the figures. Variance analyses (ANOVA) and least significant difference test were done by Statgraphics Centurion XV (StatPoint Technologies, Inc).
3 Results and discussion
3.1 Enzymatic hydrolysis
Fig. 2 shows the effect of enzymatic hydrolysis on total polyphenols, reducing sugars, and soluble lignin extraction from vine shoots. The increase in Viscozyme L concentrations up to 24 FBGUs/g significantly increases by twofold the polyphenol extraction from vine shoots as compared to water (P < 0.05). Carbohydrate-hydrolyzing enzymes (pectinase, cellulase, hemicellulase, and glucanase) are likely to release cell wall complex polyphenols, thus augmenting their extractability. Previous studies showed the efficiency of enzymatic hydrolysis on the extraction of bioactive molecules from plants [10]. More specifically, Viscozyme L was shown to be efficient for polyphenol extraction from unripe apples [9]. Viscozyme L (24 FBGUs/g) enhances the reducing sugar extraction up to 80 mg/g DM and the soluble lignin obtention up to three times as compared to water (Fig. 2). The relative proportion of reducing sugars toward polyphenols and reducing sugars augmented from 54 to 97% by adding 24 FBGUs/g of Viscozyme L to water. This specificity of the enzymatic saccharification and the biochemical conversion of the biomass into reducing sugars are likely to lead to a high efficiency of their subsequent fermentation into bioethanol.
3.2 Alkaline hydrolysis
Fig. 3 shows the influence of increasing NaOH concentrations on the extraction of polyphenols, reducing sugars, and soluble lignin from vine shoots. The highest polyphenol yield was obtained with the highest concentration of NaOH (1 M). The resulting polyphenols are either released by lignin hydrolysis [1] or liberated from cell walls by mild alkaline hydrolysis [23]. Vine shoot cell walls are characterized by the presence of phenolic acids that can be covalently linked to polysaccharides (ester bonds) and to lignin components (ester or ether bonds) [24]. Enzymatic and alkaline hydrolyses are thus likely to liberate polyphenols; however, NaOH addition (1 M) is five times more efficient than Viscozyme L (24 FBGUs/g). Around 6 mg/g of sugars are obtained with 1 M NaOH, but this result remains insignificant as compared to the 80 mg/g DM obtained with Viscozyme L (24 FBGUs/g). NaOH (1 M) at 50 °C dissolves lignin up to 8% (Fig. 3). In the literature, the delignification process was conducted with different alkalis (NH4OH, KOH, and NaOH) at different temperatures (50–130 °C) [7,25].
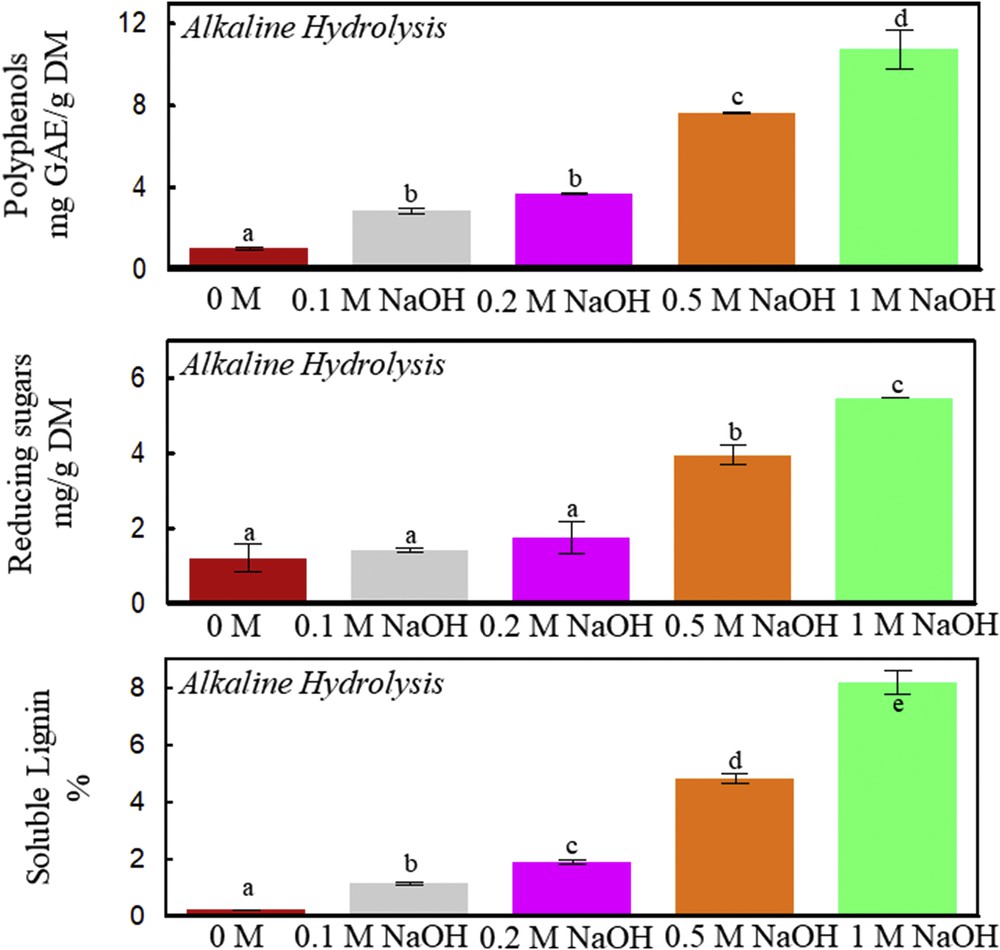
Alkaline hydrolysis of vine shoots at 50 °C, 160 rpm, and 4 h. Polyphenols, reducing sugars, and soluble lignin contents as a function of different NaOH concentrations (0, 0.1, 0.2, 0.5, and 1 M). A significant statistical difference between means is indicated by different letters (a, b, c, d, and e).
3.3 HVED-assisted enzymatic hydrolysis
The intensification of the extraction of polyphenols, reducing sugars, and soluble lignin was studied by combining the enzymatic hydrolysis with HVED. Fig. 4 shows that the HVED pretreatment of 101.6 kJ/kg significantly enhances the extraction of polyphenols, reducing sugars, and soluble lignin from vine shoots (P < 0.05). HVED induces electrical breakdown in water, bubble cavitation, and shock waves provoking structural damages to the treated product, thus enhancing the extraction of molecules from cell cytoplasm [26,27]. HVED treatment gives the highest observed relative proportion of polyphenols toward polyphenols and reducing sugars of 66.4%. This suggests that the physical treatment of HVED can be used as a first step to obtain high-concentration polyphenol extracts to be used for many industrial applications. The subsequent addition of Viscozyme L (24 FBGUs/g) gives extracts with a high relative proportion of simple sugars, which makes the combination of an electrical treatment (HVED) with a biological enzymatic hydrolysis, a selective method for reducing sugar recovery from vine shoots. HVED provokes vine shoot fragmentation [13], approximately 16% of damage degree [28], probably increasing the accessibility of carbohydrolytic enzymes to cellulose and hemicellulose, thus resulting in a better release of polyphenols (3.7 mg/g DM), reducing sugars (110 mg/g DM), and soluble lignin (1.5%). In this study, no enzymatic inactivation of proteins by HVED was observed, because the HVED + Viscozyme treatment gave a better yield of all the biomolecules as compared to the HVED treatment alone. The highest obtained RSC in this study was 11 g/100 g DM, whereas the cellulose and hemicellulose contents of vine shoots were reported to be 41.14 and 26 g/100 g, respectively [29,30].
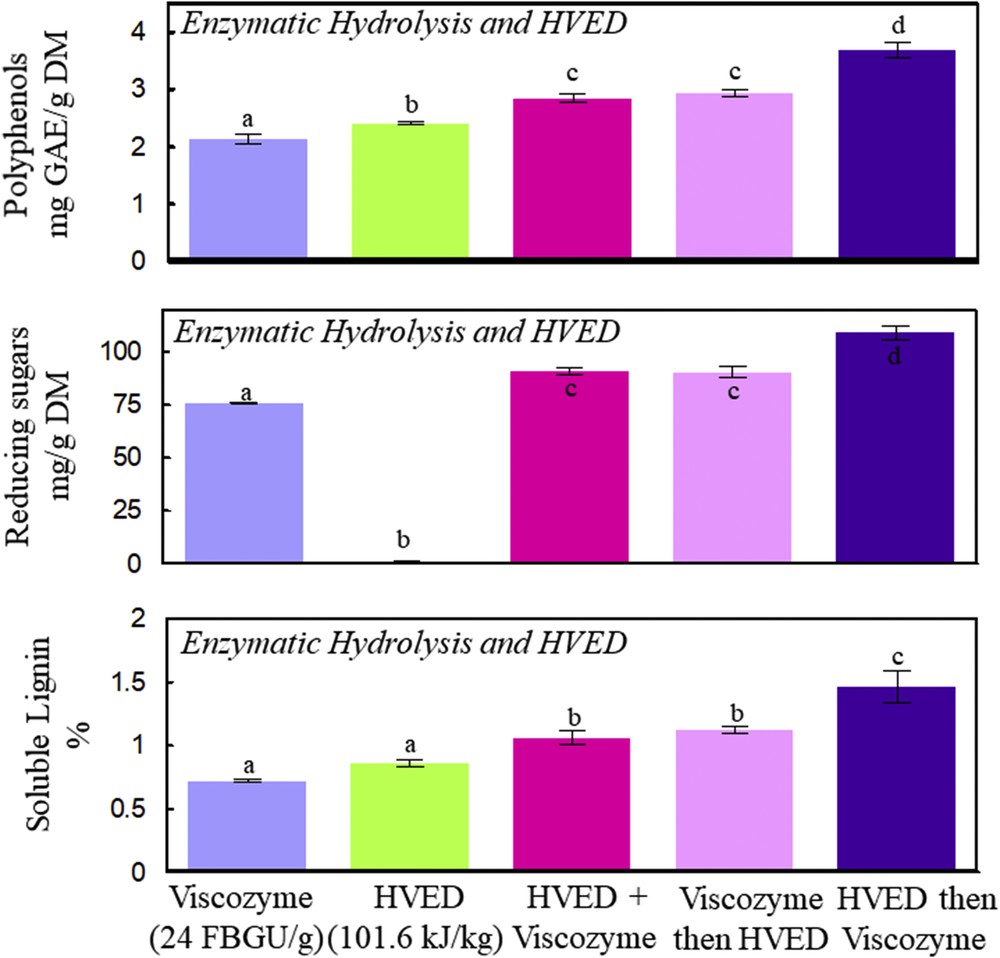
Polyphenols, reducing sugars, and soluble lignin contents as a function of enzymatic and/or HVED treatment. Enzymatic hydrolysis simultaneously, before, and after the HVED treatment. Viscozyme L (24 FBGUs/g) and HVED (101.6 kJ/kg) were used in all experiments at 50 °C, 160 rpm, and 4 h. A significant statistical difference between means is indicated by different letters (a, b, c, and d).
3.4 Effect of different pretreatments on the subsequent delignification step
Fig. 5 shows the KL content (%) of untreated vine shoots or vine shoots subjected to the previously mentioned extraction processes. The first step of the extraction process varied between the samples. It was an enzymatic hydrolysis, an alkaline hydrolysis, an HVED treatment (101.6 kJ/kg), or an HVED pretreatment (101.6 kJ/kg) followed by an enzymatic hydrolysis. The final extraction process was an alkaline hydrolysis (1 M NaOH), and it was conducted on the exhausted vine shoots. The objective of the multistep extraction was to selectively extract polyphenols, reducing sugars, and then study the effect of these different pretreatments on the subsequent delignification process. Untreated vine shoots contained 25% of KL. A first step of enzymatic extraction followed by alkaline extraction reduces this KL by 3.6%, whereas alkaline hydrolysis or HVED treatment reduces it by approximately 6.4%. The highest delignification percentage was obtained when combining HVED with enzymatic hydrolysis. A reduction of 10% KL as compared to the untreated vine shoots was reported (Fig. 5). A preliminary study was conducted on Miscanthus x giganteus to show the effect of the enzymatic pretreatment on the subsequent delignification step by Organosolv treatment. Enzymatic pretreatment resulted in a higher subsequent delignification of Miscanthus x giganteus. It was suggested that mild enzymatic hydrolysis weakens the matrix connecting hemicellulose, cellulose, and lignin [6]. In this study, the intensification of the delignification process was shown to be the most efficient by the combination of HVED, a physical pretreatment, with an enzymatic hydrolysis.

KL content of vine shoots subjected to a first step (Viscozyme L (24 FBGUs/g), alkaline hydrolysis (1 M NaOH), HVED (101.6 kJ/kg), or HVED and Viscozyme L) followed by a second step of alkaline hydrolysis (1 M NaOH) at 50 °C, 160 rpm, and 4 h. A significant statistical difference between means is indicated by different letters (a, b, c, and d).
Fig. 6 shows the polyphenol content (mg GAE/g DM) of the extracts after extraction (with water, Viscozyme L, NaOH 1 M, HVED, or HVED then Viscozyme L) followed by an extraction step with 1 M NaOH. The sum of the values presents the polyphenol mass. As previously shown with KL, a combination of HVED and enzymatic treatments before alkaline hydrolysis significantly enhances polyphenol extraction 1.7 times as compared to water followed by alkaline extraction (P < 0.05). This might be because of the reduced particle size fragmented by HVED and the fragility of the matrix caused by enzymatic treatment.
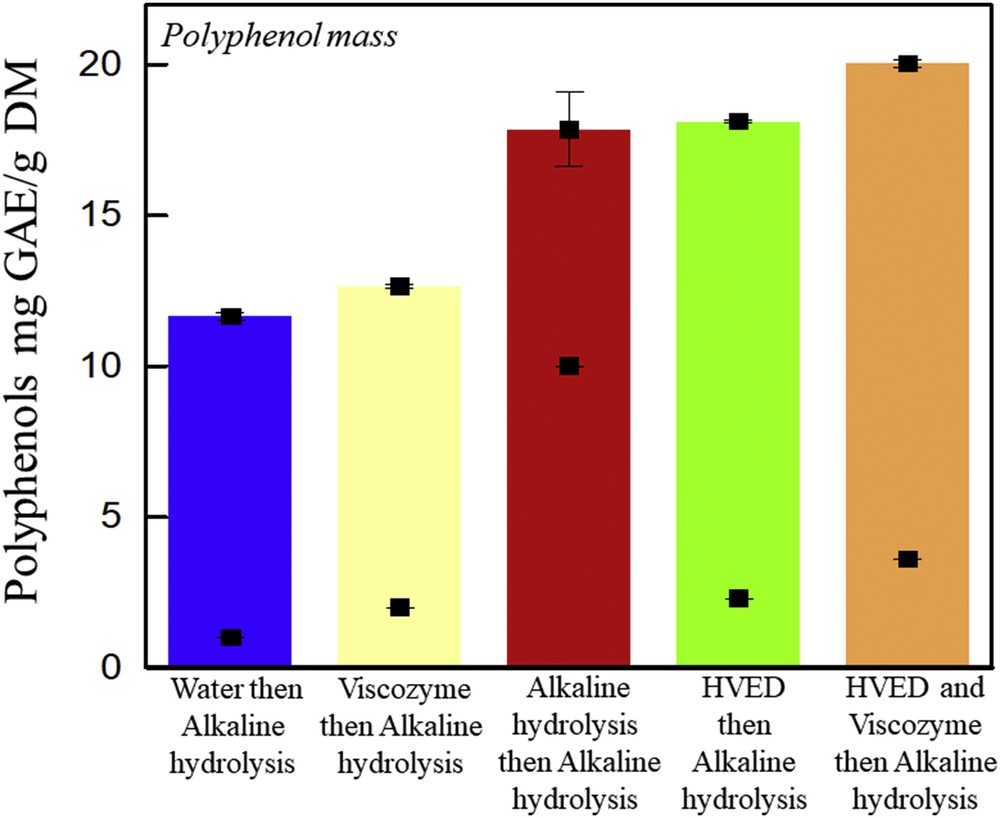
Polyphenol mass. Polyphenol content of vine shoots subjected to a first step (water, Viscozyme L (24 FBGUs/g), alkaline hydrolysis (1 M NaOH), HVED (101.6 kJ/kg), or HVED (101.6 kJ/kg) and Viscozyme L (24 FBGUs/g)) (squares below) followed by a second step of alkaline hydrolysis (1 M NaOH) (squares above) at 50 °C, 160 rpm, and 4 h. The first squares in the bar correspond to the polyphenol content obtained after the first step, and the second squares to the ones obtained after alkaline hydrolysis.
The combination of the physical pretreatment HVED with the biochemical enzymatic treatment before alkaline hydrolysis enhances the delignification process and the polyphenol extraction from vine shoots. The use of enzymes is a green process that reduces solvent use and extraction time enhancing the extraction yield of specific biomolecules [10]. Therefore, the mechanical role of HVED enhances the extraction process of biomolecules by increasing the exchange surface area with the solvent containing the enzymes. The combination of HVED with enzymatic hydrolysis provokes the fragilization of the structure of vine shoots ameliorating thus the release of biomolecules. Soda, on the other hand, generates a more intense disintegration of the structure of the shoots for the release of many biopolymers and many other compounds' bond to the cell wall.
Fig. 7 shows the mass of individual polyphenols identified by HPLC and extracted by water, Viscozyme L, NaOH 1 M, HVED, or HVED then Viscozyme L, followed by an extraction step with 1 M NaOH. FA, R, CA, and hydroxybenzoic acid were detected. The highest concentration of R (26 μg/g DM), CA (140 μg/g DM), and FA (186 μg/g DM) were obtained by combining HVED with enzymatic treatment before alkaline hydrolysis (Fig. 7 (5)). This result is in concordance with that obtained for total phenolic content. Despite recent developments in HVED applications and particularly their positive effect for enhancing the extraction of biocompounds, several areas need further research to make this technology feasible at the commercial level: understanding of the mechanisms of discharge establishment in water; development of extraction kinetics models; identification and application of electrode materials that can provide longer time of operation and lower metal migration; and process system design (including electric generators), evaluation, and cost reduction.
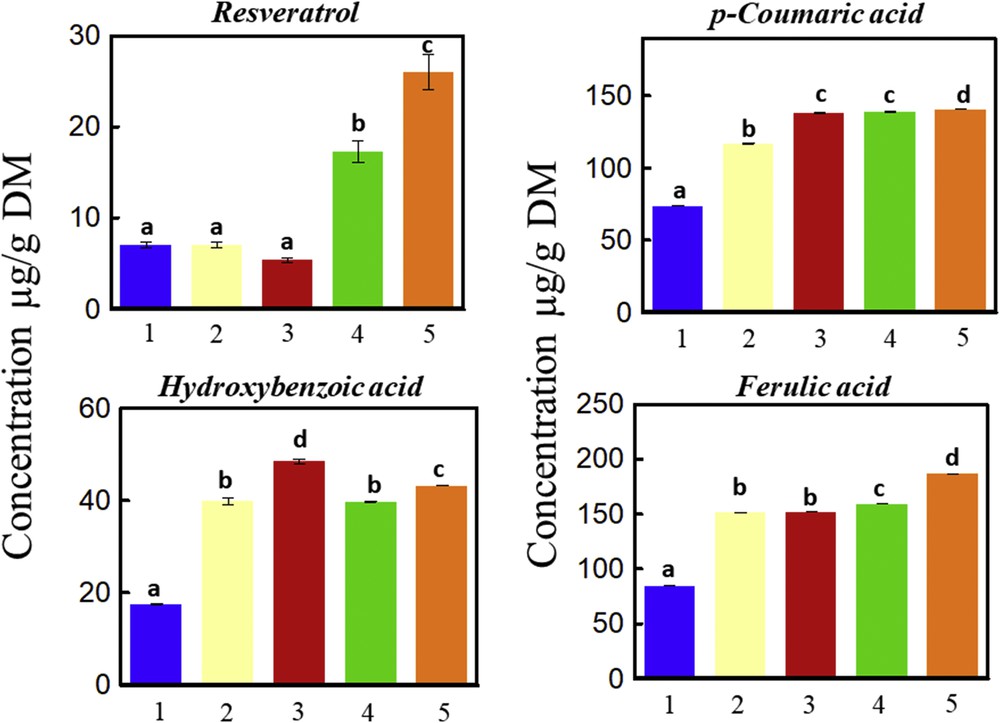
Individual polyphenol mass determined by HPLC. The sum of each polyphenol content of the vine shoots subjected to a first step of (1) water, (2) Viscozyme L (24 FBGUs/g), (3) alkaline hydrolysis (1 M NaOH), (4) HVED (101.6 kJ/kg), or (5) HVED (101.6 kJ/kg) and Viscozyme L (24 FBGUs/g) and the second step of alkaline hydrolysis (1 M NaOH) (squares above) at 50 °C, 160 rpm, and 4 h are shown. A significant statistical difference between means is indicated by different letters (a, b, c, and d).
4 Conclusions
The combination of a physical treatment (HVED) with an enzymatic hydrolysis in the first step extraction process significantly favors the subsequent liberation of polyphenols by alkaline hydrolysis. Enzymatic hydrolysis enhanced the extraction of polyphenols and reducing sugars from vine shoots. However, alkaline hydrolysis was more efficient than enzymatic extraction in terms of polyphenol extraction. The combination of HVED and enzymatic hydrolysis enhanced the extraction of polyphenols, soluble lignin, and reducing sugars. A second step delignification process by alkaline hydrolysis was ameliorated for vine shoots previously treated with HVED combined with enzymatic hydrolysis. Moreover, it significantly increased the release of R, CA, and FA in vine shoot extracts.
Acknowledgments
The authors would like to acknowledge the Research Council of Saint-Joseph University of Beirut (Project FS54) for financial support and Mr. J.-P. Crebassol for providing vine shoots.