1 Introduction
The sulfur-based bis(ethylenedithio)tetrathiafulvalene (BEDT-TTF, Fig. 1) molecule has been used as a building block for a large number of organic conductors, some of which are superconductors at ambient pressure [1]. Salts with coordination complex anions such as κ-(BEDT-TTF)2X, where X = Cu(NCS)2, Cu[N(CN)2]Br, Cu[N(CN)2]Cl or Cu(CN)[N(CN)2], exhibit superconductivity with TC exceeding 10 K [1]. Among them, single crystals of κ-(BEDT-TTF)2Cu(NCS)2 show a TC of 10.4 K. Recently, thin single crystals of κ-(BEDT-TTF)2Cu(NCS)2 have been grown on Au patterned Si/SiO2 wafers [2]. Surface-conductive polymer composites based on (BEDT-TTF)2Cu(NCS)2 have also been reported [3]. To our knowledge, the first nanostructuration and organization of this conductor has been described by Y. Li et al. [4]. They have fabricated nanorod arrays of κ-(BEDT-TTF)2Cu(NCS)2 supported on platinum foil or indium tin oxide glass. The morphologies of the nanorods have been controlled by adjusting the growth current density. We have recently demonstrated simple chemical or electrochemical ways for preparing nanoparticles of (BEDT-TTF)3Cl2, (BEDT-TTF)2Br and (BEDT-TTF)2I3 using ionic liquids, long-chain ammonium salts or neutral amphiphilic molecules as growth controlling agents [5–7]. Among amphiphilic molecules, polycarboxylates have never been studied as growth controlling agents for the preparation of organic-based nanostructured materials. However, trimethyl aconitate derivatives have already been cited as green surfactants [8]. In the present communication, we report on the preparation of (BEDT-TTF)2Cu(NCS)2 nanoparticles using biobased amphiphilic molecules, namely n-dodecanoic acid (n-C11H23COOH) and aconitate esters bearing one or several C12H25 group(s).

Molecular structure of BEDT-TTF.
2 Results and discussion
A previous study has shown the efficiency of long hydrocarbon chain imines as growth controlling agents for the selective synthesis of (BEDT-TTF)2I3 nanoparticles [7]. In the present work, we have studied the growth of (BEDT-TTF)2Cu(NCS)2 as nano-objects by using another class of neutral amphiphilic molecules with an ethylenic group, aconitate esters bearing one or several C12 hydrocarbon chains (AE, Fig. 2). n-dodecanoic acid (n-DA) has also been chosen as a conventional amphiphilic molecule with the same C12 hydrocarbon chain.

Molecular structures of aconitic acid and mono-, di- and tridodecyl aconitates. For mono- and didodecyl aconitates, only one in three molecules has been drawn, the other molecules are obtained by permutation of the C12H25 substituent(s).
Hydrophilic-lipophilic balances (HLB) of n-DA or AE are calculated according to Griffin's empirical method [9]: 4.2 for n-DA, 10.0 for the monoaconitate, 6.4 for the diaconitate, and 5.0 for the triaconitate. Taking into account the composition of esters (see the Experimental section below), a value of 6.0 is found. n-DA and AE belong to the category of emulsifiers dedicated to the dispersion of water in oil.
The chemical oxidation of BEDT-TTF using the copper(II) complex Cu(NCS)2 in a tetrahydrofuran solution containing n-DA or AE leads to an air stable dark brown powder. Whatever be the amphiphilic moiety used, X-ray diffraction patterns are very similar and confirm unambiguously that the κ-(BEDT-TTF)2Cu(NCS)2 phase is obtained (Fig. 3) [10].
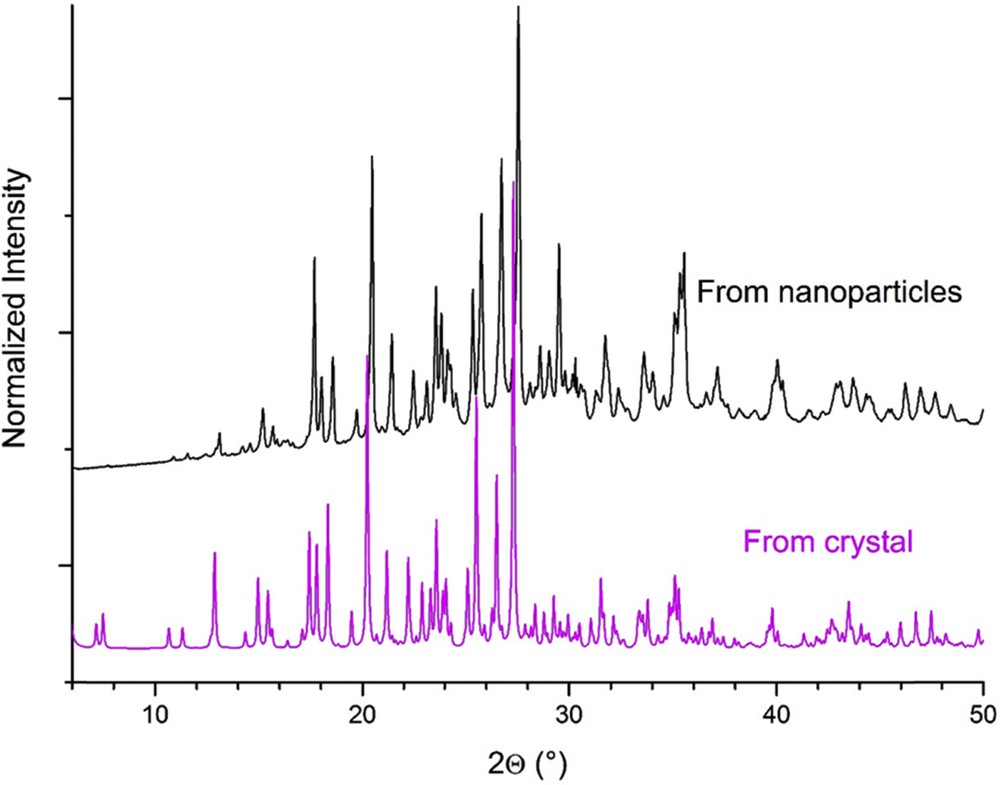
XRD pattern of (BEDT-TTF)2Cu(NCS)2 grown in the presence of n-DA (top) and simulated from (BEDT-TTF)2Cu(NCS)2 single crystals (bottom).
Infrared spectra are also in agreement with those for polycrystalline pressed samples of (BEDT-TTF)2Cu(NCS)2 (see the Experimental section below) [11]. It should be noted that the absence of CO vibrational modes implies the absence of n-DA or AE within the powders. When the synthesis is conducted in the presence of n-DA (3 molar equivalents vs. BEDT-TTF), low-magnification electron micrographs evidence strings of particles as well as few dispersed particles (Fig. 4). When AE is used (3 molar equivalents vs. BEDT-TTF), exclusively well-dispersed roughly spherical nano-objects are observed (sizes in the 35–120 nm range, Fig. 5). Similar electron micrographs are obtained for particles prepared in the presence of 5 and 10 molar equivalents versus BEDT-TTF. As mentioned above (IR spectroscopy), the amphiphilic molecules (n-DA or AE) are not present in the nanopowders. However, their HLB values confer them an appropriate behavior to control the growth of (BEDT-TTF)2Cu(NCS)2 as nano-objects in the solution. It is noticeable that aconitate esters lead to a better dispersion of the nano-objects, showing that the chemical structure of the emulsifier has also a significant role. Indeed, the presence of an ethylenic group in aconitate esters would favor a better controlled growth via π–π interactions with the multiple bonds of the BEDT-TTF molecule, as previously observed [7]. Moreover, the use of more sterically hindered molecules such as AE (in comparison to n-DA) would explain the better state of dispersion observed in the TEM micrographs. The HRTEM images of (BEDT-TTF)2Cu(NCS)2 prepared in the presence of AE are shown in Fig. 6. Nano-objects are actually made of aggregated individual smaller nanoparticles ranging from three to about 10 nm, which are also found isolated on the TEM grid. The nanoparticles exhibit a high degree of crystallinity, and spacings of 2.1 Å are measured. As previously observed for (BEDT-TTF)2I3 nanoparticles [7], the large interplanar spacings corresponding to the main crystallographic planes in the structure (e.g., 15.2 Å for (100), 12.3 Å for (001) or 8.3 Å for (101)) are not visible, most probably due to radiation damage under the 200 kV electron beam. Indeed, organic specimens are particularly sensitive to radiolysis damage, where the electron-electron interactions break chemical bonds and can contribute to creating new structures [12].
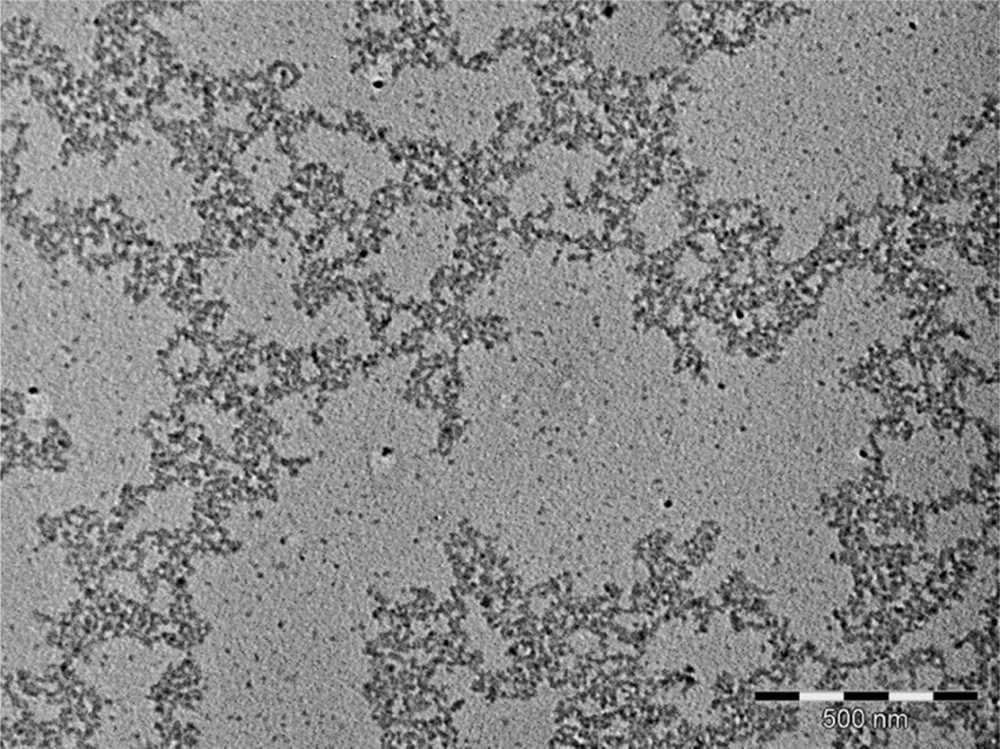
Low-magnification electron micrograph of (BEDT-TTF)2Cu(NCS)2 prepared in the presence of n-DA (bar = 500 nm).
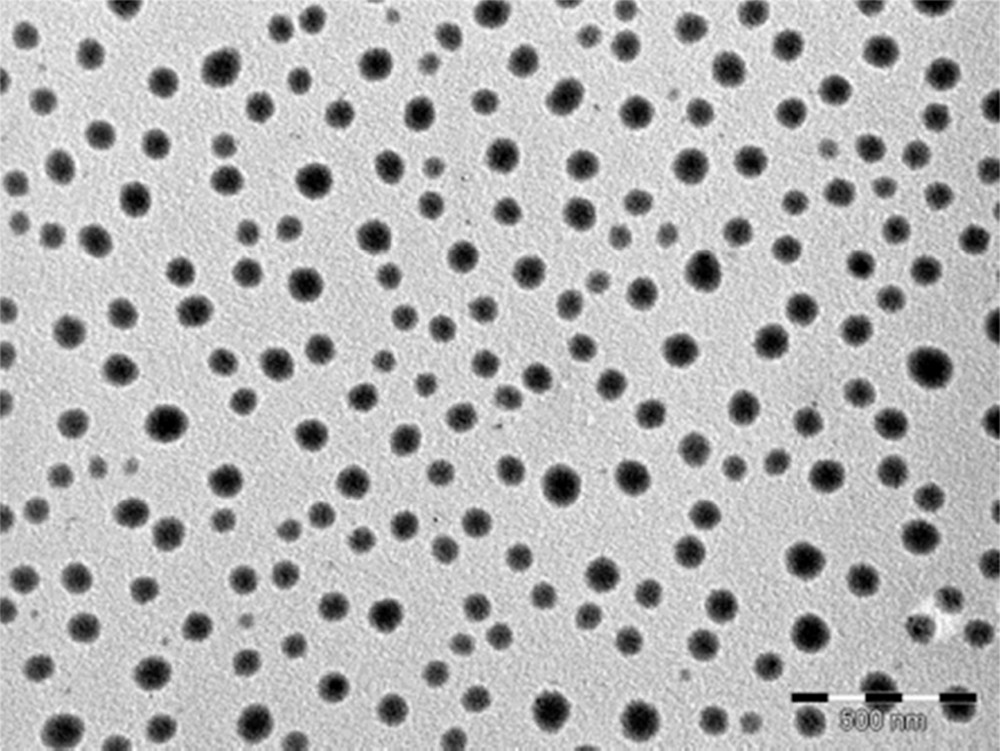
Low-magnification electron micrograph of (BEDT-TTF)2Cu(NCS)2 prepared in the presence of AE (bar = 500 nm).
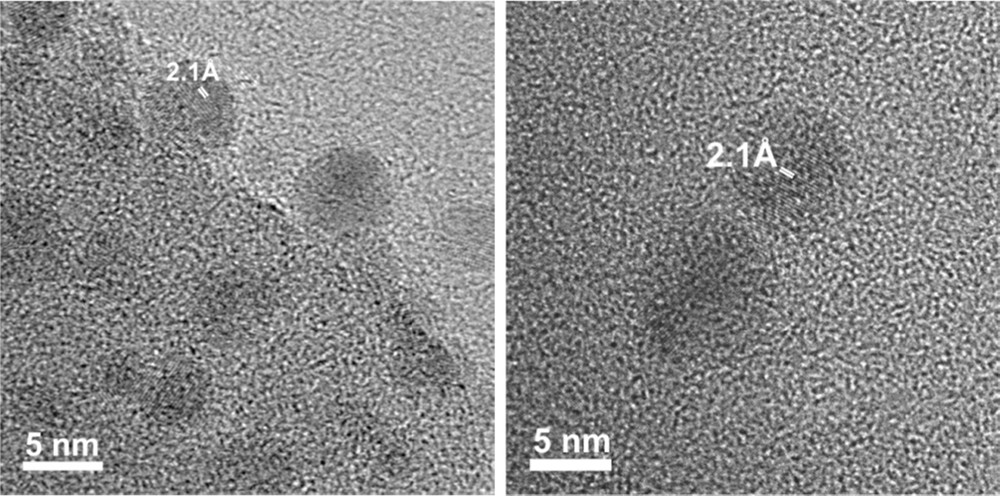
High-magnification electron micrographs of the (BEDT-TTF)2Cu(NCS)2 aggregate (left) and isolated nanoparticles (right) prepared in the presence of AE.
Fig. 7 shows an IV curve of an individual 40 nm high nanoparticle (NP) aggregate measured with an AFM. The deviation from the linear ohmic behaviour that would be expected from a metallic NP arises from the involved boundaries, i.e., NP-NP, tip-NP and substrate-NP. From the curve, an effective resistance may be obtained from the slope at V = 0, but such magnitude is very much dependent on the applied pressure on the NP aggregate, a magnitude that can only be estimated with a large source of error due to the unknown contact area between the tip and the aggregate and that can easily reach the GPa range. Note the asymmetric shape of the curve which suggests a rectifying character of the NP aggregate. Similar curves were obtained for (BEDT-TTF)2I3 nanoparticle aggregates [7].
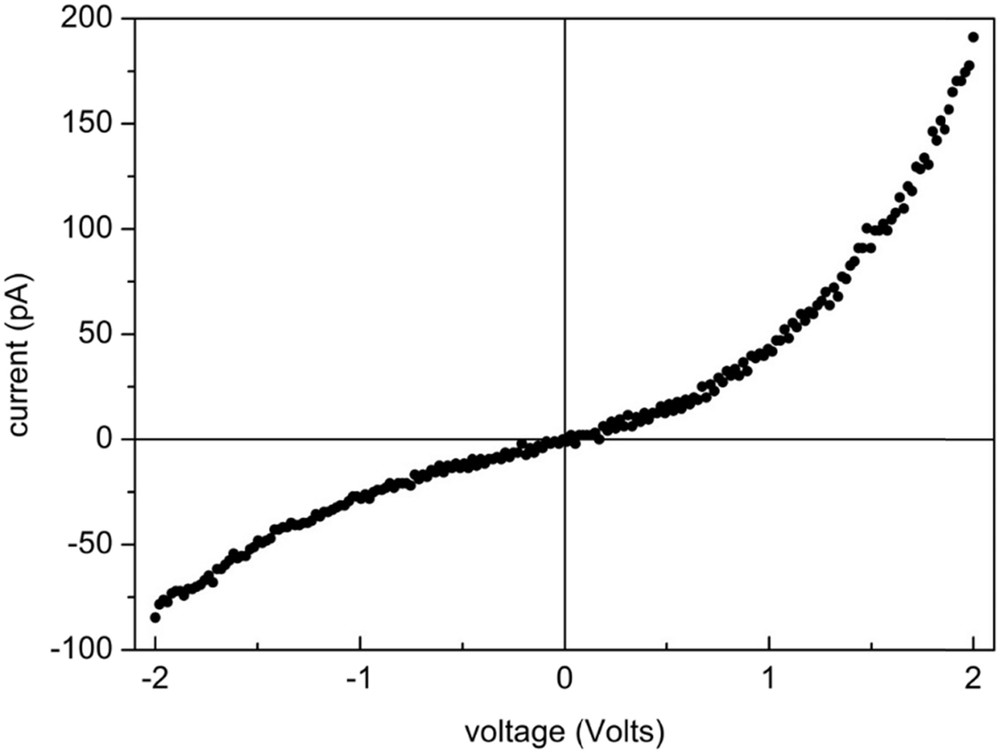
Current-voltage (I–V) curve of an individual NP aggregate with a height of 40 nm measured with an AFM in the conductivity mode.
3 Conclusion
We have isolated the first nanoparticles of the molecule-based superconductor κ-(BEDT-TTF)2Cu(NCS)2, using aconitate esters bearing C12H25 alkyl groups as growth controlling agents. Under the conditions explored, roughly spherical aggregates of very small individual nanoparticles have been obtained. We are currently investigating the use of other aconitate esters bearing C18 oleyl groups with the aim of preparing well dispersed 2–10 nm particles. We have also planned to investigate the superconducting transition of the nanoparticle powders.
4 Experimental section
4.1 General information
All syntheses are performed under an argon atmosphere. Tetrahydrofuran (THF) is distilled and stored under argon prior to use. BEDT-TTF and n-dodecanoic acid are commercially available and used as received. The Cu(NCS)2 complex is prepared following a procedure described in the literature [13].
4.2 Synthesis of aconitate esters
Aconitate esters are prepared as follows: dodecan-1-ol (12.1 g, 65 mmol) is put in a flask while heating at 40 °C, before adding trans-aconitic acid (7.5 g, 43 mmol) under stirring. After complete solubilisation of trans-aconitic acid in dodecan-1-ol, 0.24 g of concentrated sulfuric acid is added. The resulting mixture is then stirred at 100 °C for 5 h, the water being trapped by the molecular sieve placed above the flask. After cooling down to room temperature, 20 mL of ethyl acetate are added to the medium and the organic phase is washed three times with 10 mL of water. Ethyl acetate is then evaporated under vacuum to obtain about 10 g of aconitate esters. The ester composition is calculated from 1H NMR data, using the integrations of ethylenic protons whose chemical shifts are 7.00, 7.03, and 7.06 ppm (for monododecyl esters), 6.95, 6.97, and 7.01 ppm (for didodecyl esters), and 6.93 ppm (for the tridodecyl ester). The composition is as follows: monododecyl, 17%; didodecyl, 16%; tridodecyl, 67%.
4.3 Synthesis of (BEDT-TTF)2Cu(NCS)2 nanoparticles
A THF solution (30 mL) of BEDT-TTF (85 mg, 0.22 mmol), Cu(NCS)2 (20 mg, 0.11 mmol) and n-dodecanoic acid (132 mg, 0.66 mmol) [or 400 mg of the mixture of aconitate esters, i.e., 0.66 mmol, based on the average molar mass] is heated under reflux for 1h15. The resulting suspension is then filtered hot. The precipitate is washed with THF (2 × 20 mL), diethylether (2 × 20 mL), and finally dried under vacuum for 10 h to obtain a dark brown powder (yield: 70%). IR: 875 (νC−S), 1162 and 1249 (δC−CH), 1313 (νCC), 1408 (methylene deformation), 1447 (νCC), 2067 and 2110 (νNC), 2919 and 2963 (νC−H) cm−1.
4.4 Equipment and characterization details
Infrared spectra are obtained at room temperature (in KBr matrix) on a Perkin Elmer Spectrum GX spectrophotometer. X-ray diffraction data are collected at room temperature with a Bruker D8 Advance diffractometer working in the Bragg-Brentano configuration (θ-θ) using Ni filtered CuKα radiation (0.15418 nm) and fitted with a SuperSpeed Vantec Detector. For electron microscopy, the samples are sonicated, dispersed in acetonitrile, and placed onto a holey carbon-copper support grid. Low-magnification TEM experiments are performed on a JEOL Model JEM 1011 operating at 100 kV. HRTEM images are recorded with an FEI Tecnai F20 HRTEM operating at 200 kV. The IV curves of an individual NP aggregate are acquired on an AFM Smarts SPM 1000 (AIST-NT) in conductivity mode using Pt-coated cantilever tips (HQ:NSC15/Pt fromMicroMash). The NPs are dispersed on a gold substrate.
Acknowledgements
B. Cormary thanks the Centre National de la Recherche Scientifique (CNRS) for a post-doctoral fellowship. The authors thank S. Ladeira for XRD characterization and M. Tassé for AFM and IV curves. The authors also thank the GDRI HC3A (Heteroelements and Coordination Chemistry: from Concepts to Applications). The ICN2 is funded by the CERCA programme/Generalitat de Catalunya. The ICN2 is supported by the Severo Ochoa programme of the Spanish Ministry of Economy, Industry and Competitiveness (MINECO, grant no. SEV-2013-0295).