1 Introduction
It is well known that multicomponent reactions constitute a precise synthetic tool for an easy access to multifunctionalized and structurally related drug-like molecules [1,2]. On the other hand, green chemistry principles are playing very dominating role in today's organic synthesis [3]. Consequently, the use of multicomponent reactions in library synthesis of biologically important molecules obeying the demands of green chemistry has become the focal point of current day research [4]. Three main variations involved in green multicomponent synthesis of target molecules are the reaction medium, the energy source, and the catalyst. Compared to reaction medium and energy sources, catalyst can play a very important role in green organic synthesis. Most of the early developments in the field of catalysis were focused on the choice of heterogeneous catalysts; however, in the past few years use of organocatalysts with their well-documented advantages such as ready availability, low cost, low toxicity, and lack of sensitivity toward moisture as well as oxygen is considered as one of the possible solutions to green organic synthesis [5]. A few of the nontoxic organocatalysts practiced in today's organic synthesis are Baker's yeast [6], β-cyclodextrin [7], proline [8], chitosan [9], meglumine [10], thiourea dioxide [11], and so forth. At the same time, search for a new organocatalyst is a constant endeavor.
Indole is the most ubiquitous heterocyclic moiety present in a large number of bioactive natural products [12]. It is known that sharing of indole-3-carbon atom in the formation of spiroindolines and the presence of carbonyl group at C-2 in spiroindolines generate spiro-2-oxindoles (spirooxindoles), which occupy a special place in organic and medicinal chemistry. Several compounds containing spirooxindole as the structural unit of natural and synthetic origin are known to exhibit antimicrobial, antioxidant, antitubercular, anticancer, anti-HIV and anti-inflammatory activities (Fig. 1) [13]. Sharing of C-3 in spirooxindoles with pyran ring generates pyran annulated spirooxindoles (spiropyrans or spirochromenes), which are also known to exhibit useful properties like anticoagulant, spasmolytic, diuretic, anticancer, and antianaphylactic activities [14]. The biological potential of spirochromenes has always been the driving force for the chemists to develop efficient protocols for their synthesis.
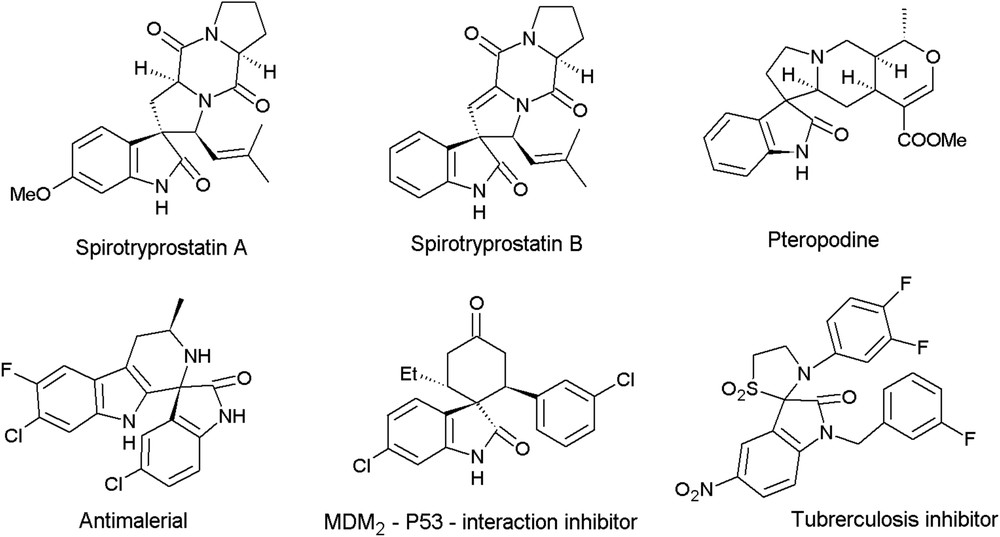
Representative structures of biologically active spirooxindoles.
Classical synthesis of spirochromenes involve one-pot, three-component condensation between isatin, malononitrile, and enolizable CH acids like dimedone, barbituric acid, naphthols, 4-hydroxycoumarin, and so forth, and the literature is enumerated with several protocols for their synthesis. A focused literature survey toward enviroeconomic protocols developed for the synthesis of spirochromenes revealed that a few catalyst-free protocols have been reported earlier [15]. Notably all the catalyst-free protocols developed for the synthesis of spirochromenes require thermal or electrochemical activation. These reports indirectly project the necessity of a catalyst, an alternate energy source, or the reaction medium for the synthesis of spirochromenes at ambient temperature. During our search on protocols operable at ambient temperature it was noticed that a range of catalysts and reaction media have been reported for their synthesis [16–18]. Each of the reported method has its own merits, whereas a few of the reported protocols suffer from the drawbacks as regards the use of expensive or difficult to prepare catalyst or the reaction medium, and to the best of our knowledge there are only a few protocols wherein the problems of economics and environmental protection have been addressed successfully [18a–c]. With our continued interest in the development of organocatalyzed protocols for the synthesis of chromene-based heterocycles [19], we set out to develop an enviroeconomic and diversity-oriented protocol for the synthesis of spirochromenes.
2 Results and discussion
Tris-hydroxymethylaminomethane (THAM) (Scheme 1) is a biodegradable, noncorrosive, physiologically inert, and thermally stable compound available commercially at extremely low cost [20a]. It contains an amino and three primary alcoholic groups and when dissolved in water or water–ethanol medium, it generates basic reaction medium [20b]. In the recent past, we have reported its use as an efficient organocatalyst in the diversity-oriented synthesis of 2-amino-4H-chromenes by multicomponent condensation between aldehydes and two different CH acids [19b]. In continuation of the same, we report herein the use of THAM in the diversity-oriented synthesis of spirochromenes by three-component reaction between isatins, malononitrile, and dimedone, 4-hydroxycoumarin, 4-hydroxy-N-methylquinolone, and in situ generated 2-methylpyrazolon-2-one (Scheme 1).
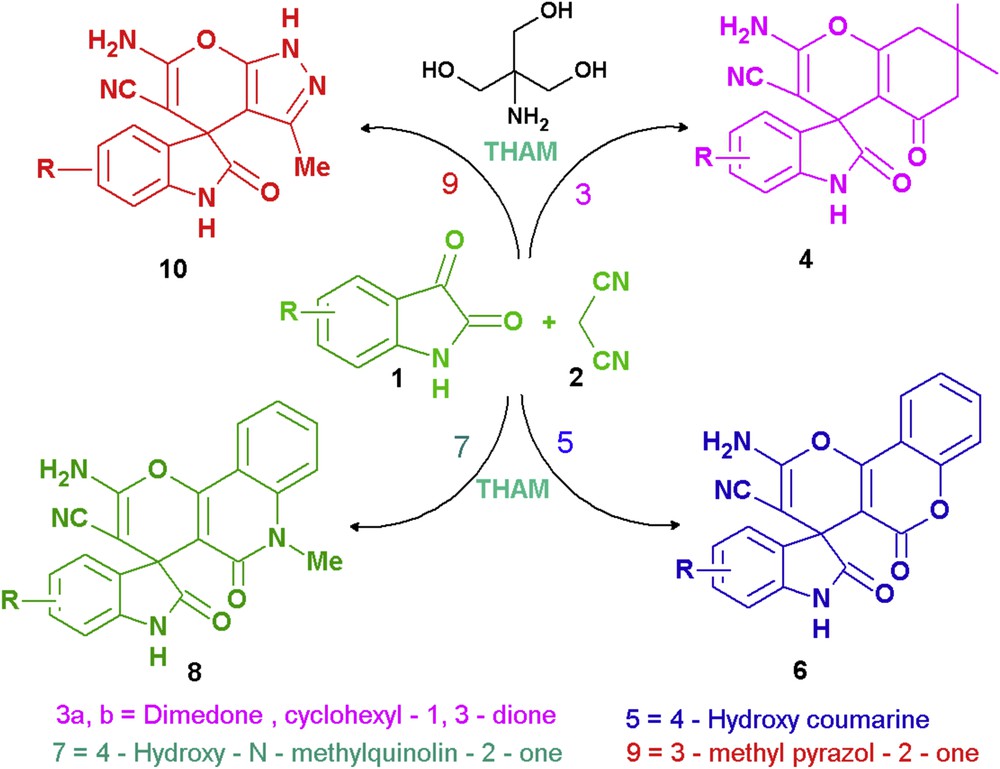
Diversity-oriented synthesis of spirochromenes using THAM as the catalyst.
We began our studies with optimization of the reaction conditions for the synthesis of spiro[indoletetrahydrochromene], 4a, as a model spirochromene (Scheme 1). Accordingly, to an equimolar and well-stirred solution of isatin, 1a, malononitrile, 2, and dimedone, 3a, (1 mmol each) in ethanol (95%, 4 mL) was added THAM (30 mol %) as the catalyst. Stirring was continued at room temperature and progress of the reaction was monitored by thin layer chromatography (TLC). Upon completion of the reaction (4 h), water (5 mL) was added and the resultant solid product was isolated by simple filtration. On the basis of physical and spectral studies, resultant product was identified to be the desired spirochromene, 4a. In pursuance to make this protocol greener, the model reaction was repeated using water and water–ethanol (1:1, v/v) as the reaction media. However, the desired product, 4a, was obtained in slightly lower yields (76% and 82%, respectively). Further optimization of the reaction conditions with respect to catalyst loading revealed that with the use of less than 30 mol % of the catalyst the reaction does not go to completion. In short, use of THAM (30 mol %) as the catalyst and ethanol (95%) as the reaction medium was observed to be the best suited reaction conditions for the optimum yield of the desired product, 4a. To check the scope of the reaction conditions, another reaction was performed by replacing the dimedone component from the model reaction with 5-methylcyclohexane-1,3-dione, 3b. In this case too the expected spirochromene, 4g, resulted in excellent yield. To examine the generality of the reaction conditions, various substituted isatins were then allowed to undergo reaction with malononitrile and dimedone 3a as well as 3b. In all the cases, corresponding spirochromenes, 4b–i, were obtained in excellent yield following simple workup procedure. Most gratifyingly, they were noticed to be pure for all analytical purposes.
From the plausible mechanism (Scheme 2), it is apparent that the enolic form of cyclic-1,3-dione is actually involved in the generation of a pyran ring in spirochromenes. On the basis of this hypothesis we surmised that 4-hydroxycoumarin, 5, and 4-hydroxy-N-methylquinolin-2-one, 7 (Scheme 1), being enolic forms of coumarin-2,4-dione and N-methylquinolin-2,4-dione, respectively, the replacement of dimedone component used in the synthesis of 4a by 5 and 7 would furnish spiro[indole-pyrano[3,2-c]chromene], 6a, and spiro[indole-pyrano[3,2-c]quinolone], 8a, respectively. The salient objective to undertake this extension was associated with the antirheumatic and anticancer activity associated with the compounds containing pyrano[3,2-c]chromene and pyrano[3,2-c]quinolone as the structural units [21].
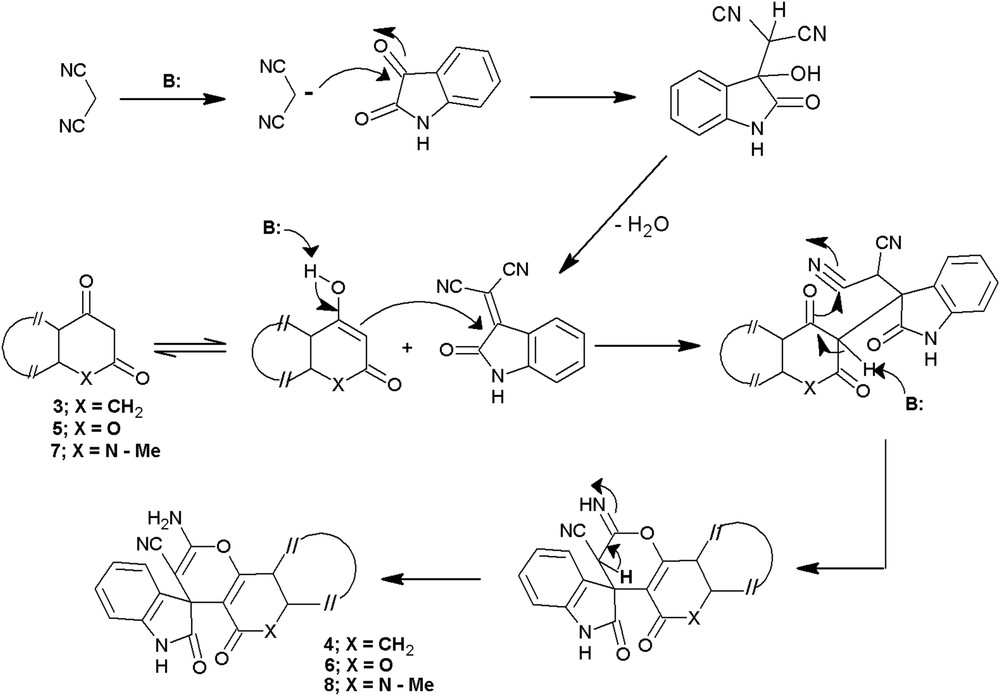
Plausible mechanism for the formation of spirochromenes.
Using reaction conditions established for the synthesis of 4a, two model reactions were performed using isatin, malononitrile, and 5, or 7, as the substrates (Scheme 1). Most gratifyingly, both the reactions proceeded smoothly (TLC) and the desired spirochromene, 6a, and spiroquinoline, 8a, were obtained in decent yields. Under the same reaction conditions, various substituted isatins were also reacted with malononitrile and 4-hydroxycoumarin as well as 4-hydroxy-N-methylquinolin-2-one when corresponding spirooxindole derivatives, 6b–f and 8b–g, were obtained in good to excellent yields (Table 1).
THAM catalyzed diversity-oriented synthesis of spirochromenes.a
Image 2 | ||||||||
Entry | Isatin (1) | CeH acid (3) | Product | Time (h) | Yield (%) | Reference | ||
R | R1 | R2 | ||||||
1 | H | H | H | 3a | 4a | 4 | 94 | [18c] |
2 | H | Me | H | 3a | 4b | 4 | 84 | [17b] |
3 | Me | H | H | 3a | 4c | 3.5 | 86 | [18c] |
4 | H | F | H | 3a | 4d | 4 | 82 | [18c] |
5 | H | NO2 | H | 3a | 4e | 3 | 92 | [17g] |
6 | H | Br | Br | 3a | 4f | 3.5 | 89 | – |
7 | H | H | H | 3b | 4g | 3 | 92 | – |
8 | H | Cl | H | 3b | 4h | 3.5 | 83 | – |
9 | H | NO2 | H | 3b | 4i | 3 | 95 | – |
10 | H | H | H | 3c | 6a | 4 | 87 | [18c] |
11 | H | Cl | H | 3c | 6b | 4 | 90 | [18c] |
12 | H | Me | H | 3c | 6c | 4 | 85 | [16f] |
13 | H | F | H | 3c | 6d | 5 | 80 | [17a] |
14 | H | NO2 | H | 3c | 6e | 3 | 94 | [18c] |
15 | H | H | Cl | 3c | 6f | 4 | 91 | – |
16 | H | Br | Br | 3c | 6g | 3 | 93 | – |
17 | H | Br | H | 3c | 6h | 4 | 89 | [18c] |
18 | H | H | H | 3d | 8a | 4 | 86 | [16d] |
19 | H | Cl | H | 3d | 8b | 4 | 90 | – |
20 | H | Me | H | 3d | 8c | 4.5 | 87 | – |
21 | H | F | H | 3d | 8d | 4 | 84 | – |
22 | H | NO2 | H | 3d | 8e | 3 | 92 | – |
23 | H | H | Cl | 3d | 8f | 4 | 90 | – |
24 | H | Br | Br | 3d | 8g | 3.5 | 87 | – |
25 | H | H | H | 3e | 10a | 4 | 84 | [18c] |
26 | Me | H | H | 3e | 10b | 4 | 87 | [17g] |
27 | H | Cl | H | 3e | 10c | 5 | 83 | [17g] |
28 | H | Br | H | 3e | 10d | 3 | 92 | [16f] |
29 | H | NO2 | H | 3e | 10e | 4 | 86 | [17g] |
a Reaction conditions: isatin, malononitrile, and enolizable CH acid (1 mmol each), THAM (30 mol %), ethanol (95%, 4 mL), rt.
Similar to pyrano[3,2-c]chromene and pyrano[3,2-c]quinolone, dihydropyrano[2,3-c]pyrazole represents an interesting template in medicinal chemistry and several compounds containing dihydropyrano[2,3-c]pyrazole as the structural motif are known to possess antimicrobial [22a], anti-inflammatory [22b], and molluscicidal activity [22c]. Our earlier success in the synthesis of spiro[indole-pyrano[3,2-c]chromenes], 6, and spiro[indole-pyrano[3,2-c]quinolones], 8, prompted us to extend our studies toward the synthesis of spiro[indoline-3,4′-pyrano[2,3-c]pyrazol]-2-ones, 10. A literature pursuance revealed that there are few early reports on the synthesis of title compounds, 10, involving the reaction between isatin, malononitrile, and 2-methylpyrazolon-2-one [23]. In all these methods, necessary 2-methylpyrazolon-2-one was generated in situ at ambient temperature by the reaction between a β-keto ester and hydrazine hydrate under catalyst-free condition. Taking recourse to these reports, a pseudo four-component reaction was carried out between isatin, malononitrile, ethyl acetoacetate, and hydrazine hydrate (1 mmol, each), using the reaction conditions established for the synthesis of spirochromenes, 4 (Scheme 1). The reaction proceeded smoothly and upon its completion, desired spiro[indoline-3,4′-pyrano-[2,3-c]pyrazol]-2-one, 10a, was obtained in decent yield. Subsequent reactions of substituted isatins with malononitrile and in situ generated 2-methylpyrazolon-2-one, 9, also furnished expected spiro[indoline-3,4′-pyrano[2,3-c]pyrazol]-2-ones, 10b–e, in decent yields (Table 1). Noteworthy feature of the protocol developed for the synthesis of spirochromenes 4, 6, 8, and 10 is that all the products were isolated by a simple procedure and none of them required chromatographic purification.
After establishing a general protocol for the diversity-oriented synthesis of spirochromenes from environmental point of view, it was quite logical to check the possibility of reuse of the catalyst. However, the catalyst, THAM, being soluble in water, it was surmised that making this nontoxic and biodegradable catalyst reusable would certainly be more expensive than the cost of the catalyst itself. Thus, no attempts were made to check reusability of the catalyst. Finally, a comparison was made between the present protocol and many other protocols reported earlier for the synthesis of 4a as a model compound. From the results summarized in Table 2, it is evident that the developed protocol is superior to most of the earlier reported protocols in terms of operational simplicity, yield, cost, easy availability, and environmental compatibility of the catalyst used.
Comparison of THAM catalyzed synthesis of 4a with earlier reported protocols.
Entry | Catalyst | Solvent | Time | Yield (%) | Reference |
1 | Fe3O4@APTPOSS MNPs | – | 45 h | 88 | [17a] |
2 | Sulfated choline-based heteropolyanion | Ethanol | 45 h | 91 | [16a] |
3 | Fe2O3 NPs@SiO2@vitB1-Npa | )))), water | 10 h | 98 | [17b] |
4 | EDDFb in PEG 600 | SF | 12 h | 91 | [16b] |
5 | Cesium fluoride | Ethanol | 5 h | 82 | [17c] |
6 | Nickel oxide nanoparticles | Water | 5 h | 98 | [17e] |
7 | [Bmim]OH | [Bmim]OH | 10 h | 96 | [16f] |
8 | Amino-appended β-cyclodextrin | Water | 7 h | 91 | [17h] |
9 | (DABCO)@mesoporous silica SBA-15 | Water | 10 h | 96 | [17f] |
10 | ZrO2 nanoparticles | SF | 1 min | 84 | [17g] |
11 | Trisodium citrate trihydrate | Water–EtOH | 2 h | 92 | [18c] |
12 | Protic guanidinium ionic liquid | SF | 5 h | 98 | [16c] |
13 | [Bmim]BF4 | SF | 3 h | 94 | [16d] |
14 | (Silica-bonded DBU) Cl | EtOH | 2.5 h | 97 | [17j] |
15 | FeN3@SiO2 nanoparticles | Water | 10 h | 95 | [17d] |
16 | CuO nanoparticles | EtOH | 8 min | 98 | [16e] |
17 | Meglumine | EtOH | 18 min | 96 | [18a] |
18 | Sodium acetatec | EtOH | 15 min | 95 | [18b] |
18 | THAM | EtOH | 4 h | 94 | This work |
a Ultrasound.
b EDDF: ethylenediammonium diformate.
c Grinding.
3 Conclusion
We have demonstrated for the first time the use of a commercially available and biodegradable THAM as an efficient organocatalyst in diversity-oriented synthesis of spirochromenes. The protocol mainly exhibits remarkable versatility on various substrates to afford the desired products in good to excellent yields. Ambient reaction conditions, simple workup procedure, and avoidance of conventional purification methods have improved the practical utility of the protocol manifold.
4 Experimental section
4.1 General procedure for the synthesis of spirooxindoles, 4, 6, 8 and 10
To a well-stirred solution of isatin and malononitrile (1 mmol each) in ethanol (95%, 4 mL) was added dimedone, 4-hydroxycoumarin, 4-hydroxy-N-methylquinolin-2-one, or 2-methyl-pyrazol-2-one [generated in situ from ethyl acetoacetate and hydrazine hydrate, 1 mmol each]. To this solution was added THAM (30 mol %) and stirring was continued at ambient temperature. Upon completion of the reaction (TLC), water (5 mL) was added and stirring was continued for 10 min more. Resultant solid product was filtered, washed repeatedly with water, and dried. The dried solid was washed thrice with hexane–chloroform mixture (1:1, v/v) and dried again. Resultant product did not require any further purification.
4.2 Spectral data of new compounds
2-Amino-5′,7′-dibromo-8,8-dimethyl-2′,5-dioxo-5,6,7,8-tetrahydrospiro[chromene-4,3′-indoline]-3-carbonitrile, 4f: white solid; mp > 280 °C; IR (KBr): 3357, 2197, 1732 cm−1; 1H NMR (DMSO-d6, 300 MHz): δ 1.03 (d, 6H, J = 2.4 Hz), 2.14 (s, 2H), 2.52 (d, 2H, J = 7.2 Hz), 6.78 (d, 1H, J = 8.1 Hz), 6.95 (s, 1H), 7.09 (br s, 2H), 10.47 (s, 1H); 13C NMR (DMSO-d6, 75 MHz): δ 27.89, 28.04, 32.32, 47.57, 50.54, 57.48, 110.86, 110.96, 117.43, 123.49, 126.38, 128.31, 136.57, 141.35, 159.30, 164.68, 178.23, 195.08 ppm.
2-Amino-2′,5-dioxo-7-methyl-5,6,7,8-tetrahydrospiro[chromene-4,3′-indoline]-3-carbonitrile, 4g: white solid; mp > 280 °C; IR (KBr): 3364, 2192, 1721 cm−1; 1H NMR (DMSO-d6, 300 MHz): δ 1.01 (d, 3H, J = 5.1 Hz), 2.05 (m, 1H), 2.24 (t, 2H, J = 15 Hz), 2.64 (t, 2H, J = 18.3 Hz), 6.78 (d, 1H, J = 7.2 Hz), 6.88 (d, 1H, J = 7.3 Hz), 6.94 (m, 1H), 7.12 (t, 1H, J = 7.5 Hz), 7.19 (br s, 2H), 10.40 (s, 1H); 13C NMR (DMSO-d6, 75 MHz):δ 20.50, 27.81, 34.68, 34.92, 44.78, 47.31, 58.92, 109.61, 111.86, 112.07, 117.75, 122.02, 123.45, 123.57, 128.51, 134.93, 142.47, 159.14, 165.24, 165.79, 178.6, 195.3 ppm.
2-Amino-2′,5-dioxo-7′-chloro-7-methyl-5,6,7,8-tetrahydrospiro[chromene-4,3′-indoline]-3-carbonitrile, 4h: white solid; mp > 280 °C; IR (KBr): 3342, 2202, 1736 cm−1; 1H NMR (DMSO-d6, 300 MHz): δ 1.03 (d, 3H, J = 6 Hz), 2.01–2.08 (m, 1H), 2.24–2.36 (m, 2H), 2.26 (d, 2H, J = Hz), 6.76 (d, 1H, J = 8.1 Hz), 6.95 (d, 1H, J = 4.5 Hz), 7.08 (s, 1H), 7.12 (br s, 2H), 10.46 (s, 1H); 13C NMR (DMSO-d6, 75 MHz): δ 20.51, 20.66, 27.71, 27.89, 44.87, 47.60, 58.92, 110.89, 111.48, 123.50, 123.62, 126.46, 128.25, 141.17, 159.21, 165.50, 178.31, 195.08 ppm.
2-Amino-2′,5-dioxo-7-methyl-7′-nitro-5,6,7,8-tetrahydrospiro[chromene-4,3′-indoline]-3-carbonitrile, 4i: white solid; mp > 280 °C; IR (KBr): 3365, 2199, 1726 cm−1; 1H NMR (DMSO-d6, 300 MHz): δ 1.02 (s, 3H), 2.00 (s, 1H), 2.24–2.56 (m, 4H), 6.71–7.18 (m, 5H), 10.44 (s, 1H); 13C NMR (DMSO-d6, 75 MHz):δ 20.55, 27.90, 35.15, 58.92, 111.42, 114, 126.31, 131.10, 159.17, 165.80, 178.24, 195.32 ppm.
2′-Amino-7-chloro-2,5′-dioxo-5′H-spiro[indoline-3,4′-pyrano[3,2-c]chromene]-3′-carbonitrile, 6f: off-white solid; mp > 280 °C; IR (KBr): 3361, 2197,1737 cm−1; 1H NMR (DMSO-d6, 300 MHz): δ 6.97 (t, 1H, J = 7.8 Hz), 7.26 (q, 2H, J = 9.3 Hz), 7.54 (q, 2H, J = 8.4 Hz), 7.77 (s, 3H), 7.95 (d, 1H, J = 6.9 Hz), 11.15 (s, 1H); 13C NMR (DMSO-d6, 75 MHz): δ 19.00, 48.95, 56.50, 56.97, 101.40, 112.89, 114.20, 117.19, 117.28, 123.21, 123.40, 123.85, 125.54, 129.48, 134.28, 135.21, 140.43, 152.56, 155.73, 158.87, 158.99, 177.66 ppm.
2′-Amino-5,7-dibromo-2,5′-dioxo-5′H-spiro[indoline-3,4′-pyrano[3,2-c]chromene]-3′-carbonitrile, 6g: white solid; mp > 280 °C; IR (KBr): 3361, 2205, 1734 cm−1; 1H NMR (DMSO-d6, 300 MHz): δ 7.54 (q, 2H, J = 8.4 Hz), 7.65 (d, 2H, J = 10.8 Hz), 7.78 (d, 1H, J = 7.5 Hz), 7.83 (s, 2H), 7.94 (d, 1H, J = 7.8 Hz), 11.19 (s, 1H); 13C NMR (DMSO-d6, 75 MHz): δ 19.00, 49.40, 56.49, 100.86, 103.07, 113.04, 114.77, 117.19, 117.24, 123.27, 125.52, 127.11, 143.09, 134.29, 136.84, 141.74, 152.60, 156.04, 159.05, 159.09, 177.37 ppm.
2-Amino-6-methyl-2′,5-dioxo-5,6-dihydro-spiro[pyrano[3,2-c]quinoline-4,3′-indoline]-3-carbonitrile, 8a: yellowish solid; mp > 280 °C; IR (KBr): 3420, 2199, 1721, 1675 cm−1; 1H NMR (DMSO-d6, 300 MHz): δ 3.48 (s, 3H), 6.84–7.00 (m, 2H), 7.02 (d, 1H, J = 7.2 Hz), 7.17 (t, 1H, J = 7.4 Hz), 7.46 (s, 3H), 7.59 (d, 1H, J = 8.7 Hz), 7.76 (t, 1H, J = 4.5 Hz), 8.06 (d, 1H, J = 7.8 Hz), 10.52 (S, 1H); 13C NMR (DMSO-d6, 75 MHz): δ 29.69, 48.65, 57.75, 106.97, 109.68, 112.76, 115.50, 117.89, 122.15, 122.87, 123.88, 128.77, 132.72, 134.74, 139.14, 142.97, 151.96, 159.22, 159.36,178.29 ppm.
2-Amino-6-methyl-5′-chloro-2′,5-dioxo-5,6-dihydro-spiro[pyrano[3,2-c]quinoline-4,3′-indoline]-3-carbonitrile, 8b: brown color solid; mp > 280 °C; IR (KBr):3417, 2192, 1717, 1670 cm−1; 1H NMR (DMSO-d6, 300 MHz): δ 3.49 (s, 3H), 6.80–6.84 (m, 1H), 7.00–7.05 (m, 1H), 7.41–7.46 (m, 1H), 7.53–7.60 (m, 3H), 7.37–7.8 (m, 1H), 8.04 (d, 1H, J = 7.8 Hz), 10.56 (s, 1H); 13C NMR (DMSO-d6, 75 MHz): δ 29.72, 49.17, 57.19, 106.41, 110.21, 110.32, 111.71, 112.82, 115.09, 115.51, 117.81, 122.89, 122.94, 132.77, 136.38, 136.48, 139.20, 152.17, 159.28, 159.46, 178.38 ppm.
2-Amino-6-methyl-5′-methyl-2′,5-dioxo-5,6-dihydro-spiro[pyrano[3,2-c]quinoline-4,3′-indoline]-3-carbonitrile, 8c: off-white solid; mp > 280 °C; IR (KBr):3420, 2192, 1721,1660 cm−1; 1H NMR (DMSO-d6, 300 MHz): δ 3.19 (s, 3H), 3.46 (s, 3H), 6.93–7.09 (m, 3H), 7.08 (s, 1H), 7.28 (t, 1H, J = 7.5 Hz), 7.45 (t, 1H, J = 7.5 Hz), 7.52 (s, 2H), 7.58 (d, 1H, J = 8.7 Hz), 7.76 (t, 1H, J = 7.2 Hz), 8.08 (d, 1H, J = 7.5 Hz); 13C NMR (DMSO-d6, 75 MHz): δ 26.94, 29.73, 48.26, 57.33, 106.85, 108.60, 112.74, 115.56, 117.77, 122.90, 123.65, 129.01, 132.08, 133.86, 139.15, 144.45, 151.99, 159.17, 159.47, 176.84 ppm.
2-Amino-6-methyl-5′-fluoro-2′,5-dioxo-5,6-dihydro-spiro[pyrano[3,2-c]quinoline-4,3′-indoline]-3-carbonitrile, 8d: off-white solid; mp > 280 °C; IR (KBr): 3416, 2197, 1721, 1670 cm−1; 1H NMR (DMSO-d6, 300 MHz): δ 3.49 (s, 3H), 6.85 (d, 1H, J = 7.8 Hz), 7.21 (d, 2H, J = 7.8 Hz), 7.44 (t, 1H, J = 7.5 Hz), 7.58 (t, 3H, J = 9.9 Hz), 7.76 (t, 1H, J = 7.5 Hz), 8.06 (d, 1H, J = 7.8 Hz), 10.67 (s, 1H); 13C NMR (DMSO-d6, 75 MHz): δ 29.75, 48.94, 57.02, 106.27, 111.03, 112.86, 115.53, 117.81, 122.91, 122.97, 124.21, 126.16, 128.66, 132.78,136.78, 139.21, 141.93, 152.25, 159.30, 159.49, 178.14 ppm.
2-Amino-6-methyl-5′-nitro-2′,5-dioxo-5,6-dihydro-spiro[pyrano[3,2-c]quinoline-4,3′-indoline]-3-carbonitrile, 8e: off-white solid; mp > 280 °C; IR (KBr): 3430, 2192, 1730, 1665, 1520, 1337 cm−1; 1H NMR (DMSO-d6, 300 MHz): δ 3.48 (s, 3H), 7.07 (d, 1H, J = 8.4 Hz), 7.46 (t, 1H, J = 7.5 Hz), 7.59 (d, 1H, J = 8.4 Hz), 7.67 (s, 2H), 7.77 (t, 1H, J = 7.5 Hz), 8.07 (d, 2H, J = 8.7 Hz), 8.17 (d, 1H, J = 8.7 Hz), 11.29 (s, 1H); 13C NMR (DMSO-d6, 75 MHz): δ 29.79, 48.83, 56.19, 105.71, 109.82, 112.91, 115.59, 117.70, 119.94, 123, 123.06, 126.33, 132.90, 135.78, 139.26, 142.98, 149.51, 152.60, 159.39, 159.74, 179 ppm.
2-Amino-6-methyl-7′-chloro-2′,5-dioxo-5,6-dihydro-spiro[pyrano[3,2-c]quinoline-4,30-indoline]-3-carbonitrile, 8f: brown colored solid; mp > 280 °C; IR (KBr):3370, 2200, 1724, 1674 cm−1; 1H NMR (DMSO-d6, 300 MHz): δ 3.49 (s, 3H), 6.90 (t, 1H, J = 7.8 Hz), 7.03 (d, 1H, J = 7.2 Hz), 7.24 (d, 1H, J = 7.8 Hz), 7.45 (t, 1H, J = 7.2 Hz), 7.58 (s, 3H), 7.76 (t, 1H, J=7.5 Hz), 8.06 (d, 1H, J = 3.2 Hz), 10.99 (s, 1H). 13C NMR (DMSO-d6, 75 MHz): δ 29.75, 49.51, 57.11, 106.47, 112.71, 113.97, 115.59, 117.76, 122.66, 122.93, 123, 123.48, 128.90, 132.88, 136.46, 139.18, 140.72, 152.06, 159.26, 159.44, 178.32 ppm.
2-Amino-6-methyl-5′,7′-dibromo-2′,5-dioxo-5,6-dihydro-spiro[pyrano[3,2-c]quinolone-4,3′-indoline]-3-carbonitrile, 8g: off-white solid; mp > 280 °C; IR (KBr): 3455, 3260, 2200, 1723, 1671 cm−1; 1H NMR (DMSO-d6, 300 MHz): δ 3.50 (s, 3H), 7.42–7.48 (m, 2H), 7.63 (d, 4H, J = 5.7 Hz), 7.77 (t, 1H, J = 7.5 Hz), 8.06 (d, 1H, J = 7.8 Hz), 11.03 (s, 1H); 13C NMR (DMSO-d6, 75 MHz): δ 29.81, 50.02, 56.50, 56.54, 102.85, 105.90, 112.86, 114.38, 115.61, 117.72, 123.03, 126.29, 132.92, 133.50, 138.21, 139.25, 142.05, 152.34, 159.34, 159.57, 178.01 ppm.
Acknowledgments
U.V.D. and S.S.K. thank UGC, New Delhi, for the financial support [F. 43-221/2014 (SR)]. The authors are thankful to M/s B. J. Corporation, Kolhapur, for the generous gift of 4-hydroxy-N-methylquinolin-2-one.