1 Introduction
For centuries, glasses and ceramics have been made via solid-state reactions at temperatures around 1000 °C. Such high temperatures lead to the usual stable and dense phases. Nowadays, nanostructured materials are becoming more and more important for modern technologies. They open new possibilities and extensive researches are devoted to improve the synthesis of such advanced materials. However, solid-state chemistry still involves rather harsh conditions. Looking at nature shows that living organisms are able to make biomaterials under much softer conditions. Biomineralization currently occurs in water at room temperature leading to nanostructured materials that often exhibit much better properties than our best materials. Looking at nature therefore offers a real challenge for solid-state chemists. In their search for new methods to make silica-based materials, chemists have turned their attention toward microorganisms such as radiolarians, diatoms, or sponges that are able to make biogenic silica glasses under ambient conditions [1]. Therefore could we learn from nature how to make new bioinspired nanostructured materials? This article describes the soft solution synthesis of silica-based materials via the so-called “sol–gel process.”
2 From biogenic glasses to sol–gel chemistry
Silica glasses are very common materials, currently used for making windows, bottles, or optical fibers. Many progresses have been made to improve their properties since their discovery about 5000 years ago, but they are still made from molten sand at temperatures much greater than 1000 °C. The example of diatoms shows that nanostructured silica glasses can be made at room temperature in water. Diatoms are a widespread group of planktonic microalgaes that can be found in the sea, fresh water, wet soils, and damp surfaces (Fig. 1). They exhibit photosynthetic properties and are responsible for about 25% of oxygen on earth. These single cell algaes are known to built a silica shell called “frustule” to protect themselves against predators. These shells are made via the condensation of silicic acid molecules Si(OH)4 arising from the dissolution of silicate minerals in water as follows [2]:
SiO2 + 2H2O → Si(OH)4 |

Diatoms are singe-cell planktonic microalgae protected by a silica shell called frustule that exhibit a large variety of shapes.
A silica network is then obtained via the polycondensation of silicic acid molecules:
SiOH + HOSi → SiOSi + H2O |
Silica nanoparticles are formed inside the cell in a silica deposition vesicle and then transported outside the cell to build the silica shell.
Actually precipitated silica powders are already made in industry via the acidification of sodium silicate solutions (water glass). However, such solutions are highly basic and the precipitation of silica is not easy to control. Therefore chemists currently use alkoxide molecular precursors Si(OR)4 (R = CH3, C2H5, etc.). Upon hydrolysis, these silicon alkoxides lead to the formation of silica:
Si(OR)4 + 2H2O ⇒ SiO2 + 4ROH |
Colloidal solutions (sols) and gels are progressively formed and this soft solution synthesis of oxide materials is commonly called the “sol–gel process”[3]. Silica glasses can then be made at temperatures much lower than the usual melting point, and sol–gel materials are now widely used in industry. Besides energy saving, the main advantage of sol–gel chemistry is that shaped materials can be obtained directly from the solution. Following this powderless processing of glasses and ceramics, nanoparticles can be formed by spray, fibers by gel extrusion, and films by dip coating. Moreover as high temperatures are not required, coatings can be deposited onto various substrates such as silicon, metals, electronic components, and even polymers [4].
The mild conditions associated with sol–gel chemistry allow the formation of hybrid nanocomposites in which organic and inorganic phases are mixed at the molecular level. A whole set of new materials, ranging from brittle glasses to plastic polymers can then be made. They are transparent and can be easily shaped by molding and polishing. Organic–inorganic hybrids already found a large number of applications in various domains: optics, microelectronics, transportation, health, energy, and so forth [5].
3 Bioinorganic hybrids
The development of biotechnology requires the immobilization of active biomolecules or cells on solid substrates. Numerous techniques such as physical adsorption, covalent grafting, entrapment, or encapsulation have been explored. But no single method or material has emerged as a generic and universal technology. Nowadays and for obvious reasons, immobilization is mainly restricted to organic or biopolymers. Inorganic silica glasses could offer some advantages such as improved mechanical strength, chemical stability, and thermal stability. They are biologically inert and do not swell in aqueous or organic solvents. Therefore combining fragile biomolecules with tough inorganic materials becomes a highly innovative research field. However, the usual high temperature processing of ceramics and glasses is not compatible with fragile biomolecules. Therefore, new “chimie douce” processes have been developed allowing the formation of a solid network via biocompatible routes [6].
Sol–gel encapsulation is quite easy to perform. Biomolecules such as enzymes are mixed with a solution of molecular precursors. Condensation reactions lead to the growth of a silica network around the biomolecules that remain physically trapped within the porous solid. Wet silica gels provide a nontoxic and biologically inert aqueous environment. Enzymes are trapped into silica cages tailored to their size. They retain their catalytic activity and appear to be protected against denaturation and leaching. Moreover, their catalytic activity can be optimized via a chemical control of the porous sol–gel matrix [7]. Lipases, for instance, are widely used in industry as a biocatalyst for hydrolysis and esterification reactions. However esters are usually not soluble with water giving rise to emulsions rather than clear solutions. The catalytic reaction then occurs at the interface between both oil and water phases. It is therefore very sensitive to the hydrophilic–hydrophobic nature of this interface. The catalytic properties of lipases in hydrophilic sol–gel silica are very poor, but they become much better when hybrid organic–inorganic gels are used. Sol–gel lipases are now commercially available [8].
4 Living materials
Diatoms are made of a single cell trapped within a silica shell. They suggest that living cells could be trapped within an oxide matrix. Actually, the first article was published by Carturan et al. [9] who showed the bioactivity of yeast cells (Saccharomyces cerevisiae) immobilized in porous silica was preserved after sol–gel encapsulation. Since then whole cell encapsulation has been extended to many other prokaryotic and eukaryotic species (bacteria, microalgae, plant cells, and animal cells) [10,11]. Trapped cells remain accessible to external reagents through the pores of the silica matrix and can then be used for the realization of biosensors or bioreactors. Chlorella vulgaris microalgae, for instance, have been trapped within a thin film deposited at the end of an optical fiber to measure water pollution. Their photosynthetic behavior depends on the presence of herbicides in the water leading to a modification of chlorophyll fluorescence that can be easily measured through the optical fiber [12].
One of the main challenges for the sol–gel encapsulation of living cells would be to preserve their viability as long as possible. Trapped cells have to fight against the stress due to the solid matrix. Escherichia coli bacteria, for instance, have been shown to retain their biocatalytic activity after encapsulation but the number of viable bacteria decreases quite fast [13,14]. Their viability can be improved by adding some chemicals such as glycerol to protect the cell (Fig. 2). Then they can be used as a bioreactor to produce some chemicals. Serratia marcescens bacteria, for instance, are known to produce a red pigment called prodigiosin that exhibits some cytotoxic activity against cancer cells. We have shown that these bacteria retain their metabolic activity when trapped in silica gels. They even produce more pigment than when they are just suspended in a solution. This should be because of the diffusion of the hydrophobic prodigiosin pigment from the hydrophilic silica matrix toward the supernatant organic solution that contains all nutrients. This can be easily seen with naked eyes. The silica matrix where bacteria are trapped remains colorless whereas the solution becomes pink.
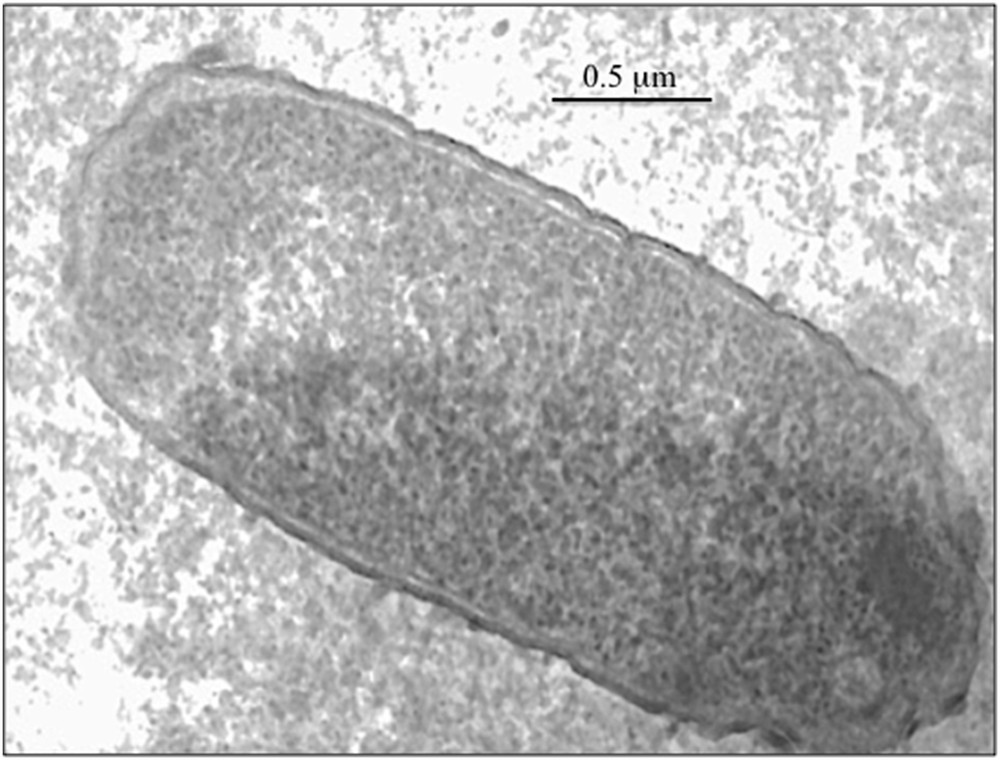
E. coli cell trapped in a silica gel (bar = 0.5 μm).
However, the viability of trapped bacteria is not very high. Only 40% of them remain viable after 1 month. Nevertheless their viability can be improved significantly by adding quorum sensing molecules. These molecules (homoserine lactone) are responsible for the chemical communication between bacteria. In a culture medium, they become active when the population increases and bacteria come close together to form a colony. We have shown that adding homoserine lactone to the nutrient solution improves the production of prodigiosin and increases significantly their lifetime. The plate count technique shows that almost 100% S. marcescens are still viable after 1 month in the gel. Sol–gel encapsulation could also provide useful information about the mechanism of bacterial quorum sensing chemical communication [15]. When trapped in a silica matrix, bacteria remain far apart so that there is no chemical communication between them. It is therefore possible to study how they respond to the addition of foreign molecules [16,17]. Such studies could provide useful information to fight against bacterial infections. Quorum quenching molecules that prevent chemical communication between bacteria could be used instead of antibiotics to prevent infection [18].
5 Multifunctional nanostructured biomaterials
In industry, both the structure and composition of advanced materials are controlled to optimize their properties. Boron, for instance, is added to the silicate composition of pyrex glasses to improve their thermal behavior. Lead is used to increase the reflective index of crystal glasses and Na to decrease their melting temperature. This is no longer possible with biomaterials made by living microorganisms. The same materials have to be used all along the synthesis but should exhibit several properties at the same time. Nature has to find a compromise, which is usually obtained via a careful control of their nanostructure. Diatom shells, for instance, have to be strong to protect the cell. But they should also be transparent to allow their photosynthetic activity. Therefore silica glasses are chosen instead of calcium carbonate used by most marine organisms. Moreover, trapped cells should be able to exchange with the outside medium to perform their metabolic activity. Therefore, diatom frustules are highly porous (Fig. 3). This is the main use of diatomaceous earths, widely used in industry for making filters. The well-known dynamite invented by A. Nobel was actually made with diatom earth impregnated with nitroglycerin.
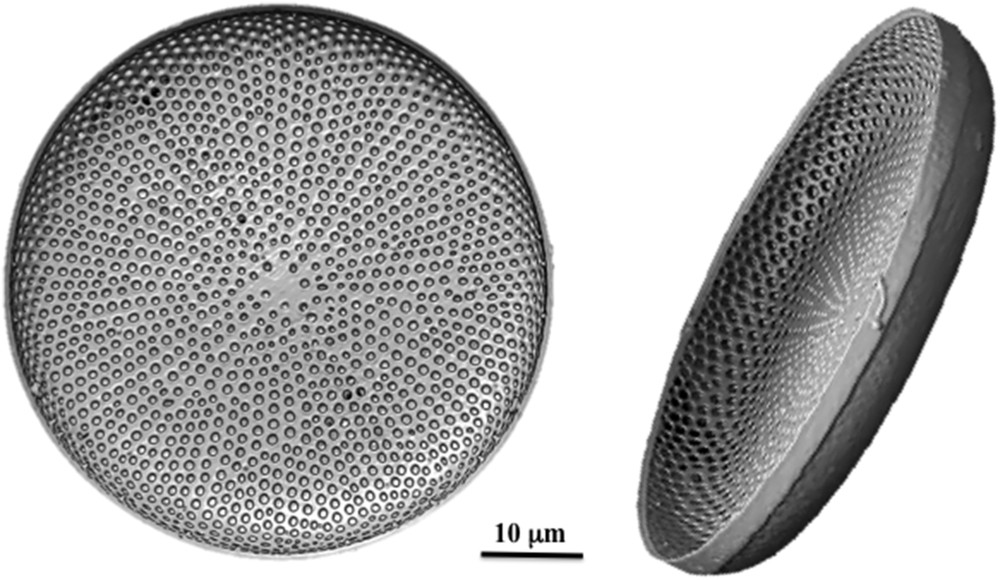
Both parts of the frustule of Coscinodiscus alesia diatoms are highly porous.
The most remarkable property of the porous shell of diatoms is that pores are not randomly distributed. Coscinodiscus diatoms, for instance, exhibit a highly nanostructured frustule. It is made of three overlapping silica layers containing, respectively, small (≈50 nm), medium (≈250 nm), and large (≈1 μm) pores (Fig. 4a).
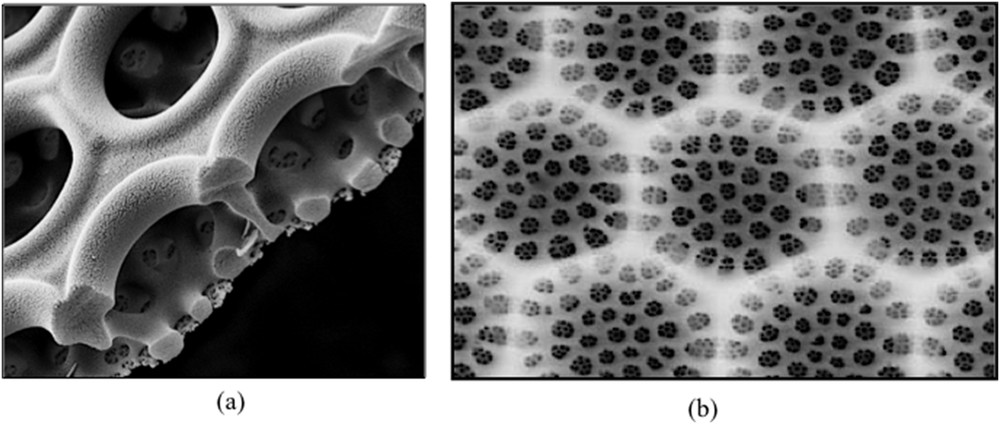
Pores of different sizes are distributed within three different layers (a). Diatoms exhibit photonic crystal properties because of the periodic distribution of pores within a silica layer (b).
Moreover, these pores exhibit a periodic distribution. They form a hexagonal array with a lattice parameter of about 0.6 μm leading to a periodic distribution of the refractive index (Fig. 4b). Diatom shells then behave as living photonic crystals. Visible light is focused inside the cell where photosynthesis occurs, whereas UV light is reflected. Frustules then behave as an optical filter protecting the cell against UV irradiation [19,20].
The nanostructured frustules of diatoms can be exploited for making a large variety of devices [21,22]. The photoluminescence properties of silica, for instance, have been used for gas detection (NO, NO2). The light focusing properties of diatoms can be used to focus solar light onto the “p–n” junction of a solar cell. Diatoms could also be described as living bioreactors. The chemical composition of their silica frustule can be modified by adding foreign elements in the culture medium. Adding Ge(OH)4, for instance, leads to Ge doped frustules that exhibit blue electroluminescence [23]. Interesting results have been obtained with dye sensitized solar cells. Adding titanium at the end of culture leads to the formation of a TiO2 layer at the surface of the frustules. A large interface between dyes and the porous electrodes is thus obtained, improving the energy conversion efficiency [24].
The whole chemical composition of diatom frustules can be changed without modifying their nanostructure via their magnesioisothermic reduction at relatively low temperatures, around 600 °C [25]. This so-called Bioclastic and Shape-preserving Inorganic Conversion process can be used to obtain other nanostructured ceramics such a BatiO3, ZrO2, and even BN.