Oximes and oxime ether functional groups are used in a large range of pharmaceutical molecules and are incorporated into many organic medicinal agents, including some antibiotics such as gemifloxacin mesylate I, pralidoxime chloride II and obidoxime chloride III (Scheme 1), which are used in the treatment of poisoning by organophosphate insecticides, malathion and diazinon [1]. Moreover, oximes are frequently used in synthetic organic chemistry [2]. Its synthetic utility is principally as an important precursor for numerous functional group transformations such as nitriles [3], nitro compounds [4], amines [5] and amides [6]. The conventional synthesis procedure for oximes is based on the reactions of hydroxylamine hydrochloride and corresponding aldehydes or ketones in an acidic or basic medium, and typically produces both isomeric oximes, E and Z [7]. However, some oxidative preparations of aldehydes or ketones are not desirable due to the presence of other readily oxidizable functional groups. In addition, the highly reactive nature of aldehydes and ketones makes them unsuitable for oxime-forming reactions [8].
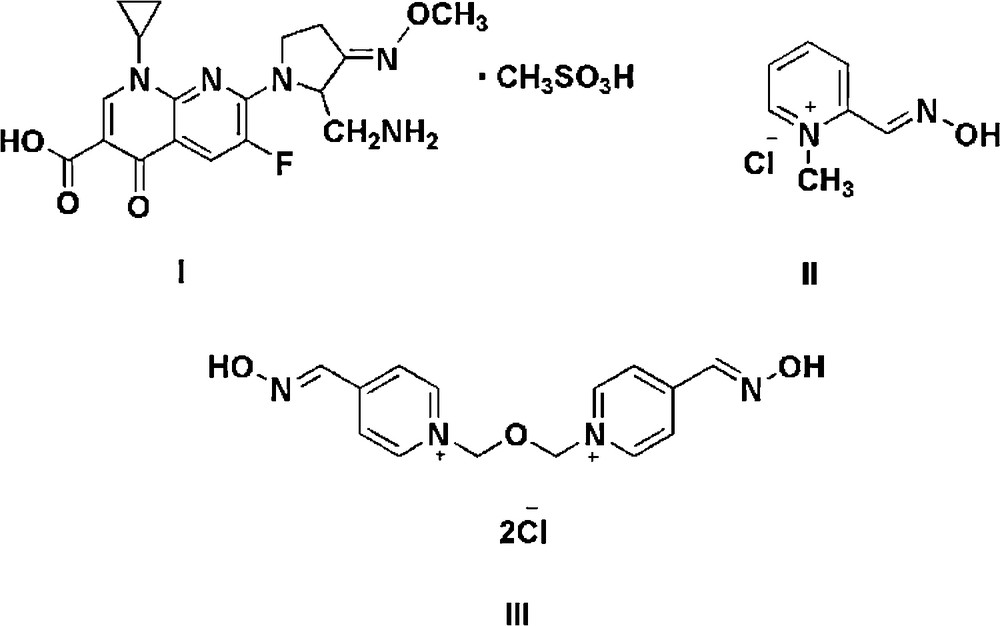
Task-specific ionic liquids (TSILs) have gained increasing interest in the context of green synthesis with their dual roles as catalysts and solvents (media) in recent years. Although ionic liquids were initially introduced as alternative green reaction media because of their unique chemical and physical properties (e.g. non-volatility, high thermal and chemical stability, good solvating capability, wide liquid range and ease of recycling), nowadays these ionic liquids have achieved success and show increasing promise in controlling the reaction as catalysts [9]. Furthermore, there are many reports concerning the applications of these organic salts in the literature such as cellulose dissolution [10], energetic materials [11], lubricants [12], fuel cells [13], solar cell [14], CO2 capture [15] and active pharmaceutical ingredients (APIs) [16].
According to the literature, very few improved procedures have been reported for the direct conversion of alcohols to oximes [5,8,17]. Thus, development of an efficient and eco-friendly method for a direct conversion of alcohols to oximes is desirable. In view of this fact and also as a part of our program to explore the full potential of TSILs in chemical transformations [18], an efficient, one-pot, environmentally-benign protocol for direct microwave-promoted conversion of a variety of alcohols to the corresponding oximes without going through aldehydes and ketones, using Brønsted acidic ionic liquid, 1-methylimidazolium nitrate [Hmim][NO3], as a promoter and medium is reported in this communication.
The general synthetic strategy for the preparation of various types of oximes is simple and versatile. The reaction of alcohols with hydroxylamine hydrochloride using [Hmim][NO3] in the presence of air and under microwave irradiation (Scheme 2) resulted in formation of the desired oximes. Brønsted acidic [Hmim][NO3] was prepared simply by neutralization. Combination of 1-methylimidazole with HNO3 (67% w/w) in a 1:1 molar ratio produces, after water removal, a white solid (Tm = 60 °C) identified as 1-methylimidazolium nitrate. This ionic liquid is air-stable, hydrophilic, easy to prepare and handle, and its physicochemical properties have been studied [19]. It has been used as a co-solvent and even as a promoter in the oxidative aromatic chlorination and has considerable potential as a reaction medium [20].

The reactions were carried out in a Micro–SYNTH labstation microwave system in an open vessel. During the experiments; power, temperature, time and pressure were monitored and controlled with the “easyCONTROL” software.1 Reaction of benzyl alcohol 1a and hydroxylamine hydrochloride 2 was selected as a model experiment in order to optimize the reaction conditions. The type of ionic liquids, as well as the effect of temperature and reaction time were investigated and the obtained results are illustrated in Table 1.
Synthesis of benzaldeheyde oxime under various experimental conditions.a.
Entry | Catalyst | Temp. (°C) | Time (min) | Conditions | Yield (%)d |
1 | No catalyst | 85 | 10 | MWb | 0 |
2 | HNO3 | 85 | 10 | MWb | 0 |
3 | [bmim][PF6] | 85 | 5 | MWb | 0 |
4 | [bmim][NTf2] | 85 | 5 | MWb | 0 |
5 | [bmim][Cl] | 85 | 5 | MWb | 0 |
6 | [Hmim][Cl] | 85 | 5 | MWb | 0 |
7 | [Hmim][HSO4] | 85 | 5 | MWb | 0 |
8 | [Hmim][NO3] | 80 | 2.5 | MWb | 95 |
9 | [Hmim][NO3] | 85 | 2.5 | MWb | 95 |
10 | [Hmim][NO3] | 75 | 2.5 | MWb | 89 |
11 | [Hmim][NO3] | 80 | 2 | MWb | 86 |
12 | [Hmim][NO3] | 80 | 3 | MWb | 95 |
13 | [Hmim][NO3] | 80 | 2.5 | CHc | 0 |
a Reaction conditions: Alcohol (1 mmol), hydroxylamine hydrochloride (1.3 mmol), [Hmim][NO3] (2 mL).
b Microwave irradiation (80 W).
c Conventional heating.
d Isolated yields.
The efficiency of the potential oxidative systems was studied in the model reaction. Experiments were carried out using nitric acid (Table 1, entry 2), different types of classic (Table 1, entries 3–5) and Brønsted acidic ionic liquids (Table 1, entries 6 to 13) in the presence of air and under microwave irradiation (80 W). It was found that [Hmim][NO3] was the most effective system, producing almost quantitative yields of corresponding oxime 3a (Table 1, entry 8). While the reaction occurred even at 85 °C, the best yield of benzaldehyde oxime 3a was obtained at 80 °C for 2.5 min under microwave irradiation (80 W) (Table 1, entry 9). Reducing the reaction temperature and time led to a reduction in the desired product's yield (Table 1, entries 10 and 11). In addition, over longer reaction times, an increase in the yield of benzaldehyde oxime was not observed (Table 1, entry 12). The role of microwave energy was also determined by conducting a model reaction at elevated temperature (80 °C) applying conventional heating conditions. As expected, this reaction failed to give the desired product and starting materials were recovered unchanged (Table 1, entry 13) possibly because of the strong synergic effect of the microwave energy and ionic liquid.
In order to explore the scope and versatility of [Hmim][NO3] ionic liquid, various types of structurally divergent primary and secondary alcohols 1 and hydroxylamine hydrochloride 2 (Scheme 2) underwent smooth microwave-promoted oxidation/oxime-forming reactions to afford the corresponding oximes 3a–n in excellent yields (85–99%) within short time periods (2.5–6 min).2 Results are summarized in Table 2. Benzyl-, allyl-, and propargyl alcohols are also good substrates for this reaction and their corresponding oximes were obtained in 95, 96, and 96% yields, respectively (Table 2, entries 1, 8 and 9). Secondary alcohols led to ketoximes in the same manner as aldoximes (Table 2, entries 3, 6 and 14). Similarly, this protocol is also applicable for the direct synthesis of oximes from hindered alcohols. For instance, 2’,4’,6’-trimethylacetophenone oxime can be easily prepared in 6 min under microwave irradiation (Table 2, entry 6), while this reaction required a very long time, 32 days, for completion using hindered ketones at room temperature [7c]. No cis- and trans-isomerization of the double bond was observed in a cis-α,β-unsaturated alcohol (Table 2, entry 8). Furthermore, the putative avoidance of carbonyl intermediates makes this method effective for the preparation of β,γ-unsaturated oxime (Table 2, entry 10). It is also noteworthy that in the conversion of alcohols bearing acid-sensitive functional groups to the corresponding oximes, epoxide ring-opening (Table 2, entry 11), acetal deprotection (Table 2, entry 13) and ester hydrolysis (Table 2, entry 14) reactions were not observed. As illustrated in Table 2, a preference for the formation of Z isomer over the E isomer was observed using this synthetic methodology and literature reports also show this tendency [5,8,17].
Microwave-promoted synthesis of oximes by oxidation/oxime-forming reaction using [Hmim][NO3] as a catalyst-medium.a
Entry | Alcohol | Oxime | Time (min) | Yield (%)b | E/Z (%)c | |
1 | 3a | 2.5 | 95 | 0/100 | ||
2 | 3b | 3 | 97 | 25/75 | ||
3 | 3c | 5 | 89 | 0/100 | ||
4 | 3d | 3 | 93 | 12/88 | ||
5 | 3e | 2.5 | 95 | 0/100 | ||
6 | 3f | 6 | 85 | 0/100 | ||
7 | 3g | 2.5 | 99 | 35/75 | ||
8 | 3h | 2.5 | 96 | 34/76 | ||
9 | 3i | 2.5 | 96 | 31/69 | ||
10 | 3j | 3 | 97 | 30/70 | ||
11 | 3k | 3 | 97 | 41/59 | ||
12 | 3l | 3 | 94 | 44/56 | ||
13 | 3m | 4.5 | 92 | 39/61 | ||
14 | 3n | 4 | 94 | 0/100 |
a Reaction conditions: alcohol (1 mmol), hydroxylamine hydrochloride (1.3 mmol), [Hmim][NO3] (2 mL), MW power (80 W).
b Isolated yield.
c Separated by column chromatography.
To further expand of the scope of [Hmim][NO3], the reusability of this ionic liquid was studied with the model reaction. The ionic liquid could be recovered and reused over the three runs with only limited reduction (80–95%) in the catalytic activity of the ionic liquid.
In summary, we have reported herein a microwave-promoted, efficient, environmentally-benign and one-pot conversion of alcohols to corresponding oximes using [Hmim][NO3] as a solvent-promoter (or catalyst). This present protocol offers attractive features such as reduced reaction times, high yields and economic viability of the ionic liquid.
1 The microwave system used in these experiments includes the following items: Micro-SYNTH labstation, equipped with a glass door, a dual magnetron system with pyramid shaped diffuser, 1000 W delivered power, exhaust system, magnetic stirrer, “quality pressure” sensor for flammable organic solvents, and ATC-FO fiber optic sensor TS3517 for automatic temperature control.
2 Z isomer: white crystalline solid, Tm = 74–75 °C (lit. [8] 72.0–72.8 °C); IR (neat, cm-1): νmax 3244, 1739, 1461, 1326, 1244, 1175, 1043; 1H NMR (CDCl3, 400 MHz) δH 3.89 (s, 3H), 2.22 (s, 3H); 13C NMR (CDCl3, 100 MHz) δC 164.8, 152.3, 55.7, 10.9.