1 Introduction
Increasing attention has been paid to the organopalladium complexes containing a Pd–Pd bond [1]. The coordination behavior or transformation of unsaturated hydrocarbons at a dinuclear palladium center has been investigated by employing reactive PdI–PdI complexes [1]. Representatively, the homoleptic nitrile dipalladium(I) complexes [Pd2(CH3CN)6][X]2 (1, X = BF4; 1′, X = PF6) have been developed as the substitutionally labile Pd–Pd complexes for studying coordination modes and reaction patterns of unsaturated substrates at a dinuclear palladium center [2,3]. By employing 1, indeed, several new coordination modes or reaction patterns have been recently disclosed in our laboratory, such as dinuclear palladation of arenes [4] and the bridging π-coordination of pyrrole and indole [5]. Agapie et al. reported the bridging π-coordination of thiophene and furan to a diphosphine-arene supported PdI–PdI moiety [6]. Here, we report the sandwich-type bridging π-coordination of furan and toluene to a dicationic PdI–PdI moiety.
2 Results and discussion
The coordination behavior of weakly coordinating organic substrates such as arenes and hetero-arenes to a PdI–PdI moiety may be addressable when highly substitutionally labile PdI–PdI complexes are used as the starting materials. We recently reported the bridging π-coordination of pyrrole or indole to a dicationic PdI–PdI moiety derived from [Pd2(CH3CN)6][BF4]2 (1) [5]. The resultant μ-pyrrole Pd2 complex [Pd2(μ-pyrrole)2(CH3CN)2][BF4]2 (2) or μ-indole Pd2 complex [Pd2(μ-indole)2(CH3CN)2][BF4]2 (3) are stable in solution at ambient temperature, while the π-adducts of non-chelating, parent pyrrole or indole to mononuclear PdII or Pd0 moieties have not been isolated so far. As a part of our study on the bridging π-coordination of weakly coordinating arenes and hetero-arenes [4,5], here, we employed furan as the bridging substrate. Addition of furan (2 equiv) to 1 in CD3NO2 at ambient temperature showed no sign of furan coordination in the 1H NMR spectra. However, precipitation from the solution of 1 or 1′ in CH3NO2 in the presence of a large excess of furan gave the bis-furan sandwich complex [Pd2(μ-furan)2(CH3CN)2][X]2 (4, X = BF4; 4′, X = PF6) (Eq. 1). A single crystal of 4 suitable for X-ray structure analysis was obtained through vapor diffusion of Et2O into a CH3NO2 solution at ambient temperature. The molecular structure of 4 was shown in Fig. 1. The furan ligands coordinate to a PdI–PdI moiety via μ-η2:η2 bridging mode in a mutually staggered arrangement (st-4). The Pd–Pd bond length (2.486(2) Å) is in the range of normal PdI–PdI lengths [1a], and is similar to that of the bis-pyrrole Pd2 complex 2 (Pd–Pd = 2.4783(9) Å) [5]. A longer Pd–Pd bond was reported in a 2-methylfuran-bridged Pd2 complex [Pd2(μ-2-methylfuran)(μ-terphenyldiphosphine)][BF4]2 (Pd–Pd = 2.6518(9) Å), where the backside bridging ligand is the μ-η2:η2-arene ring [6].
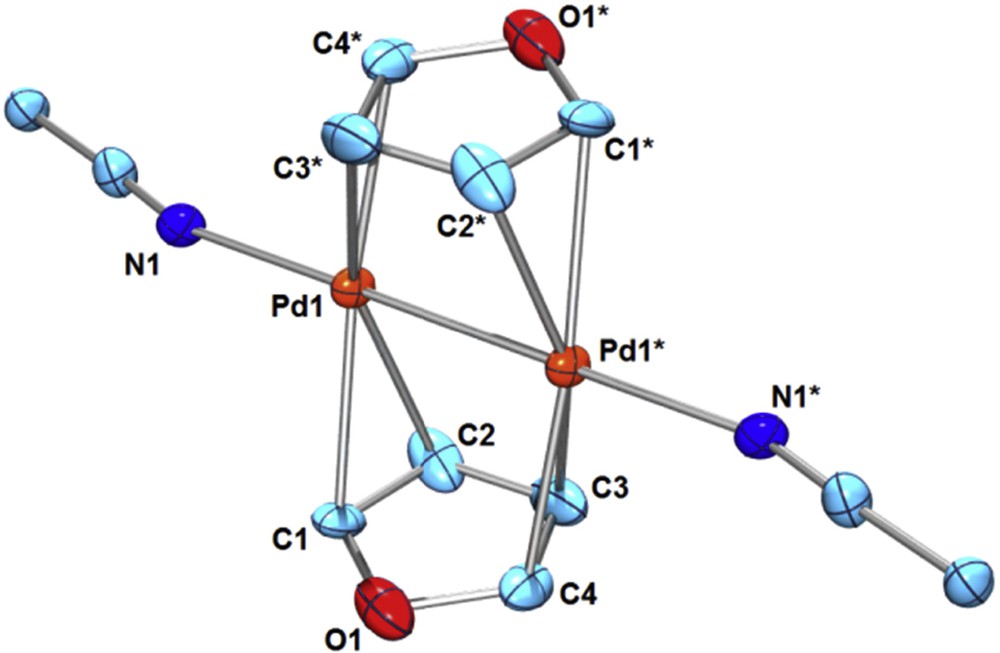
(Color online.) ORTEP of [Pd2(μ-furan)2(CH3CN)2][BF4]2 (4) (30% probability ellipsoids; hydrogen atoms and counter anions are omitted for clarity. Selected bond lengths (Å): Pd1–Pd1* 2.486(2), Pd1–C1 2.28(3), Pd1–C2 2.27(2), Pd1–C3* 2.31(2), Pd1–C4* 2.25(2), Pd1–N1 2.13(2), C1–C2 1.30(4), C2–C3 1.42(3), C3–C4 1.31(4), C4–O1 1.39(3), O1–C1 1.36(2).
The 1H NMR spectra of 4 in a mixed solvent CD2Cl2-CD3NO2 at 25 °C exhibited two broad resonances for the furan protons at δH2 = 8.57 ppm (2-position) and δH3 = 6.98 ppm (3-position). Lowering the temperature down to −50 °C caused each resonance to decoalesce to two resonances, indicating a rapid equilibrium between two isomers (major/minor = 62/38; for major isomer, δH2 = 8.63 ppm and δH3 = 6.88 ppm; for minor isomer, δH2 = 8.19 ppm and δH3 = 6.95 ppm). There are two possible isomers for the μ-η2:η2-furan sandwich, i.e. a staggered arrangement (st-4) and an eclipsed arrangement (ec-4), where the former was identified in the crystalline state. In solution, presumably, these two possible isomers st-4 and ec-4 exist in equilibrium (Eq. 2). The bis-furan dipalladium complex 4 gradually decomposed in CD3NO2 at ambient temperature.
We confirmed that the bridging furan ligands in 4 or 4′ are easily replaced with toluene or pyrrole. Addition of large excess toluene to a CH3NO2 solution of 4 or 4′ afforded the bis-toluene dipalladium(I) complex [Pd2(μ-toluene)2(CH3CN)2][X]2 (5, X = BF4; 5′, X = PF6) (Eq. 3). A related dicationic bis-benzene dipalladium complex [Pd2(μ-benzene)2(CH3CN)2][BF4]2 was recently prepared directly from 1 in the presence of a large excess of benzene [4], but its structure has not been determined by X-ray structural analysis. A single crystal of 5 or 5′ suitable for X-ray analysis was obtained by recrystallization from CH3NO2/toluene at –30 °C. The molecular structure of 5′ is shown in Fig. 2. The two toluene ligands coordinate to a Pd–Pd moiety (Pd–Pd = 2.5651(4) Å) in a μ-η2(C1–C2):η2(C3–C4) manner (Fig. 2). The related neutral bis-toluene Pd2 sandwich complexes, Pd2(μ-toluene)2(GaCl4)2 (6), Pd2(μ-toluene)2(GaBr4)2 (7), and Pd2(μ-toluene)2(Ga2Cl7)2 (8), were structurally characterized by Kloo et al.; in each case, the coordination mode of toluene is in a μ-η2(C1–C2):η2(C3–C4) mode or a μ-η2(C1–C2):η2(C4–C5) mode [7–9]. Concerning the arene rings, uncoordinated C5–C6 (1.379(5) Å) is considerably shorter than other C–C ring bonds (C1–C2, C2–C3, C3–C4, C4–C5, and C1–C6 are in the range of 1.406(5) Å–1.429(7) Å). 13C NMR spectra of 5 in CD3NO2 showed the averaged resonance for the ortho- or meta-carbons at 25 °C (δ = 112 ppm for ortho-carbons, δ = 106 ppm for meta-carbons). The 13C NMR resonance pattern for the toluene ligands did not change, even at lower temperature down to –20 °C in a mixed solvent CD3NO2–CD2Cl2, indicating the rapid fluxional behavior of the μ-toluene ligands in solution. The bis-toluene dipalladium complex 5 decomposed gradually in CD3NO2 solution at ambient temperature.

(Color online.) (A) ORTEP of [Pd2(μ-toluene)2(CH3CN)2][PF6]2 (5′) (50% probability ellipsoids; hydrogen atoms and counter anions omitted for clarity). Selected bond lengths (Å): Pd1–Pd1* 2.5651(4), Pd1–C1 2.227(4), Pd1–C2 2.383(4), Pd1*–C3 2.276(4), Pd1*–C4 2.273(4), Pd1–N1 2.099(3), C1–C2 1.412(7), C2–C3 1.406(5), C3–C4 1.422(7), C4–C5 1.421(7), C5–C6 1.379(5), C6–C1 1.429(7), C6–C7 1.505(7). (B) Ball-and-stick drawing of 5′ showing the positions of Pd atoms on the toluene ligands.
Treatment of the bis-furan dipalladium complex 4 with pyrrole (2 equiv) in CD3NO2 at ambient temperature afforded the bis-pyrrole sandwich complex [Pd2(μ-pyrrole)2(CH3CN)2][BF4]2 (2) [5] almost quantitatively, where the volatiles including furan were readily removed by the evaporation (Eq. 4).
3 Conclusion
In summary, the dicationic bis-furan dipalladium(I) complexes were isolated and structurally characterized. The bridging furan ligands in the dipalladium complexes are readily replaced with toluene or pyrrole. The dicationic bis-toluene dipalladium(I) complex was also structurally characterized. It is expected that the bis-furan dipalladium(I) complexes can be used as useful starting materials for the synthesis of di- and polypalladium clusters, due to its substitutionally labile nature as well as the easy removal of the volatile furan after ligand substitution reactions.
4 Experimental
4.1 General procedures
All manipulations were conducted under a nitrogen atmosphere using standard Schlenk or dry-box technique. 1H (400 MHz) and 13C{1H} (100 MHz) NMR spectra were recorded using a JEOL JNM-ECS400 apparatus. The chemical shifts were referenced to the residual resonances of deuterated solvents. Elemental analyses were performed at the Instrument Center of Institute for Molecular Science. X-ray crystal data were collected by Rigaku R-AXIS RAPID diffractometer or Rigaku Saturn CCD area detector, with graphite-monochromated Mo Kα (0.71075 Å) radiation. Unless specified, all reagents were purchased from a commercial supplier and used without purification. Nitromethane, acetonitrile, diethyl ether, dichloromethane, n-hexane, furan, toluene, CD3NO2, and CD2Cl2 were purified according to standard procedures. [Pd2(CH3CN)6][BF4]2 [2a] and [Pd2(CH3CN)6][PF6]2 [3c] was prepared according to the literature.
4.2 Synthesis of [Pd2(μ2-furan)2(CH3CN)2][BF4]2 (4)
To a solution of [Pd2(CH3CN)6][BF4]2 (52.5 mg, 83.0 μmol) in CH3NO2 was added a large excess of furan, and the resultant yellow precipitates were collected and dried in vacuo to afford 4 (41.1 mg, 82% yield). The complex 4 was crystallized from by using vapor diffusion method with CH3NO2/furan. 1H NMR (400 MHz, CD3NO2, 25 °C) δ 8.57 (s, 4H, H1), 6.98 (s, 4H, H2), 2.45 (s, 6H, H3). 13C{1H} NMR (100.5 MHz, CD3NO2, 25 °C) δ 130.8 (s, C1), 125.4 (s, C4), 92.4 (s, C4), 3.02 (s, C3). Anal. calcd. for: C12H14B2F8O2N2Pd2: C, 23.83; H, 2.33; N, 4.63. Found: C, 24.33; H, 2.50; N, 4.71.
4.3 Synthesis of [Pd2(μ2-furan)2(CH3CN)2][PF6]2 (4′)
To a solution of [Pd2(CH3CN)6][PF6]2 (312 mg, 416 μmol) in CH3NO2 was added a large excess of furan, and the resultant yellow precipitates were collected and dried in vacuo to afford 4′ (252 mg, 84% yield). 1H NMR (400 MHz, CD3NO2, 25 °C) δ 8.55 (s, 4H, H1), 6.97 (s, 4H, H2), 2.45 (s, 6H, H3). 13C{1H} NMR (100.5 MHz, CD3NO2, 25 °C) δ 130.5 (s, C1), 125.3 (s, C4), 92.1 (s,C2), 2.99 (s, C3). Anal. calcd for C12H14P2F12O2N2Pd2: C, 19.99; H, 1.96; N, 3.89. Found: C, 20.04; H, 2.10; N, 4.03.
4.4 Synthesis of [Pd2(μ2-toluene)2(CH3CN)2][BF4]2 (5)
To a solution of 4 (54.5 mg, 90.1 μmol) in CH3NO2 was added a large excess of toluene, and the mixture solution was evaporated in vacuo. This treatment was repeated seven times to afford a brown powder of complex 5. Recrystallization from CH3NO2/toluene at −30 °C gave brown crystals of complex 5 (36.8 mg, 63% yield). 1H NMR (400 MHz, CD3NO2, 25 °C) δ 7.21 (brs, 8H, H2 and H3), 6.96 (brs, 2H, H1), 2.55 (s, 6H, H6), 2.34 (s, 6H, H5). 13C{1H} NMR (100.5 MHz, CD3NO2, 25 °C) δ 143.0 (s, C4), 125.9 (s, C7), 112.1 (s, C3), 108.1 (s, C1), 106.4 (s, C2), 21.9 (s, C5), 3.40 (s, C6). Anal. Calcd. For. C18H22B2F8N2Pd2: C, 33.12; H, 3.40; N, 4.29. Found: C, 31.10; H, 3.67; N, 4.89.
4.5 Synthesis of [Pd2(μ2-toluene)2(CH3CN)2][PF6]2 (5′)
To a solution of 4′ (56.0 mg, 77.7 μmol) in CH3NO2 was added a large excess of toluene, and the mixture solution was evaporated. This treatment was repeated ten times to afford a brown powder of 5′. Recrystallization from CH3NO2/toluene at −30 °C gave brown crystals of complex 5′ (23.9 mg, 40%). 1H NMR (400 MHz, CD3NO2, 25 °C) δ 7.21 (brs, 8H, H2 and H3), 6.95 (brs, 2H, H1), 2.56 (s, 6H, H6), 2.34 (s, 6H, H5). 13C{1H} NMR (100.5 MHz, CD3NO2, 25 °C) δ 143.0 (s, C4), 125.8 (s, C7), 112.1 (s, C3), 108.1 (s, C1), 106.4 (s, C2), 21.9 (s, C5), 3.39 (s, C6). Anal. Calcd. For. C18H22P2F12N2Pd2: C, 28.10; H,2.88; N,3.64. Found: C, 26.66; H, 2.79; N, 4.00.
4.6 X-ray crystallography analyses
Crystals were mounted on a CryoLoop (Hampton Research Corp.) with a layer of paraton-N oil and placed in a nitrogen stream at 103(2) or 123(2) K. All measurement were performed on a R-AXIS RAPID II Imaging Plate or a Rigaku Saturn CCD area detector with graphite-monochromated Mo Kα (0.71075 Å) radiation. The structure was solved by direct methods (SIR92 [10] or SHELXS2013 [11]) and refined on F2 by full-matrix least-squares methods; using SHELXL2013 [11]. Non-hydrogen atoms were anisotropically refined. H-atoms were included in the refinement on calculated positions riding on their carrier atoms. The function minimized was , where , with from counting statistics. The functions R1 and wR2 were , respectively. The ORTEP-3 program was used to draw the molecules [12].
Crystal data for [Pd2(furan)2(CH3CN)2][BF4]2: C12H14B2F8N2O2Pd2, Mr = 604.66, triclinic, space group P (No. 2), a = 7.5299(13) Å, b = 7.8934(14) Å, c = 9.0589(18) Å, α = 62.437(6)°, β = 69.793(5)°, γ = 88.319(5)°, Z = 1, V = 442.39(14) Å3, F(000) = 290, Dc = 2.269 g cm−3, μ(Mo Kα) = 21.25 cm−1, T = 123 K, 10,546 reflections collected, 2027 unique (Rint = 0.0818), 128 variables refined with 2027 reflections with I > 2σ(I) to R1 = 0.0986. CCDC 1042194.
Crystal data for [Pd2(toluene)2(CH3CN)2][BF4]2: C18H22B2F8N2Pd2, Mr = 652.79, triclinic, space group P (No. 2), a = 7.0269(4) Å, b = 7.6162(5) Å, c = 11.5979(7) Å, α = 76.4805(12)°, β = 72.4127(14)°, γ = 73.3492(14)°, Z = 1, V = 559.47(6) Å3, F(000) = 318, Dc = 1.937 g cm−3, μ(Mo Kα) = 16.82 cm−1, T = 123 K, 12,864 reflections collected, 2541 unique (Rint = 0.0461), 145 variables refined with 2541 reflections with I > 2σ(I) to R1 = 0.0702. CCDC 1042195.
Crystal data for [Pd2(toluene)2(CH3CN)2][PF6]2: C18H22F12N2P2Pd2, Mr =769.11, triclinic, space group P (No. 2), a = 7.9072(5) Å, b = 8.8810(6) Å, c = 9.7005(7) Å, α = 111.8489(19)°, β = 94.193(2)°, γ = 109.4019(17)°, Z = 1, V = 581.11(7) Å3, F(000) = 374, Dc = 2.198 g cm−3, μ(Mo Kα) = 17.94 cm−1, T = 103 K, 5697 reflections collected, 2645 unique (Rint = 0.0335), 165 variables refined with 2645 reflections with I > 2σ(I) to R1 = 0.0353. CCDC 1042196.
Acknowledgment
This work was supported by Ministry of Education, Science, Sports, and Technology (MEXT) Japan, the Japan Society for the Promotion of Science (JSPS), and Institute for Molecular Science.
Appendix A Supplementary data
CCDC 1042194–1042196 contain the supplementary crystallographic data for 4, 5, and 5′. Data can be obtained free of charge from The Cambridge Crystallographic Data Centre via www.ccdc.cam.ac.uk/data_request/cif.