1 Introduction
Over the recent decades, the catalytic application of nanoparticles (NPs) is of importance because of their high specific surface area leading to increase in the collision between reactants and catalyst, maximize the reaction rates, and reduce consumption of the catalyst [1]. However, the nanocatalysts have several advantages over conventional catalyst systems but the isolation and recovery of these nanocatalysts from the reaction medium is difficult and time-consuming. One of the best solutions to overcome this problem is the use of magnetically recoverable nanocatalysts [2], which can be separated by magnetic decantation after the reaction, therefore avoiding traditional filtration processes. Besides, most of these catalysts are recyclable [3–7]. Fe3O4 magnetic nanoparticles (MNPs) are one of the well-known magnetic materials, which have attracted more attention because they are easy to prepare, environmentally benign, economic, rather biocompatible, and easily separated from the reaction media using an external magnetic field [8]. Fe3O4 NPs have been applied in various organic transformations such as regioselective synthesis of propargylic amines [9], synthesis of α-aminonitriles [10], synthesis of quinoxalines [11], Sonogashira–Hagihara reaction [12], and N-protection of amines [13]. In addition, Fe3O4 MNPs have been used as a heterogeneous catalyst in the Fenton and Fenton-like processes [14,15].
Fenton process is a method that results in the formation of HO by a reaction between H2O2 as an oxidant and Fe2+/Fe3+ ions as a catalyst (Scheme 1) [16].

Fenton process.
The produced free radicals, HO, are strong oxidant agents for organic compounds [16,17].
Keeping the above facts in mind and based on our previous work about application of magnetically reusable nanocatalysts in organic synthesis [18–20], we have synthesized Fe3O4 MNPs and used it as an effective heterogeneous catalyst for the oxidation of thiols to sulfonic acids and sulfides to sulfoxides and sulfones using hydrogen peroxide as an inexpensive and environmental oxidant.
Sulfonic acids and their derivatives have many applications such as dyes, surfactants, pesticides, animal feeds, pharmaceuticals, and catalysts. Many aliphatic sulfonic acid derivatives show pharmacological activity, as well as several aromatic sulfonic acids have many medicinal uses and are suitable for treatment of a range of infections [21]. On the other hand, sulfoxides and sulfones are versatile intermediates and precursors in the preparation of fine chemicals and pharmaceuticals such as antifungal and antihypertensive agents [22]. Sulfoxides are also important in CC bond construction and molecular rearrangements [23].
2 Experimental section
2.1 General
All substances were purchased from the Merck and Aldrich Chemical Companies and used without purification. Products were characterized by assessment of their physical and spectral data with those of authentic samples. Fourier transform infrared (FTIR) and NMR spectra were recorded using Thermo Nicolet Nexus 670 FTIR and Bruker NMR spectrometers (1H NMR 250.1 MHz, 13C NMR 69.1 MHz), respectively. The X-ray powder diffraction data were collected using an X'Pert MPD Philips diffractometer with Cu Kα radiation source (λ = 1.54050 Å) at 40 kV voltage and 40 mA current. The particle morphology was determined by scanning electron microscopy (SEM) using FESEM-TESCAN MIRA3. Magnetic properties of the samples were obtained using a vibrating sample magnetometer (VSM, model MDKFT) under magnetic fields up to 20 kOe.
2.2 General procedure for the preparation of Fe3O4 MNPS
In a typical synthesis, FeCl3·6H2O (5.838 g, 0.0216 mol) and FeCl2·4H2O (2.147 g, 0.0108 mol) were sonicated in deionized water (100 mL) for 30 min. Afterward, NH4OH (25%, 10 mL) was added to the reaction mixture under N2 atmosphere at 85 °C. The reaction continued for another 25 min. After cooling to room temperature, the NPs were then separated from the reaction mixture using an external magnetic field, washed with doubly distilled water and NaCl solution (0.02 M), and dried at room temperature [24].
2.3 General procedure for oxidation of thiols to sulfonic acids
In a round-bottomed flask (25 mL) equipped with a magnetic stirrer, a mixture of thiol (1 mmol) and Fe3O4 MNPs (0.025 g) in CH3CN or EtOH was prepared. Next, H2O2 (10 mmol, 2 g) was added to the mixture and stirred under reflux conditions. After completion of the reaction as judged by thin-layer chromatography (eluent, n-hexane/EtOAc, 1/1), the catalyst was separated using an external magnetic field. Then, the mixture of the reaction was extracted with EtOAc (3 × 8 mL) and the organic layer dried over anhydrous Na2SO4. Evaporation of the solvent affords the pure sulfonic acid.
2.4 General procedure for the oxidation of sulfides to sulfoxides or sulfones
Fe3O4 MNPs (0.01 or 0.025 g) were added to a mixture of sulfide (1 mmol) and 30% H2O2 (10 or 20 mmol) in EtOH (2.5 mL). Then, the mixture was stirred at room temperature for an appropriate time. Thin-layer chromatography monitored the progress of the reaction. After completion of the reaction, the solvent was removed under reduced pressure to give the corresponding pure sulfoxide or sulfones in most cases.
3 Results and discussion
3.1 Catalyst preparation
Fe3O4 NPs were prepared via chemical coprecipitation of FeCl3·6H2O and FeCl2·4H2O in aqueous ammonia as reported in the literature (Scheme 2) [24].

Synthesis of Fe3O4 MNPs.
The nanocatalyst was characterized by X-ray diffraction (XRD), VSM, FTIR spectroscopy, and SEM.
3.2 Catalyst characterizations
The size and morphology of Fe3O4 NPs were measured by SEM analysis (Fig. 1).
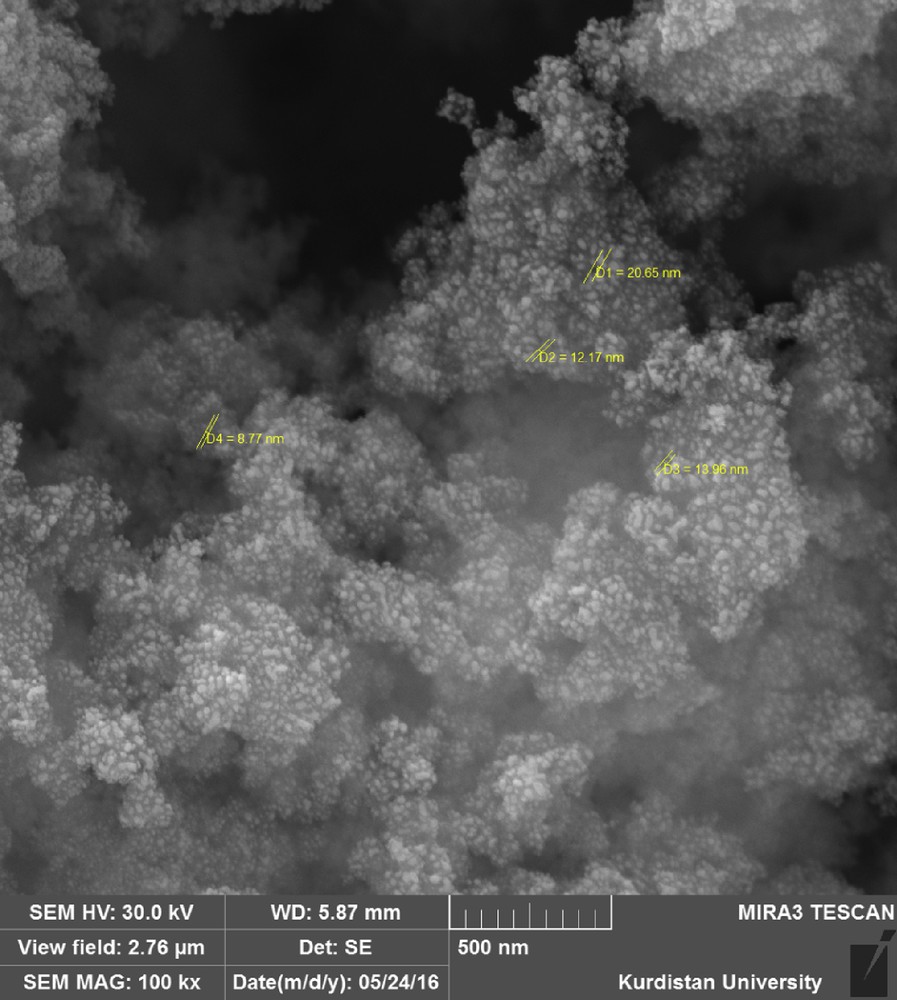
SEM image of Fe3O4 MNPs.
XRD pattern was applied to show that Fe3O4 MNPs were properly synthesized. XRD patterns of these NPs are shown in Fig. 2, reveal peaks at 2θ = 30.248, 35.628, 43.338, 53.638, 57.298, and 62.808, respectively, which are corresponding to the peaks of pure Fe3O4 (JCPDS 01-1111).
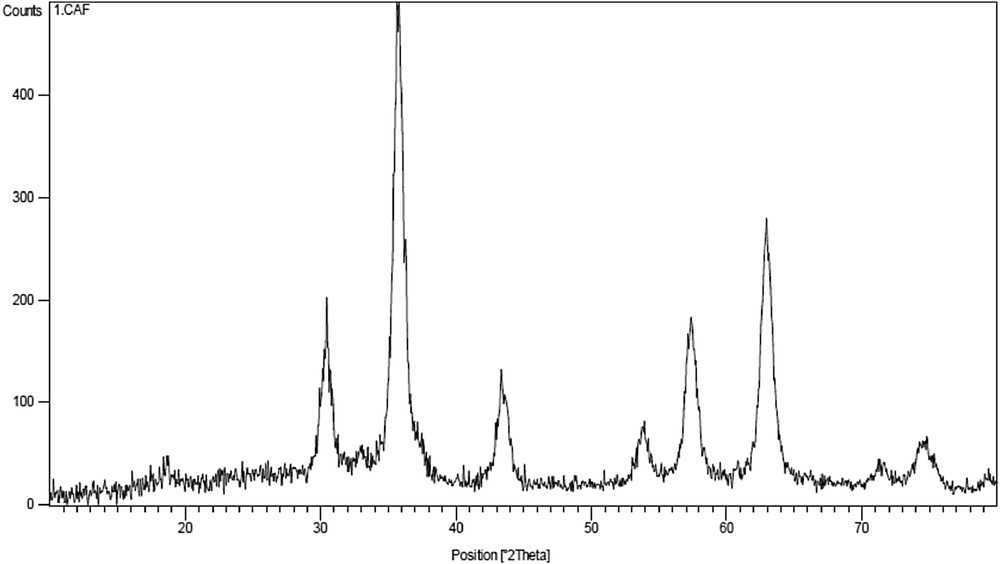
The XRD pattern of Fe3O4 MNPs.
The FTIR spectrum of Fe3O4 MNPs as shown in Fig. 3a, indicates a band at 3420 cm−1, which associated to stretching vibrations of the OH bonds on the surface of iron atoms. Also, the band at 571 cm−1 relates to vibrations of FeO bonds of iron oxide [25]. The magnetic property of the catalyst was determined by the VSM at 298 K (Fig. 3b). The magnetization curves revealed that the saturation magnetization (Ms) was 66.976 emu g−1 at an applied field of 10,000 Oe and showed superparamagnetic property of the catalyst at room temperature.
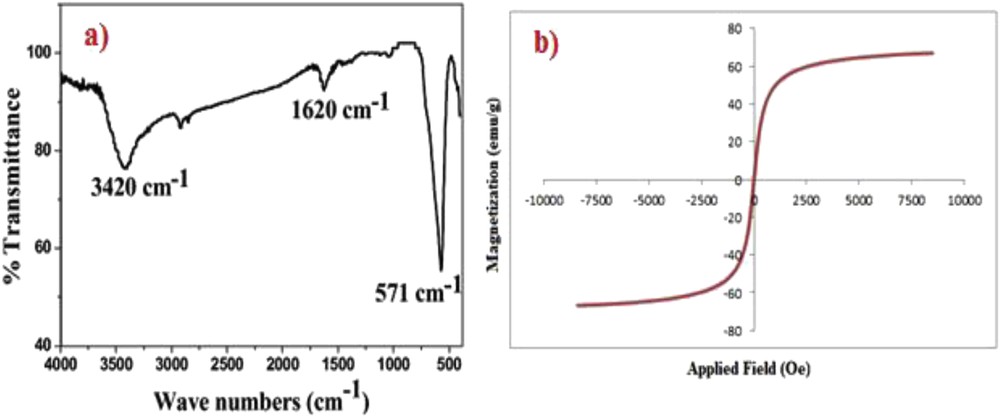
a) FTIR of Fe3O4 MNPs. (b) Magnetization curve of Fe3O4 MNPs.
3.3 Catalytic activity
To optimize reaction conditions for the oxidation of thiols to sulfonic acids, we surveyed the influence of different parameters, for example, solvent, temperature, and different amounts of Fe3O4 MNPs on the oxidation of thiophenol to benzenesulfonic acid as a model reaction (Table 1). As shown in Table 1, the best conditions were obtained in the presence of Fe3O4 MNPs (0.025 mg) using H2O2 (30%, 10 mmol, 2 g) in CH3CN under reflux conditions (Table 1, entry 9).
Optimization of the reaction conditions with respect to the effect of solvent, temperature, and the amounts of catalyst on the oxidation reaction of thiophenol to benzenesulfonic acid.a
Entry | Fe3O4 (g) | Solvent (mL) | Temperature (°C) | Time | Yield (%) |
1 | 0.01 | CH3CN | Reflux | 150 min | 40 |
2 | 0.015 | CH3CN | Reflux | 150 min | 55 |
3 | 0.02 | CH3CN | Reflux | 150 min | 65 |
4 | 0.025 | CH3CN | Reflux | 150 min | 83 |
5 | 0.025 | CH3CN | rt | 22 h | 70 |
6 | 0.025 | CH3CN | 45 | 14 h | 70 |
7 | 0.025 | CH3CN | 55 | 9 h | 75 |
8 | 0.025 | CH3CN | 65 | 5 h | 83 |
9 | 0.025 | CH3CN | Reflux | 150 min | 83 |
10 | 0.025 | n-Hexane | Reflux | 150 min | – |
11 | 0.025 | Et2O | Reflux | 150 min | – |
12 | 0.025 | CH2Cl2 | Reflux | 150 min | – |
13 | 0.025 | CHCl3 | Reflux | 150 min | – |
14 | 0.025 | DMF | Reflux | 150 min | 30 |
15 | 0.025 | EtOH | Reflux | 150 min | 45 |
16 | 0.025 | H2O/EtOH | Reflux | 300 min | 83 |
a Reaction conditions: thiophenol (1 mmol), H2O2 (30%, 10 mmol, 2 g), solvent (2.5 mL).
We also investigated the effect of solvent on the oxidation of thiosalicylic acid as another thiolic compound with different polarity. The results of these experiments are presented in Table 2.
The effect of solvent on the oxidation of thiosalicylic acid to 2-sulfobenzoic acid using Fe3O4/H2O2system.a
Entry | Fe3O4 (g) | Solvent (mL) | Temperature (°C) | Time (min) | Yield (%) |
1 | 0.025 | n-Hexane | Reflux | 150 | – |
2 | 0.025 | Et2O | Reflux | 150 | – |
3 | 0.025 | CH2Cl2 | Reflux | 150 | – |
4 | 0.025 | CHCl3 | Reflux | 150 | – |
5 | 0.025 | DMF | Reflux | 150 | 60 |
6 | 0.025 | EtOH | Reflux | 150 | 90 |
7 | 0.025 | H2O/EtOH | Reflux | 300 | 90 |
8 | 0.025 | CH3CN | Reflux | 150 | 90 |
a Reaction conditions: thiosalicylic acid (1 mmol), H2O2 (30%, 10 mmol, 2 g), solvent (2.5 mL).
The results presented in Tables 1 and 2 show that the solvent plays an important role in the type and yield of the product. In nonpolar solvents (immiscible in water), the reaction proceeds to coupling of thiols or one of the intermediates (Scheme 3) [26,27] because oxidants and thiols are located in two different phases and the possibility of the collision is low. However, in the presence of the solvents that are miscible in water and are capable of dissolving the thiols and other intermediates, the reaction has gone to the considered product because oxidant, thiols, and its intermediates are in the same phase.
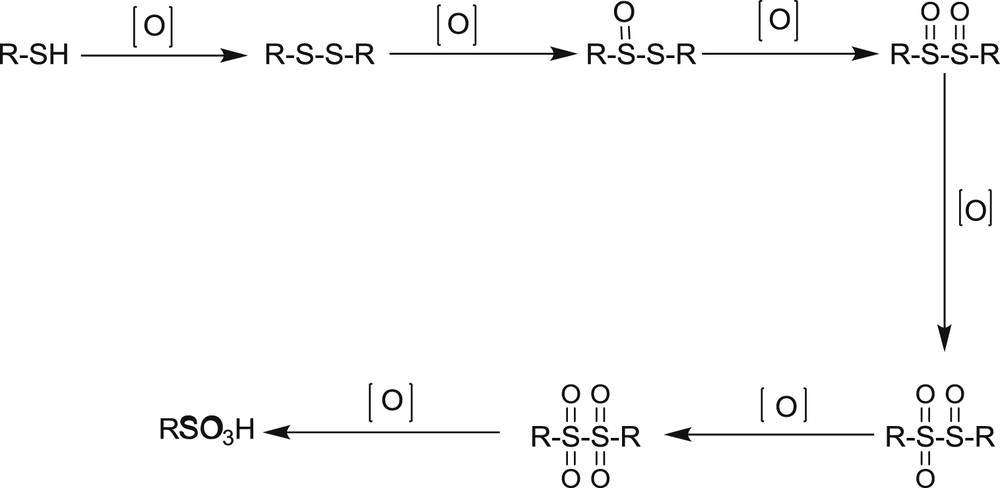
The oxidation steps of thiols to sulfonic acids.
The best results for the oxidation of thiophenol in terms of reaction time and the product yield were attained in CH3CN, whereas for oxidation of thiosalicylic, ethanol was the best solvent of choice.
With optimal conditions in hand, to extend the scope of this method, oxidation reactions for different types of thiols were studied in both CH3CN and EtOH (Table 3).
Oxidation of thiols to sulfonic acids using Fe3O4 MNPs/H2O2 system in CH3CN or EtOH solvent under reflux conditions.
Entry | Substrate | Producta | Time | Yield | ||
CH3CN | EtOH | CH3CN | EtOH | |||
1 | 150 | 150 | 83 | 40 | ||
2 | 120 | 120 | 85 | 50 | ||
3 | 180 | 180 | 80 | 33 | ||
4 | 60 | 45 | 90 | 90 | ||
5 | 120 | 120 | 83 | 52 | ||
6 | 90 | 60 | 90 | 90 | ||
7 | 60 | 45 | 90 | 93 | ||
8 | 150 | 180 | 83 | 78 | ||
9 | 45 | 60 | 83 | 81 | ||
10 | 50 | 30 | 95 | 95 | ||
11 | 30 | 15 | 93 | 97 | ||
12 | 120 | 90 | 80 | 85 |
a All products are known and were characterized by IR and NMR spectroscopy as compared with those reported [28].
The results in Table 3 show that the thiols with more polarity were oxidized faster in ethanol (Table 3, entries 4, 6, 7, 11, and 12); however, the thiols with the lower polarity reacted faster in acetonitrile (Table 3, entries 1, 2, 3, 5, 8, and 9). Also, the reaction rate was increased with electron-donating substituents, but aromatic thiols with electron-withdrawing substituents reacted more slowly.
The chemoselectivity of this synthetic method was also studied with the oxidation 2-mercaptoethanol as a model for thiols with a hydroxyl functional group. The results showed that both of the functional groups were oxidized with the same reactivity and did not show any selectivity (Table 3, entry 10).
The catalytic activity of Fe3O4 MNPs in the presence of H2O2 for the oxidation of a series of structurally diverse sulfides was also investigated (Scheme 4).

The oxidation of sulfides to sulfoxides and sulfones.
As shown in Table 4, Fe3O4 MNPs (0.01 g) and H2O2 (30%, 10 mmol) were suitable for oxidation of sulfides to sulfoxides, and the use of excess H2O2 (20 mmol) in the presence of Fe3O4 MNPs (0.025 g) produced the corresponding sulfones in a clean reaction.
The oxidation of sulfides to sulfoxides and sulfones with H2O2/Fe3O4 system.
Entry | Substrate | Producta,b 1a | Time (min) | Yield (%) | Productb,c 2a | Time (min) | Yield (%) |
1 | 30 | 93 | 180 | 95 | |||
2 | 5 | 97 | 75 | 97 | |||
3 | 8 | 95 | 210 | 93 | |||
4 | 5 | 95 | 180 | 90 | |||
5 | 60 | 90 | 210 | 87 | |||
6 | 150 | 87 | 390 | 85 | |||
7 | 120 | 50 | 24 | 20 | |||
8 | 30 | 97 | 150 | 97 |
a Reaction conditions: sulfide (1 mmol), H2O2 (30%, 10 mmol), catalyst (0.01 g), EtOH (2.5 mL), rt.
b All products are known and were characterized by IR and NMR spectroscopy as compared with those reported [23,29–32].
c Reaction conditions: sulfide (1 mmol), H2O2 (30%, 20 mmol), catalyst (0.025 g), EtOH (2.5 mL), reflux.
A possible mechanism for oxidation of thiols and sulfides was proposed on the basis of the Fenton reaction (Scheme 5) [33].

Proposed mechanism.
3.4 Catalyst recovery
To investigate the recyclability and reusability of the catalyst for the oxidation of thiols to sulfonic acids, we examined the oxidation of 2-mercaptoacetic acid as a model compound. After the completion of the reaction, the catalyst was separated magnetically and washed with Et2O to remove the residual product. The separated catalyst was reused five times without a considerable decrease in activity as shown in Fig. 4.

Recoverability of Fe3O4 MNPs in the oxidation of 2-mercaptoacetic acid.
3.5 Selected spectral data
3.5.1 2-Sulfoacetic acid
FTIR (KBr): υ (cm−1) = 1051, 1217, 1640, 1723, 3447; 1H NMR (D2O, 250.1 MHz): δ (ppm) = 4.17 (s, 2H, CH2), 4.69 (s, 1H, DOH); 13C NMR (D2O, 62.9 MHz): δ (ppm) = 56.08, 169.23 (Table 3, entry 11).
3.5.2 Phenylmethanesulfonic acid
FTIR (KBr): υ (cm−1) = 1058, 1196, 1413, 1449, 1580, 2998, 3093, 3430; 1H NMR (D2O, 250.1 MHz): δ (ppm) = 4.44 (s, 2H, CH2), 7.66 (s, 5H); 13C NMR (D2O, 62.9 MHz): δ (ppm) = 57.25, 128.66, 129.24, 130.88, 132.09 (Table 3, entry 8).
3.5.3 2-Sulfoacetic acid
FTIR (KBr): υ (cm−1) = 1056, 1216, 1642, 1724, 3458; 1H NMR (DMSO, 250.1 MHz): δ (ppm) = 2.34 (s, 1H, SO3H), 2.48 (s, 3H, CH3), 3.42 (s, 2H, CH2), 4.22 (s, 1H, DOH); 13C NMR (DMSO, 62.9 MHz): δ (ppm) = 57.19, 168.25 (Table 3, entry 10).
3.5.4 Methylsulfinylbenzene
IR (KBr): υ (cm−1) = 696, 1029, 1128, 1296, 1451, 1488, 3030; 1H NMR (CDCl3, 250 MHz): δ (ppm) = 7.5–7.7 (m, 5H, ArH), 2.72 (s, 3H, CH3) (Table 4, entry 1) [31].
3.5.5 Methylsulfonylbenzene
IR (KBr): υ (cm−1) = 744, 1082, 1145, 1291, 1324, 1444, 1572, 2925, 3090; 1H NMR (CDCl3, 250 MHz): δ (ppm) = 7.3–7.53 (m, 5H, ArH), 2.72 (s, 3H, CH3) (Table 4, entry 1) [31].
3.5.6 Sulfinyldibenzene
IR (KBr): υ (cm−1) = 688, 734, 1021, 1077, 1436, 1471, 1576, 3060; 1H NMR (CDCl3, 250 MHz): δ (ppm) = 7.56 (d, 4H, 3JHH = 6.7, ArH), 7.29–743 (m, 6H, ArH) (Table 4, entry 7).
3.5.7 Sulfonyldibenzene
IR (KBr): υ (cm−1) = 678, 734, 1156, 1329, 1370, 1441, 1475, 1574, 3077; 1H NMR (CDCl3, 250 MHz) δ (ppm) = 7.94 (d, 4H, 3JHH = 7.7, ArH), 7.45–7.58 (m, 6H, ArH) (Table 4, entry 7) [27].
3.5.8 2-(Phenylsulfinyl)ethanol
IR (KBr): υ (cm−1) = 691, 737, 1041, 1085, 1435, 1474, 1579, 2925, 3060, 3405; 1H NMR (CDCl3, 250 MHz): δ (ppm) = 7.33 (d, 2H, 3JHH = 7.25 Hz, ArH), 7.2–7.3 (m, 3H, ArH), 3.70–3.75 (t, 2H, OCH2), 3.05–3.10 (t, 2H, CH2SO), 3.00 (m, 1H, OH) (Table 4, entry 3) [28].
3.5.9 2-(Phenylsulfonyl)ethanol
1H NMR (CDCl3, 250 MHz): δ (ppm) = 7.95 (d, 2H, 3JHH = 7.75 Hz, ArH), 7.58–7.70 (m, 3H, ArH), 3.99–4.03 (m, 2H, OCH2), 3.44–3.49 (m, 1H, OH), 3.34–3.38 (m, 2H, CH2SO2) (Table 4, entry 3).
3.5.10 2-(Methylsulfinyl)ethanol
IR (KBr): υ (cm−1) = 933, 1031, 1058, 1424, 1456, 2915, 3395; 1H NMR (CDCl3, 250 MHz): δ (ppm) = 3.53–3.58 (m, 2H, OCH2), 3.49 (m, 1H, OH), 2.48–2.53 (m, 2H, CH2SO), 1.94 (s, 3H, CH3) (Table 4, entry 4).
3.5.11 2-(Methylsulfonyl)ethanol
1H NMR (CDCl3, 250 MHz): δ (ppm) = 4.13–4.17 (m, 2H, OCH2), 3.6–3.8 (m, 1H, OH), 3.2–3.3 (m, 2H, CH2SO2), 3.03 (s, 3H, CH3) (Table 4, entry 4).
4 Conclusion
We have demonstrated that robust and magnetically reusable Fe3O4 MNPs can catalyze the oxidation of a wide variety of thiols and sulfides using hydrogen peroxide as an inexpensive and environmental oxidant. This simple reported procedure offers several significant advantages such as the use of a commercially available, eco-friendly oxidant, the mild reaction conditions, short reaction times and high yields, simple workup procedure, and recyclability of the catalyst.
Acknowledgments
We are grateful to the Research Council of Kurdistan University for the financial support of this work.