Dans ce travail, nous étudions les concepts fondamentaux du rayonnement de Hawking dans les systèmes astrophysiques et analogiques. Nous nous concentrons sur les fréquences négatives et les normes négatives : leur définition, leur relation et leur rôle dans le processus de création de particules de l’effet Hawking. Nous caractérisons la relation de dispersion par les signes de la fréquence et de la norme. Nous concluons que le cadre le plus naturel pour étudier l’effet Hawking est celui dans lequel l’horizon est statique, où le signe de la norme peut être rendu égal au signe de la fréquence décalée par effet Doppler dans ce cadre. Nous utilisons comme exemples les quatre systèmes analogues expérimentaux les plus réussis : les vagues d’eau, les condensats de Bose–Einstein, les fluides à polaritons et les fibres optiques.
In this work, we study the core concepts of Hawking radiation in the astrophysical and analogue systems. We focus on negative frequencies and negative norms: their definition, their relationship, and their role in the particle creation process of the Hawking effect. We characterize the dispersion relation by the signs of the frequency and the norm. We conclude that the most natural frame for studying the Hawking effect is that in which the horizon is static, where the sign of the norm can be made equal to the sign of the Doppler-shifted frequency in that frame. We use as examples the four most successful experimental analogue systems: water waves, Bose–Einstein condensates, polaritons fluids, and optical fibers.
Révisé le :
Accepté le :
Première publication :
Mot clés : gravité analogique, fréquence négative, norme négative, rayonnement de Hawking, impulsion optique, condensat de Bose–Einstein, polariton
Raul Aguero-Santacruz 1 ; David Bermudez 1
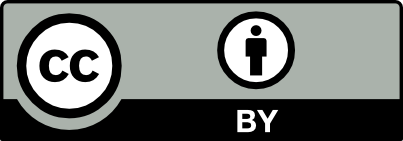
@article{CRPHYS_2024__25_S2_A2_0, author = {Raul Aguero-Santacruz and David Bermudez}, title = {Negative frequencies and negative norms in analogue {Hawking} radiation systems}, journal = {Comptes Rendus. Physique}, publisher = {Acad\'emie des sciences, Paris}, year = {2024}, doi = {10.5802/crphys.177}, language = {en}, note = {Online first}, }
Raul Aguero-Santacruz; David Bermudez. Negative frequencies and negative norms in analogue Hawking radiation systems. Comptes Rendus. Physique, Online first (2024), pp. 1-17. doi : 10.5802/crphys.177.
[1] Black hole explosions, Nature, Volume 248 (1974) no. 5443, pp. 30-31 | DOI
[2] Black holes and entropy, Phys. Rev. D, Volume 7 (1973) no. 8, pp. 2333-2346 | DOI
[3] Particle creation by black holes, Commun. Math. Phys., Volume 43 (1975) no. 3, pp. 199-220 | DOI
[4] GW190521: Search for echoes due to stimulated Hawking radiation from black holes, Phys. Rev. D, Volume 108 (2023) no. 4, 044047 | DOI
[5] Quantum fields in curved space, Cambridge Monographs on Mathematical Physics, 7, Cambridge University Press, 1982 | DOI
[6] The entropy of Hawking radiation, Rev. Mod. Phys., Volume 93 (2021) no. 3, 035002 | DOI
[7] Lifshitz theory of the cosmological constant, Ann. Phys., Volume 411 (2019), 167973 | DOI
[8] Hawking radiation in optics and beyond, Philos. Trans. R. Soc. Lond., Ser. A, Volume 378 (2020) no. 2177, 20190223 | DOI
[9] Has Hawking radiation been measured?, Found. Phys., Volume 44 (2014), pp. 532-545 | DOI
[10] Experimental black-hole evaporation?, Phys. Rev. Lett., Volume 46 (1981) no. 21, pp. 1351-1353 | DOI
[11] Observation of negative-frequency waves in a water tank: a classical analogue to the Hawking effect?, New J. Phys., Volume 10 (2008) no. 5, 053015 | DOI
[12] Horizon effects with surface waves on moving water, New J. Phys., Volume 12 (2010) no. 9, 095018 | DOI
[13] Measurement of stimulated Hawking emission in an analogue system, Phys. Rev. Lett., Volume 106 (2011) no. 2, p. 021302 | DOI
[14] Effective spacetime and Hawking radiation from a moving domain wall in a thin film of 3 He-A, JETP Lett., Volume 68 (1998), pp. 874-880 | DOI
[15] Artificial black holes, World Scientific, 2002
[16] Comment on “Questioning the recent observation of quantum Hawking radiation” [Ann. Phys. (Berlin) 2018, 530, 1700114], Ann. Phys. (Berlin), Volume 530 (2018) no. 5, 1700459 | DOI
[17] Observation of thermal Hawking radiation and its temperature in an analogue black hole, Nature, Volume 569 (2019) no. 7758, pp. 688-692 | DOI
[18] Resonant Hawking radiation as an instability, Class. Quant. Grav., Volume 36 (2019) no. 2, 024001 | DOI
[19] Fiber-optical analog of the event horizon, Science, Volume 319 (2008) no. 5868, pp. 1367-1370 | DOI
[20] Hawking spectrum for a fiber-optical analog of the event horizon, Phys. Rev. A, Volume 93 (2016) no. 5, 053820 | DOI
[21] Observation of stimulated Hawking radiation in an optical analogue, Phys. Rev. Lett., Volume 122 (2019) no. 1, 010404 | DOI
[22] Analogue quantum simulation of the Hawking effect in a polariton superfluid, Eur. Phys. J. D, Atomic Mol. Opt. Plasma Phys., Volume 76 (2022) no. 8, 152 | DOI
[23] Quantum vacuum excitation of a quasinormal mode in an analog model of black hole spacetime, Phys. Rev. Lett., Volume 130 (2023) no. 11, 111501 | DOI
[24] Analogue models of and for gravity, Gen. Relativ. Gravit., Volume 34 (2002) no. 10, pp. 1719-1734 | DOI
[25] Essential and inessential features of Hawking radiation, Int. J. Mod. Phys. D, Volume 12 (2003) no. 04, pp. 649-661 | DOI
[26] Derivation of Hawking radiation in dispersive dielectric media, Phys. Rev. D, Volume 93 (2016) no. 10, p. 104010 | DOI
[27] Departing from thermality of analogue Hawking radiation in a Bose–Einstein condensate, Phys. Rev. Lett., Volume 124 (2020) no. 6, 060401 | DOI
[28] Black hole evaporation and ultrashort distances, Phys. Rev. D, Volume 44 (1991) no. 6, pp. 1731-1739 | DOI
[29] Negative-frequency resonant radiation, Phys. Rev. Lett., Volume 108 (2012) no. 25, 253901 | DOI
[30] Negative frequencies get real, Physics, Volume 5 (2012), 68 | DOI
[31] Negative frequencies in pulse propagation equations and the double analytic signal, New J. Phys., Volume 25 (2023) no. 10, 103045 | DOI
[32] Hawking radiation without trans-Planckian frequencies, Phys. Rev. D, Volume 52 (1995) no. 8, pp. 4559-4568 | DOI
[33] Introduction to quantum effects in gravity, Cambridge University Press, 2007
[34] et al. Relativistic quantum mechanics, 2, Springer, 2000 | DOI
[35] Analogue gravity and the Hawking effect: historical perspective and literature review, Eur. Phys. J. H, Volume 48 (2023) no. 1, 15 | DOI
[36] The Trans-Planckian problem as a guiding principle, J. High Energy Phys., Volume 2011 (2011) no. 11, pp. 1-18 | DOI
[37] The theory of Hawking radiation in laboratory analogues, J. Phys. B. At. Mol. Opt. Phys., Volume 45 (2012) no. 16, 163001 | DOI
[38] Modeling of ultrashort optical pulses in nonlinear fibers (2022) (Technical report)
[39] Black-hole radiation in Bose–Einstein condensates, Phys. Rev. A, Volume 80 (2009) no. 4, pp. 12-26 | DOI
[40] Bogoliubov theory of acoustic Hawking radiation in Bose–Einstein condensates, Phys. Rev. A, Volume 80 (2009) no. 4, 043603 | DOI
[41] Observation of quantum Hawking radiation and its entanglement in an analogue black hole, Nat. Phys. (2016), pp. 959-965 | DOI
[42] The life of an analogue black hole, Nat. Phys., Volume 17 (2021) no. 3, pp. 300-301 | DOI
[43] Acoustic black hole in a stationary hydrodynamic flow of microcavity polaritons, Phys. Rev. Lett., Volume 114 (2015) no. 3, 036402 | DOI
[44] Polariton fluids for analogue gravity physics, Philos. Trans. R. Soc. Lond., Ser. A, Volume 378 (2020) no. 2177, 20190225 | DOI
[45] Rotating black hole geometries in a two-dimensional photon superfluid, Optica, Volume 5 (2018) no. 9, pp. 1099-1103 | DOI
[46] Essential Quantum Optics, Cambridge University Press, 2009
[47] Nonlinear Fiber Optics, Academic Press Inc.; Elsevier science, 2019 (digital format)
[48] Photonic crystal fibers, Science, Volume 299 (2003) no. 5605, pp. 358-362 | DOI
[49] Practitioner’s guide to laser pulse propagation models and simulation, Eur. Phys. J.: Spec. Top., Volume 199 (2011) no. 1, pp. 5-76 | DOI
[50] Exploring the quantum-to-classical vortex flow: Quantum field theory dynamics on rotating curved spacetimes (2023) (preprint, arXiv:2308.10773) | DOI
[51] Confirmation of stimulated Hawking radiation, but not of black hole lasing, Phys. Rev. D, Volume 106 (2022) no. 10, 102007 | DOI
[52] Spectroscopic measurement of the excitation spectrum on effectively curved spacetimes in a polaritonic fluid of light (2023) (preprint, arXiv:2311.01392)
[53] Spectrum of collective excitations of a quantum fluid of polaritons, Phys. Rev. B, Volume 107 (2023) no. 17, 174507 | DOI
[54] Entanglement from superradiance and rotating quantum fluids of light (2023) (preprint, arXiv:2310.16031) | DOI
[55] Effective Michelson interference observed in fiber-optical analogue of Hawking radiation, Opt. Express, Volume 30 (2022) no. 5, pp. 8063-8074 | DOI
Cité par Sources :
Commentaires - Politique