1 Introduction
In plants, many micro-organisms and most yeasts, a mitochondrial alternative oxidase (AOX) bypassing complex III and cytochrome c oxidase catalyses the oxidation of ubiquinol and the reduction of O2 to H2O [1–3]. The activity of AOX is coupled neither to proton translocation nor consequently to ATP synthesis via the activation of the ATP synthase. AOX is cyanide-resistant but is inhibited by salicylhydroxamic acid (SHAM) (Fig. 1) [4].

Molecular structure of SHAM.
Dutuit and Lhéritaud-Trousard [5] have shown that SHAM inhibited Nicotiana tabacum protoplast division when cyanide, which is an inhibitor of the phosphorylative pathway, stimulated it strongly. In other words, the overstimulation of the SHAM-sensitive pathway, obtained indirectly by the phosphorylative pathway inhibition with cyanide, leads to cell-division stimulation. Moreover, SHAM has been shown to inhibit the proliferation of the microalgae Porphyridium cruentum and Parietochloris incisa [6,7].
The working hypothesis, according to which the AOX pathway activity is necessary to cell division, has not been reformulated since, even if Cory and Cory [8] evoked the involvement of SHAM and salicylic acid derivatives in proliferation inhibition of tumour cells, focusing on the inhibitory molecules themselves, and not on the respiratory pathway. Interestingly, the presence of a mitochondrial AOX has been reported in protista, algae, fungi and in several invertebrates from three different phyla (Mollusca, Nematoda, and Chordata) [4,9]. The expression of a cyanide-resistant and SHAM-sensitive respiration does not seem to have been demonstrated in vertebrates, excepted in Lewis lung carcinoma cells [10]. In this case, no hypothesis has been formulated relative to the involvement of the alternative respiratory pathway in cell division and differentiation.
The question of SHAM specificity has been discussed for a long time in the past, especially in the case of plant organs and of plant isolated mitochondria [1]. A wide recent literature relates research on the role of lipoxygenases (LOX), cyclooxygenases (COX), and desaturases (which are inhibited by SHAM and salicylate derivatives) involved in plant and animal cell metabolism, proliferation, ovulation and differentiation [11–18]. Altogether, these observations suggest that SHAM may have several effects, including inhibition of AOX, LOX, COX, and desaturases. Compared to these studies, our experimental approach takes into account cell division and differentiation, but not enzymatic activities.
In order to gain insight into how SHAM may influence cell division, we studied its effects on the proliferation of cells from a plant Atriplex halimus and murine neuroblastoma × glioma NG108-15 cells. Its effects on cell differentiation were studied on egg laying and development of the fly Drosophila melanogaster.
2 Materials and methods
2.1 Proliferation of Atriplex halimus cells
The experiments were performed on cells isolated from a mother plant of Atriplex halimus L. harvested in the area of Djelfa (Algeria). The synthetic medium was a solution of Murashige and Skoog mineral salts [19] supplemented with 30 g l−1 sucrose, 2.5 μM 2,4-dichorophenoxyacetic acid, and 2.5 μM kinetin. SHAM was dissolved in dimethyl formamide (DMF) and added to the cell suspension at different concentrations. Cell growth was evaluated from the increase in weight of the dry material after moderate filtration and drying for 48 h at 60 °C. In all cases, the results were expressed as the mean ± SEM of 10 to 14 samples performed on the same initial cell suspension.
2.2 Experiments on murine neuroblastoma cells
Undifferentiated hybrid mouse neuroblastoma × rat glioma NG108-15 cells were cultured as previously described [20]. SHAM was dissolved in DMF and added to the culture medium at different concentrations. In each condition, including controls, the concentration of DMF was 0.1%, which by itself did not influence cell proliferation.
Cell number and mean cell volume were electronically determined with a Coulter channelizer (model Z2, Beckman-Coulter, Villepinte, France), as previously described [21]. The relative rate of cell proliferation (P) in the different experimental conditions was calculated from the cell number (N) at days 2 (D2) and 3 (D3) of culture, and expressed as: . For each individual experiment, cell volume and number were determined in triplicate. Cell viability was determined by counting the proportion of Trypan blue excluding cells.
To evaluate an eventual differentiating effect, membrane currents and potential were recorded with the whole-cell patch-clamp technique in the voltage or current clamp configuration at room temperature. The external solution contained (in mM): NaCl 140; KCl 5; CaCl2 1; MgCl2 2; Hepes 10. The pipette solution contained (in mM): KCl 140; MgCl2 2; Hepes 10. The pH of external and pipette solutions was adjusted to 7.4 with NaOH. Filled with the standard solution, the pipettes had resistances of 3–5 MΩ, and the seal resistance was at least 10 GΩ.
The cell cycle determination was studied by flow cytometry. The NG108-15 cells were plated on 10-ml Petri dishes in the medium, and aliquots of 1 ml at the beginning of experiments and 0.5 ml at the end of cell culture (47 h) were centrifuged and resuspended in 0.5 ml of a buffer (pH 7) containing 46 mM MgCl2, 30 mM sodium Tris citrate, 20 mM MOPS, and 0.1% (w/v) Triton X100 for releasing nuclei [22]. Each sample was analyzed 15 min after the addition of 5 μg/ml of propidium iodide, on a FACScan cytometer (Becton-Dickinson, Le Pont-de-Claix, France). The excitation at 488 nm was applied by an argon laser (Spectra-Physics, Mountain View, USA), and the fluorescence was measured through a 650/30-nm filter. For each sample, 10,000 nuclei were analyzed. Cell-cycle phases were analyzed by using the Modfit LT3 software (Verity Software House Inc., Topsham, USA). The percentages of cells in G1, S, and G2/M phases were determined by FACS at different times in control and in the presence of 0.3 mM SHAM. In each condition, the concentration of DMF was 0.1%.
2.3 Egg laying and development of Drosophila melanogaster
Drosophila presents well-characterized development phases. For instance, oogenesis starts at the end of the third larval stage [23], and the first eggs are laid two or three days after adult emergence.
The oocyte development starts with the asymmetric division of germinal stem cells, generating new stem cells and differentiated cells. The latter cells contribute to an egg chamber (follicle) [24]. Then, follicles pass through different stages of maturation leading to laid eggs. This process develops in ovarioles, which are regrouped in two ovaries (Fig. 2). One can divide the oogenesis into two stages: one early stage that allows the oocytes to be put in place in each egg chamber, and a late stage where the oocytes give rise to eggs ready to be laid. This event activates meiosis and the mature eggs are fertilized during the laying [25].

Schematic representation of a Drosophila female gonad.
In order to study the effect of SHAM on the female fertility, virgin females were isolated since their emergence. At time zero, samples of 10 females (2–4 h old), were placed at 25 °C in tubes containing various concentrations of SHAM, in the presence of males. SHAM was dissolved in 0.02% DMF, which at this concentration had no effect on oogenesis and egg laying. The number of laid eggs was counted as a function of time, and a Chi2 test was made. After each counting, females were put on a fresh medium, giving the following egg series. Eggs were stored at 25 °C and observed until adult emergence. The eventual effect of SHAM on toxicity and viability, from oogenesis to adult emergence and on morphological defects of embryonic, larval, pupal, and adult stages could thus be verified. The number of new white pupae was checked as a function of time since egg laying. The egg-laying precocity was studied from samples of 30 virgin females (2 h old) put with males in tubes containing 0 or 0.6 mM SHAM.
To better characterize the effects of SHAM on oogenesis, ovaries, which were put on media containing 0 or 0.6 mM SHAM, were dissected after 6, 24, and 48 h, fixed in 4% formaldehyde for DAPI staining, and observed with epifluorescence. The number of ovarioles per ovary was counted. The progression of oogenesis as a function of time was estimated by counting the number of ovarioles producing a follicle in the late stage.
3 Results
3.1 Effects of SHAM on Atriplex halimus cell growth
Fig. 3A shows the effects of various concentrations of SHAM on the evolution with time of the weight of dry material. SHAM dose-dependently delayed cell growth, apparently without cytotoxicity, since, even at the highest concentration used (0.125 mM), there was no decrease in the weight of dry material. It should be noted that at this concentration, SHAM inhibited cell growth for at least 10 days, whereas 0.05 mM SHAM had no effect or even stimulated cell growth and 0.1 mM SHAM delayed cell growth for 7 days, but had no effect on the maximal weight of dry material. After 7 days of cell culture, the IC50 (50% inhibition concentration) on the weight of dry material was about 0.09 mM (Fig. 3B).
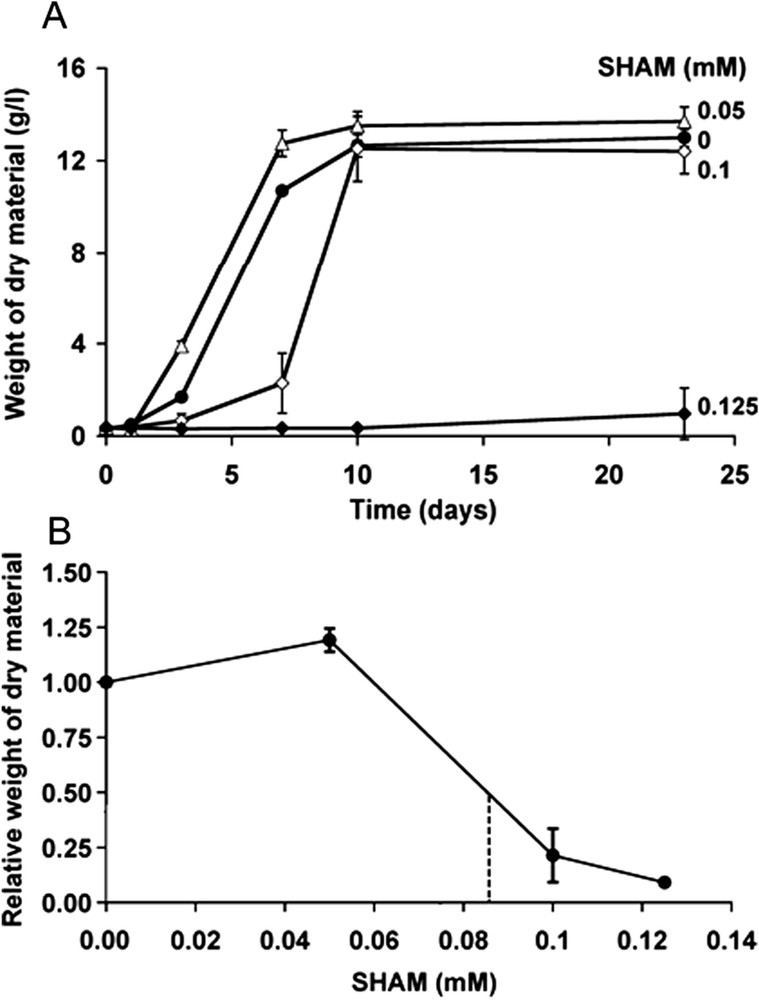
Effects of SHAM on the proliferation of Atriplex halimus cells. A: The cells were cultured in the presence of various concentrations of SHAM and their proliferation was evaluated from the weight of dry material between day 0 and day 23. Vertical bars represent the SEM. B: Relative weight of dry material after seven days of cell culture as a function of SHAM concentration. The dashed line corresponds to 50% reduction of weight of dry material.
3.2 Effects of SHAM on the proliferation and size of neuroblastoma cells
Fig. 4A shows the evolution with time of the cell number in the presence of various concentrations of SHAM. It should be noted that, whereas 0.15 mM SHAM slightly decreased cell proliferation, 0.3 mM SHAM almost completely inhibited cell proliferation and 0.6 mM SHAM decreased the cell number and thus had a cytotoxic effect. Fig. 4B shows that 0.3 mM SHAM increased the cell volume. Fig. 5 shows the dose-response curves of the effects of SHAM on the relative rate of proliferation (Fig. 5A), and the relative cell volume (Fig. 5B). The IC50 on these two parameters were about 0.25 mM.

Effects of SHAM on the proliferation and size of neuroblastoma cells. A: Evolution with time of the cell number in the presence of various concentrations of SHAM. Mean ± SEM of 12 experiments in control and three experiments in the presence of SHAM. In each case, the SEM bars were smaller than the points. B: Evolution with time of the mean cell volume under control conditions (circles) and in the presence of 0.3 mM SHAM (squares). Mean ± SEM of 12 experiments in control and three experiments in the presence of SHAM. In most cases, the SEM bars were smaller than the points. The insert shows the cell volume distribution at day 2 of culture of one representative experiment under control conditions and in the presence of 0.3 mM SHAM.

Dose-response curves of the effects of SHAM on neuroblastoma cell proliferation and volume. A: Relative rate of cell proliferation between day 2 and day 3 of culture. B: Relative cell volume at day 2 of culture. In A and B, the points are the mean of three experiments, and dashed lines correspond to 50% inhibition of cell proliferation and increased cell volume, respectively.
3.3 Cell cycle and viability of neuroblastoma cells
Fig. 6 shows the cell cycle progression under control conditions and in the presence of 0.3 mM SHAM. After 2 h of application, SHAM induced an acceleration of the transition from the G1/S to the G2 phase. In contrast, at 23 and 47 h, SHAM induced an accumulation of the cells in S phase. In control, it should be noted there was a progressive accumulation of cells in G1 phase. This last phenomenon is likely due to increased cell density and cell metabolism-induced extracellular acidification [21]. The cell viability, determined from the percentage of trypan blue excluding cells, was 97.1 ± 0.3 () in control and 94.1 ± 1.3 () after 24 h in the presence of 0.3 mM SHAM (Student's t-test: ).
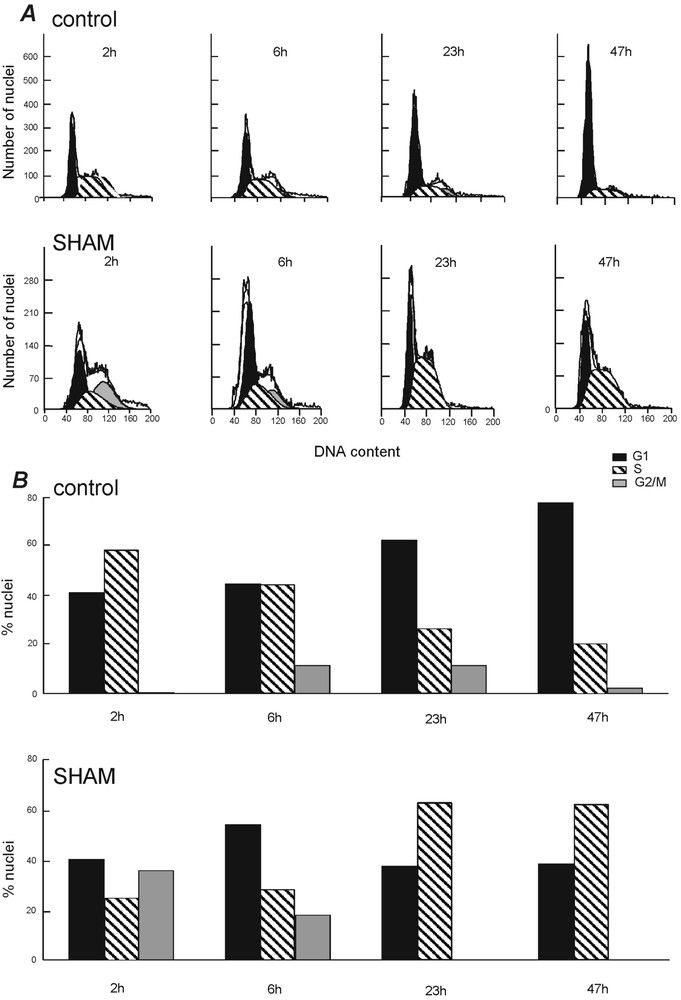
Effects of SHAM on neuroblastoma cell-cycle progression. The percentage of cells in G1, S, and G2/M was determined by FACS at different times after addition to the cultures of DMF (control) or 0.3 mM SHAM.
3.4 Electrophysiological properties of neuroblastoma cells
Compared with undifferentiated NG108-15 cells, chemically differentiated neuroblastoma cells have several neurites, are about two times larger, are excitable, and have a several-nA maximal peak inward Na+ current and transient outward K+ current [26–28]. Given that SHAM inhibits lipoxygenases that may influence cell differentiation, we examined whether it induced differentiation of NG108-15 cells. When cultured for one or two days in the presence of 0.3 mM SHAM, the cells were morphologically similar to control cells (except their increased size). They were unexcitable, and the amplitude of their maximal peak inward Na+ and outward K+ currents was not significantly different from that in control cells. However, the cells were depolarized by about 10 mV and their input membrane resistance was decreased by 27% (Table 1).
Effects of SHAM on the electrophysiological properties of neuroblastoma cells. The cells were cultured for 1 or 2 days under control conditions (0 mM SHAM) or in the presence of 0.3 mM SHAM. Then, in the standard external solution, we measured the resting potential (), the input membrane resistance () and the amplitude of the peak Na+ and K+ currents during depolarisations to −20 and +50 mV, respectively, from a holding potential of −80 mV. was calculated in current clamp conditions from the hyperpolarisation induced by the injection into the cells of a 10-pA current. Mean ± SEM of 5 to 11 cells in each condition
SHAM (mM) | (mV) | (GΩ) | (nA) | (nA) |
0 | −60 ± 3 | 2.36 ± 0.38 | 0.80 ± 0.16 | 1.50 ± 018 |
0.3 | −51 ± 3 | 1.73 ± 0.18 | 0.60 ± 0.14 | 1.19 ± 0.29 |
3.5 Fecundity and development of Drosophila melanogaster
Fig. 7 shows the cumulative number of eggs laid by 10 females as a function of time. Starting with the first series of laying (0–24 h), 0.6 and 1.25 mM SHAM increased the number of laid eggs by about 50%. A Chi2 test made on the number of non-cumulative laid eggs was significant (). In the presence of 0.6 and 1.25 mM SHAM, the increase of laid eggs was maintained during 11 days. In contrast, 2.5 mM SHAM had no significant effect.
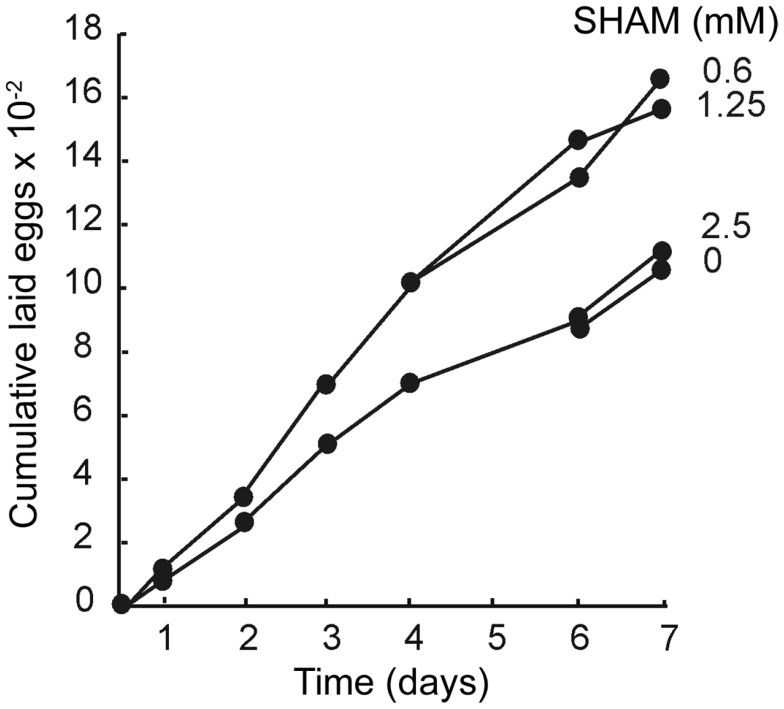
Effects of SHAM on the cumulative number of laid eggs by Drosophila. Cumulative eggs laid by 10 females were counted as a function of time in the presence of various concentrations of SHAM.
The development of laid eggs was observed until adult emergence in the presence of SHAM. SHAM had effects neither on the different phases of development (embryonic, larval, and pupal) nor on the morphology of the adult cuticle. Moreover, the flies could be maintained in the presence of SHAM for longer times than the seven days corresponding to Fig. 7, without any consequence on viability.
We examined the lapse of time between egg laying and the emergence of the first pupae in some series. Between 72 and 116 h after egg laying (AEL), white pupae were 18% with 0.6 mM SHAM and 9% without SHAM (Fig. 8a). Between 92 and 116 h AEL, white pupae are 43% with 0.6 mM SHAM and only 20% without SHAM (Fig. 8b). All fertilized eggs gave pupae after 140 h without mortality in control and in the presence of 0.6 and 1.25 mM SHAM (Fig. 8c). These differences were significant in a Chi2 test (). All pupae gave adults in control and in the presence of SHAM.
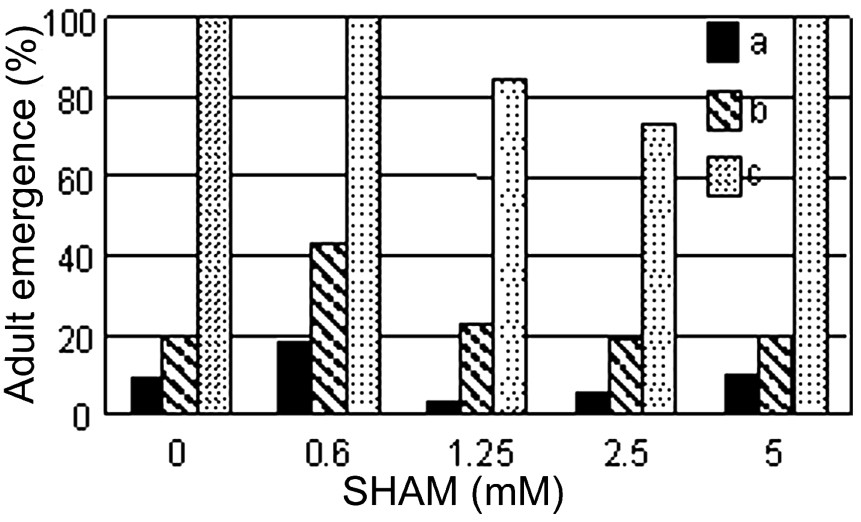
Effects of SHAM on Drosophila pupae emergence. Percentage of pupae relatively to the initial fecundated eggs. Pupae were counted (a) 72 to 116 h after egg laying (AEL), (b) 92 to 116 h AEL, (c) 116 to 140 h AEL.
In the presence of 0.6 mM SHAM, the first eggs appeared as early as 16 h, with nine eggs. In contrast, in the absence of SHAM, no eggs appeared until 22 h. At time 29 h, the control females produced 23 eggs, whereas the SHAM-treated females produced 49 eggs. These results indicated clearly that SHAM induced an egg laying precocity.
In order to study the oogenesis and development, ovaries of females cultured 6, 24, and 48 h in the presence of 0.6 mM SHAM or in control medium were observed after DAPI staining. It appeared (Table 2) that: (1) whatever the time, there were less ovarioles per ovary in the presence of SHAM than in control (Student's t-test: ), but the production of egg chambers was not stopped; (2) until 24 h, there were more ovarioles producing a late follicle in the presence of SHAM than in control (Chi2 test: ). In contrast, at 48 h, there were less ovarioles with late follicle in the presence of SHAM than in control (Chi2 test: ); (3) at 48 h, there were more ovarioles with an abortive egg-chamber with SHAM than in control. Few or no abortive egg chambers were observed in control and in SHAM-treated flies before 48 h. Thus, oogenesis in the presence of SHAM was faster than in control, which was coherent with the observation of the earlier egg laying. The persistence of the increased-egg number until 11 days was more surprising. Moreover, the fact that there were less ovarioles per ovary in the presence of SHAM than in control could mean that the increased rate of oogenesis induced by SHAM was more important than the results indicate. Altogether, our results give evidence for precocity of the different stages of development in the presence of SHAM.
Effects of SHAM on the kinetics of Drosophila oogenesis. Numbers of whole ovaries, ovarioles (1: in complete ovaries; 2: all the observed ovarioles), ovarioles with one follicle in late stage (L), ovarioles with one abortive stage (A) and their respective frequencies, as a function of time in control and in the presence of 0.6 mM SHAM
Time (h) | 6 | 24 | 48 | |||
Medium | Control | SHAM | Control | SHAM | Control | SHAM |
Ovaries | 16 | 20 | 5 | 15 | 18 | 14 |
Ovarioles (1) | 192 | 213 | 103 | 251 | 331 | 207 |
Ovarioles (1) per ovary | 12 | 10.65 | 20.60 | 16.73 | 18.39 | 14.79 |
Ovarioles (2) | 192 | 213 | 152 | 290 | 405 | 288 |
Ovarioles (2L) | 16 | 27 | 30 | 91 | 166 | 100 |
Ovarioles (2L) per ovariole (2) | 0.08 | 0.13 | 0.20 | 0.31 | 0.41 | 0.35 |
Ovarioles (2A) | 0 | 2 | 2 | 10 | 0 | 25 |
Ovarioles (2A) per ovariole (2) | 0 | 0.01 | 0.01 | 0.03 | 0 | 0.09 |
4 Discussion
4.1 Cytotoxicity of SHAM
0.125 and 0.6 mM SHAM did not induce particular toxicity on Atriplex cells and Drosophila, respectively. On neuroblastoma cells, 0.3 mM SHAM did not induce toxicity, but 0.6 mM SHAM induced a slight toxicity. In the case of Drosophila, neither abnormal morphogenesis nor defects in the adult cuticle were associated with the growth in the presence of SHAM. The viability of the different stages of development was identical in control and in the presence of SHAM. The fact that there were more abortive egg chambers in the presence of SHAM than in control was not necessarily an indication of cytotoxicity, but could be due to an accelerated differentiation of the different oogenesis phases and to defects associated with an increased speed of these processes. Moreover, 2.5 mM SHAM induced a production of eggs near to that of control. This may reflect the existence of an optimum in the concentration-dependent effects of SHAM. Nevertheless, no perturbation in the mechanism of egg production or the viability of adults at high SHAM concentrations was observed.
4.2 Cytostatic effects of SHAM
0.3 mM SHAM had a cytostatic effect on neuroblastoma cells, since, after 23 h of action, the cells accumulated in S phase (Fig. 6). Correlatively, the cell size increased (Fig. 4B). This is in agreement with the observation that the rate of neuroblastoma and glioma cell proliferation decreases when the cell size increases beyond an optimal value [21,29].
The effects of salicylates on the cell cycle have been largely documented, but their role is not clear. Salicylates exert direct modulation of the growth regulatory pathways by inhibition of the protein kinase effectors JNK, AP-1, and p70s6k [30]. Several studies have shown that salicylates induced a terminal arrest of the cell cycle in G1/S phases. In our experiments on neuroblastoma cells, after 6 h of application, 0.3 mM SHAM boosted the passing from G1 to S phase and inhibited the passing to G2/M phases. A possible hypothesis is that SHAM activated cyclin E via a p21 increase and inhibited cyclin B1, as obtained with other salicylates [31]. Law et al. [32] found that salicylates at concentrations higher than 5 mM inhibited cyclin A and cyclin D1, via the inhibition of p70s6k. Marra and Liao [30] showed that the inhibition of vascular smooth cell proliferation was due to an upregulation of p21 and p27, cyclin A was inhibited, but cyclins D and E were not affected. Ricchi et al. [33] demonstrated that the protection of Caco-2 cells from apoptosis by aspirin resulted from the activation of the phosphatidylinositol 3-kinase/AKT/p21 pathway. Our results are in agreement with these conclusions, but the pleiotropic effects of salicylates make an interpretation highly hazardous.
A great difference between SHAM and other salicylates is the range of concentrations necessary to obtain a visible biological effect. SHAM acts between 0.1 to 0.3 mM, whereas aspirin and salicylic acid present activity between 2 to 10 mM. Moreover, 0.3 mM SHAM did not induce apoptosis in neuroblastoma cells. What is more, in mouse thymocytes and in K-562 adenocarcinoma cells, no sign of apoptosis was observed, even if the SHAM concentration was higher than 2.5 mM (personal unpublished results).
4.3 Differentiating effects of SHAM
SHAM increased the rate of oogenesis and of the different stages of fly development. These effects were significant and not transient, since in the presence of 0.6 and 1.25 mM SHAM, the number of laid eggs remained 50% larger than in control during 11 days.
Two hypotheses can be proposed to explain the long-lasting increase of laid eggs in the presence of SHAM. The first one is an increase of ovarioles per ovary. This hypothesis is not valid, since the results show the contrary (Table 2). The second hypothesis is an acceleration of oocyte maturation. According to this hypothesis, this accelerating process could concern all the different phases of oogenesis. The first stage of oogenesis is the differentiation of oocytes in the germarium. At the initial stage of gametogenesis, the division of one germinal mother stem cell gives a new stem cell and a cell that enters in differentiated phase. It remains to define the target of SHAM in this process of cell division and differentiation of one of the daughter cells. It is unlikely that the affected process is upstream from the division of the mother cell, since, in this case, the two daughter cells would differentiate and engage in the production of follicles. Consequently, the production of follicles should be stopped, and this is not observed in our experiments.
The effects were fast, since as early as 6 h, the percentage of ovarioles producing a late follicle was larger in the presence of SHAM than in control. Similarly, a cytostatic effect of SHAM was observed in cytometry studies on neuroblastoma cells as early as 6 h. Moreover, all phases that follow the fertilization of Drosophila eggs were faster with than in the absence of SHAM.
In conclusion, the present results show that SHAM has effects on proliferation and differentiation in our three experimental models. For the three preparations we studied, the fact that SHAM had dose-dependent effects in a very narrow concentration range suggests that it inhibits different enzymes, including oxygenases and desaturases, and thus has additive effects. Given that SHAM preferentially inhibits the division of highly proliferative cells, it could be a potent inhibitor of cancer cells that overexpresses lipoxygenases [34]. Further experiments on tumour and normal cells would confirm or dismiss this hypothesis. Moreover, given the effects of SHAM on Drosophila egg laying, it could be used to stimulate the production of oocytes and eggs in different animals.
Acknowledgements
We thank Prof. Claude Lance and Dr Seana O' Regan for critical reading on the manuscript, Dr. Catherine Agier and Michel Bury for scientific collaboration in Atriplex halimus experiments and Monique Auberbiac for her technical assistance in Drosophila experiments.