1 Introduction
A great interest is actually devoted to the preparation of nanocomposites. These multifunctional materials are usually built from a topotactic reaction between a 2D host structure and a guest molecule [1,2]. Owing to the highly tuneable properties of their two constituents (for instance, thermoplasticity, insulative or conductive properties for the polymer, UV protection, delay in flammability from the inorganic host structure point of view), the nanocomposites find their applications in a large number of fields, such as those emphasising mechanical enhancement, gas permeability or polymer electrolyte [3–7].
In this study, we focussed our attention on the incorporation of a polymer into the lamellar structure of layered, double hydroxides. The latter are made of sheets of edge-sharing metal octahedra where, by comparison to brucite M(OH)2, a fraction of the divalent metal cations (M) is replaced by trivalent cations (M′). The excess of charge, i.e. M′, is counterbalanced by interlayered anions, leading to an anionic exchange capacity. Two LDH materials M2M′ (OH)6Cl·2 H2O, where (M, M′) = (Zn, Al) and (Cu, Cr) are designed as the inorganic host framework.
Direct polymer incorporation is hampered by the adsorption on crystallites, thus limiting the diffusion between the layers. Therefore, the polymerisation of the interleaved monomer is an appropriate method, as illustrated by several systems such as pyrrole/V2O5 xerogel [8], aniline/RuCl3 [9], etc., although in situ polymerisation requires usually low pH or/and the use of an external oxidising agent, such as (NH4)2S2O8 or FeCl3. LDH material is plagued by these two conditions, as it dissolves at low pH, and it presents a great affinity for the counter-anions of the oxidising agent, thus back-exchanging the monomer [10]. An alternative method consists of synthesising the LDH material in the presence of the polymer, the so-called ‘templating method’. However, this method gives rise to ill-defined solids [11].
We present an easy pathway to reach in situ polymerisation. First, the monomers, vinyl or amino-benzene sulfonate (called VBS and ABS), are incorporated by an anion exchange reaction from the chlorine anions present between the LDH sheets. A soft thermal treatment is then applied to the monomer/LDH derivatives. Recently, this process was found to induce the polymerisation of aniline carboxylic acid in the galleries of a LiAl2(OH)6Cl·H2O hydrotalcite-type sample [12], the pristine material being, however, partially exchanged with the monomer. In the present contribution, the in situ polymerisation of VBS and ABS between the layers of LDH materials are characterised by 13C CP-MAS NMR and ESR spectroscopies, respectively.
2 Experimental section
2.1 Preparation
The starting LDH substrates M2M′ (OH)6Cl·n H2O were prepared using a coprecipitation method for (M, M′) = (Zn, Al) [13] and the ‘salt and oxide dissolution’ synthesis for (M, M′) = (Cu, Cr) [14]. For the first method, a solution of ZnCl2 (2 M) and AlCl3 (1 M) was slowly added to decarbonated water under a vigorous stirring at a constant pH of 9. For the second, 250 cm3 of CrCl3 (10–2 M) were added dropwise to a suspension of copper oxide (CuO) (20 mmol; grain size ∼ 5 mm; Aldrich). In each case, the slurry was aged overnight, centrifuged and then washed several times with decarbonated water and finally dried at room temperature.
For the exchange reactions from the chloride anions, the quantity of monomer corresponded to twice the exchange capacity. VBS (CH= CH2–C6H4SO3H, Aldrich 99% purity grade) and ABS (NH2–C6H4SO3H, 97%, Aldrich) were used as received. The exchange capacity is of 270 and 290 mequiv/100 g for (M, M′) = (Cu, Cr) and (Zn, Al), respectively. The exchange reaction time was 24 h. The resulting powder was separated as described above.
Elemental analysis was performed at the Vernaison Analysis Centre of CNRS by using the inductive conduction plasma coupled to atomic emission spectroscopy (ICP/AES) technique.
2.2 Instrumentation
X’Pert Pro Philips equipped with a HTK16 Anton Paar chamber and a PSD-50m Braun detector was used to record XRD diagrams. The conditions were: step width of 0.0387 °, time per step of 60 s, and an aperture of 2 ° (155 channels).
ESR spectra were recorded using an X Band Bruker EMX spectrometer equipped with a standard variable temperature accessory and operating at 9.653 GHz at room temperature and at 9.411 GHz when the temperature accessory is set up. Diphenylpicrylhydrazyl (DPPH) is used to determine the resonance frequency (g = 2.0036 ± 0.0002).
13C solid-state NMR experiments were performed on a 360 Brüker spectrometer at room temperature with a 4-mm diameter zirconia rotor. To enhance the sensitivity of the nucleus, cross-polarisation (CP) technique was performed. Under a spin-locking of the protons, the conditions of Hartmann–Hahn are applied. 13C CP-MAS NMR spectra were obtained at 75.46 MHz, 1H 90 °, with a pulse width of 4.7 μs, a CP time of 1.5 ms, and a recycling time of 3 s. The chemical shift was calibrated with the carbonyl of glycine at 176.03 ppm. The spinning condition was 10 kHz.
Electrochemical measurements were performed in a three-electrode cell, with acetonitrile (ACN) and LiClO4 (0.5 M) as electrolyte. The counter- and reference electrodes were in platinum. The experiments were carried in potentiostatic mode, with steps of 50 mV s–1, and measured with a Princeton EGG–273 apparatus. The composite working electrode deposited on Pt grid is based on 80, 10, and 10% in weight of active material, carbon black, and polyvinylidene difluoride, respectively.
3 Results and discussion
The chemical analyses are reported in Table 1. For the chlorine LDH pristine material, noted as Zn2Al/Cl and Cu2Cr/Cl, the ratio between the two cations matches the nominal 2:1 value, showing that the reaction of formation is completed. The exchange reactions with the organic molecules, VBS and ABS, are also completed, since the chloride anions are present as traces only. The monomer-modified LDH materials are noted Zn2Al/VBS and Cu2Cr/ABS.
Chemical compositions of LDH and derivative materials reported per (OH)2.
Sample | Composition |
Zn2Al/Cl | Zn0.67Al0.33(OH)2Cl0.33·0.75 H2O |
Cu2Cr/Cl | Cu0.68Cr0.32(OH)2Cl0.33·0.92 H2O |
Zn2Al/VBS | Zn0.67Al0.33(OH)2(CH2CHC6H4SO3)0.33Clε·0.78 H2O |
Cu2Cr/ABS | Cu0.67Cr0.33(OH)2(C6H4NH2SO3)0.32Clε·0.85 H2O |
The powder X-ray diffraction patterns of MM′(OH)6Cl·n H2O, (M, M′) = (Zn, Al) or (Cu, Cr) are characteristic of the LDH structure [14]. The pattern was refined using R3m space group in rhombohedral symmetry (eight distinct dhkl distances were indexed). The cell parameters a and c (equal to three times the interlamellar distance, c = 3 d(003)) are 3.07 and 23.28 Å, respectively, for [Zn2Al–Cl], and 3.11 and 22.53 Å for [Cu2Cr–Cl]. The exchange of the chlorine anions by the monomer molecules increases the basal spacing, d(003), of the host structure, from 7.76 to 18.04 Å for the incorporation of VBS between [Zn2Al] sheets, and from 7.51 to 15.35 Å for ABS between [Cu2Cr] (Fig. 1). The values are consistent with two layers of monomer on each side of the interlamellar gallery.

In situ XRD patterns of (1) LDH pristine and monomer derivatives at (2) 25 °C and (3) 200 °C for the materials (a) Zn2Al/VBS and (b) Cu2Cr/ABS. The asterisk corresponds to PSD system. The Miller indexing is given.
A soft thermal treatment was applied for 4 h, at 160 and 180 °C, to the monomer-modified LDH materials; they are then called Zn2Al/VBS-T and Cu2Cr/ABS-T. For the former, the colour turns from white to yellow and, for the latter, pale green to dark brown. The harmonics (00l) for the two materials become broader, and a decrease in the interlayer distance, d(003), is observed, down to 14.46 and to 12.71Å for the nanocomposites Zn2Al/VBS-T and Cu2Cr/ABS-T, respectively (Fig. 1). A similar contraction of the lamellar structure was observed for the in situ polymerisation of α,β-aspartate into hydrotalcite-type compounds [15].
To elucidate whether or not the polymerisation does occur after the thermal treatment, 13C CP–MAS NMR and ESR spectroscopies are employed to scrutinise the nanocomposites Zn2Al/VBS-T and Cu2Cr/ABS-T, respectively.
The 13C CP-MAS spectrum of VBS/Zn2Al before and after thermal treatment is displayed in Fig. 2. The resonance peaks for the VBS molecule C1H2= C2H-C3(C44H4)C5–SO3 are assigned as follows: C1, 113.9 ppm; C2, 137.4 ppm; C3, 141.5 ppm; C4, 125 ppm; C5, 143.8 ppm. When incorporated between [Zn2Al] LDH sheets (no heat treatment), some resonance peaks are shifted; this corresponds to a shielding effect for C2, C3, and C5. This is explained by the electrostatic interaction between SO3 function and the LDH surface, thus weakening the electrophilic character toward C5. The effect propagates through the benzene backbone down to C1, which is downfield shifted. After the treatment, the resonance peaks of the material resemble that of poly(styrene) sulfonate, noted as PSS, with the disappearance of C1 and the formation of humps located at ~40–50 ppm, characteristic of –CH and –CH2 responses. In the meantime, C3 is shifted, as expected in the absence of the vinyl group. Undoubtedly, it means that VBS monomers have been polymerised between Zn2Al LDH layers.
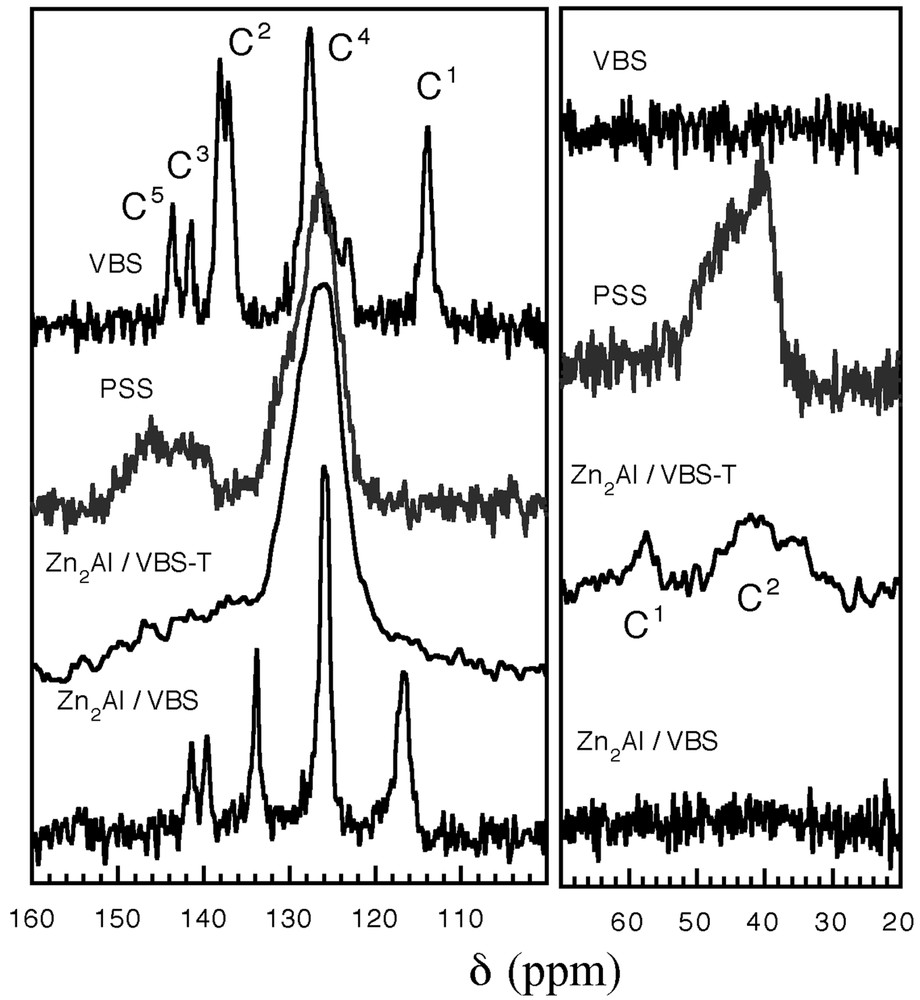
13C CP- MAS spectra. Assignment of the resonance peaks is indicated in the text.
ESR measurements are conducted for the nanocomposite ABS/Cu2Cr hybrid (Fig. 3). In addition to the LDH response (associated with a peak-to-peak linewidth, noted as ΔHpp, close to 1100 ± 20 G (g = 2.0621 ± 0.0005), a narrow signal appears at 393 K (ΔHpp = 30 ± 4 G), associated with a g value of 2.0034 ± 0.0004. This value is in the range commonly observed for organic radicals and/or conduction electrons and comparable to what is found for other relative systems, such as PANI intercalated in layered perovskite [16]. When the thermal treatment is performed in the absence of air, the narrow signal is absent. Additionally, the molecules ABS do not provide such a signal in temperature, pointing out the importance of the confinement supplied by LDH framework.
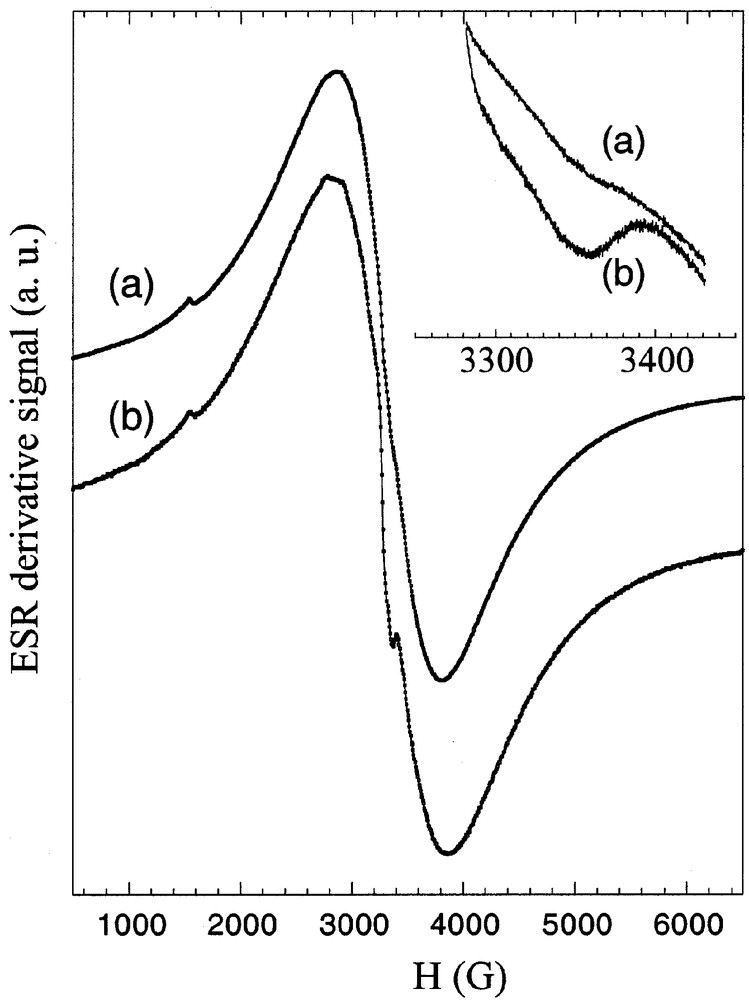
In situ derivative ESR signal of the material Cu2Cr/ABS at (a) 273 K and (b) 413 K recorded with a sweep width of 6500 G. In inset, the spectra are recorded with a sweep width of 150 G to magnify the narrow signal (receiver gain: 5000).
The presence of a quinoid structure in the nanocomposite ABS/Cu2Cr-T was confirmed by a FTIR study, with the presence of a vibration band at 1597 cm–1 [17].
To further ascertain results, the electrochemical behaviour of the material Cu2Cr/ABS-T is studied in an aprotic solvent (Fig. 4). The anodic sweep is characterised by a peak at Va = –800 mV/Pt. The current peak is ascribed to the quasi-reversible oxidation of leucoemeraldine to emeraldine [18]. In contrast, the pristine material Cu2Cr/Cl exhibits a featureless capacitive response (Fig. 4).
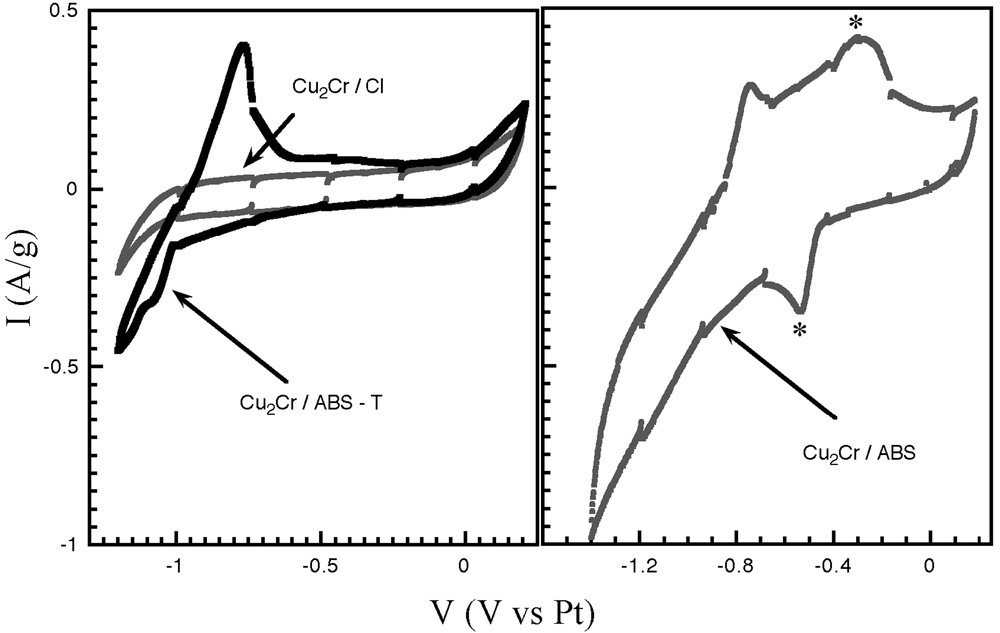
Cyclic voltammograms for the materials Cu2Cr/Cl, Cu2Cr/ABS, and Cu2Cr/ABS-T. The asterisks (*) indicate the response of the dimers (see text).
The electropolymerisation of the material Cu2Cr/ABS was also studied. On the anodic sweep, two current peaks are observed, the peak at –750 mV is ascribed to the p-doping of the polymer [18], the reversible response at –280 mV (and Vc = –550 mV, marked by an asterisk) is associated to the stabilisation of dimers [19].
As reported by other authors, the polymerisation of an amino ring-substituted monomer may then be achieved in hydrophobic solvent [20, 21], although, in our case, it is accompanied by the formation of dimers. Moreover, the absence of the electrochemical response of dimer confirms the efficiency of the thermal treatment for the polymerisation process.
4 Conclusion
We demonstrate that in situ polymerisation may be achieved within the interlayered space of non-oxidising LDH host structure by a soft heat treatment. In the present contribution, it is exemplified by the nanocomposites Zn2Al/VBS and Cu2Cr/ABS. The organic–inorganic materials are characterised by a combination of techniques.