1 Introduction
The chemistry of fullerenes has developed fast in recent years. Early investigations emphasized the understanding of the new type of reactivity presented by their curved surfaces [1]. Then, exohedral organic functionalization of fullerenes followed [2]. Nowadays, supramolecular chemistry and self-assembly of fullerene-based materials are receiving increasing attention [3]. It is already known that fullerenes possess a strong tendency to form clusters of different sizes, especially in polar solvents [4–11]. The main advantage gained upon functionalization of fullerenes is the solubility enhancement of the so-produced organofullerene derivatives. The difficult processibility of fullerenes due to their poor solubility in most organic solvents is surmounted with the aid of functionalization and the construction of novel organofullerene materials [12]. Therefore, organic functionalization of fullerenes put them in pole position in the run for applying envisioned innovative uses.
Fullerenes are very good electron acceptor materials. Particularly, the most intriguing characteristics of C60 in electron-transfer processes have been found to be its low reorganization energy and the acceleration of photo-induced charge separation. Novel applications that have been envisioned include energy storage devices, conversion of light into electric current and mimicking natural photosynthesis [13–18]. The electron-transfer process can be controlled by covalently linking donor and acceptor moieties incorporating fullerene units. In addition, it has already been shown that the rates of forward and back electron transfer in such hybrid systems are strongly dependent on the molecular topology [19, 20]. In other words, the extent and the rate of charge recombination could be decreased by modulating the separation (or the spatial orientation) of the donor and acceptor units by means of flexible or rigid hydrocarbon chains or even steroid bridges, while maintaining a high rate of charge separation.
Several organofullerene donor–acceptor molecular material hybrid systems have been synthesized, especially via 1,3-dipolar cycloaddition reactions of azomethine ylides. The most active research topic on this particularly technological field relies on: (i) the arrangement of several redox-active building blocks, in which multi-step electron transfer can occur, (ii) the design of supramolecular systems of non-covalently attached chromophores and (iii) the enhancement of the electron-acceptor properties of the hybrid material [21, 22].
2 Fullerene-based nanostructures
Recently, different techniques for synthesizing and characterizing nanostructured assemblies have been developed at the interface between chemistry and materials science. In order to make the final assembly stable enough and to have a well-defined shape, the non-covalent interactions between molecules must be stable and strong. Therefore, molecules in self-assembled aggregates should be joined by many non-covalent interactions, in order to achieve acceptable stability. Moreover, these interactions must be more favourable energetically than competing interactions with solvent and must be able to overwhelm the entropic advantages of disintegration of the ordered aggregate into disordered or dissociated states.
In Fig. 1, the chemical structures of amphiphilic fulleropyrrolidines that have been synthesized [23, 24] and studied as regards their self-assembly [24] are presented. Fulleropyrrolidines 1, 2 and 3 were obtained by 1,3-dipolar cycloaddition of azomethine ylides to C60 [25, 26] and characterized by standard analytical methods.
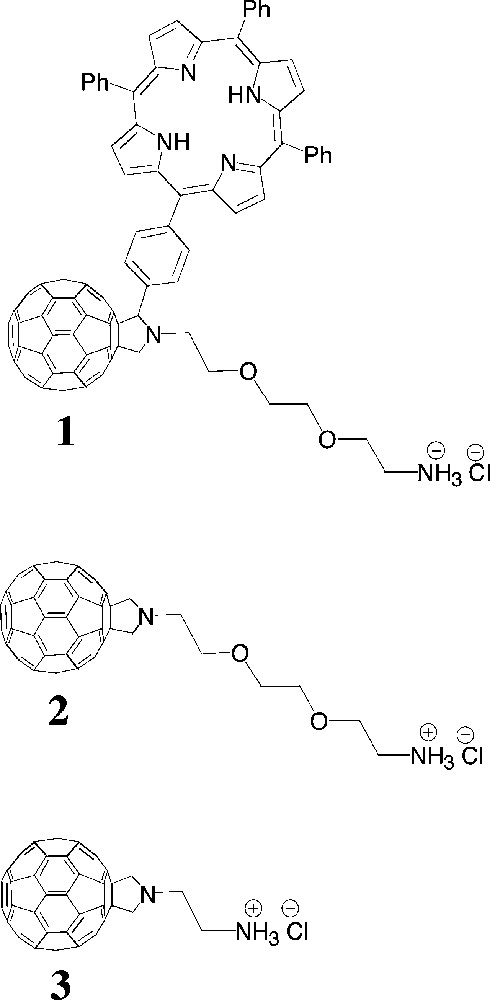
Chemical structures of amphiphilic fulleropyrrolidines
Azomethine ylides are organic 1,3-dipoles possessing a carbanion next to an immonium ion [27]. Cycloadditions to dipolarophiles provide access to pyrrolidine derivatives, useful intermediates in organic synthesis with stereo- and regiochemical control. Azomethine ylides can be readily produced upon decarboxylation of immonium salts derived from the condensation of α-aminoacids with aldehydes or ketones. When they are added to C60, a fulleropyrrolidine monoadduct is formed, in which a pyrrolidine ring is fused to the junction between two six-membered rings of a fullerene (Fig. 2) [25, 26, 28]. Very importantly, structurally different derivatives can be obtained by either using properly functionalized azomethine ylides or by modifying a fulleropyrrolidine intermediate itself.

General reaction scheme of 1,3-dipolar cycloaddition of azomethine ylides to C60.
It is the combination of the hydrophobic fullerene core with the hydrophilic oligoethylene glycol ammonium salt groups and/or the porphyrinic skeleton that forces these compounds to assembly in either spheres, bundles of ropes or tubules, respectively, when dissolved in aqueous media. Briefly describing the experimental procedure, various types of aggregates were organized by ultrasonication of water dispersions of the examined fulleropyrrolidine materials 1, 2 and 3. After centrifugation of the heterogeneous mixture, the supernatant solution was transferred onto a grid for Transmission Electron Microscopy (TEM) study.
In this manner, fulleropyrrolidine 1 was visualized to self-assemble in tubular superstructures reaching 30 nm in diameter and 500 nm in length, while 2 was found to assemble in nanorods of 4 nm in diameter and several microns in length, respectively. Furthermore, changing the oligoethylene glycol/ammonium salt chain with a short aliphatic one, as in fulleropyrrolidine 3, resulted in a different morphologically organized arrangement: superstructures of nanospheres with diameters ranging from 500 nm to 1.2 μm, that were found to aggregate together. Representative images for all the above-mentioned superstructures are shown in Fig. 3.

TEM images of structures resulted by aggregation in water of fulleropyrrolidines 1 (left), 2 (middle) and 3 (right).
Mainly the porphyrin–porphyrin, porphyrin–C60 and C60–C60 interactions are the driving forces of the self-assembly of these systems. In addition, the self-organization of fullerene-based materials from tubules to spheres shows that indeed the morphology of aggregates can be controlled in a fine way [8].
The existence of C60 and porphyrin in the same molecule creates an attractive synergism for the fabrication of high-quality electrodes for solar energy conversion. Electrostatic and van der Waals interactions have been used for the deposition of layers containing compound 1 onto indium tin oxide (ITO) electrodes [29]. Such developed electrodes could give rise to improved light harvesting in the visible spectrum.
In summary, we can control the shape of self-assembled superstructurally organized fulleropyrrolidine materials at the nanometer level. Therefore, removal of the porphyrin fragment generates long uniform bundles of aligned parallel nanotubules. Furthermore, removal of the long oligoethylene glycol chains in favour of a short aliphatic one results in spherical aggregates recovering the supramolecular topology of C60 mesoscopic aggregates.
3 Carbon nanotubes
Carbon nanotubes are cylindrical molecules with diameters of as little as 1 nm and lengths up to several micrometers. Thus, not surprisingly, they have been considered as one of the most perfect realizations of one-dimensional physical objects. Since their inception [30], carbon nanotubes have been regarded as materials [31] and most naturally related to the other carbon allotropes – graphite and diamond. Recent work has shown that, with appropriate processing, carbon nanotubes may take on properties that are normally associated with molecular species [32], such as solubility in various solvents. Herein, we summarize the progress made in our laboratories and the promise of the new field of carbon nanotubes chemistry.
SWNTs are extremely resistant to wetting [33]. They typically exist as ropes or bundles; SWNT ropes are entangled together in the solid state to form a highly complex network structure. These phenomena make their dispersion in organic media very difficult. Therefore, derivatization is desirable as it improves both solubility and processibility.
Recently, our group succeeded in functionalizing and thus solubilizing carbon nanotubes [34]. Inspired from the 1,3-dipolar cycloaddition of azomethine ylides to fullerenes [25, 26], a similar methodology was applied to modify carbon nanotubes. Thus, carbon nanotubes were appropriately functionalized when suspended in dimethylformamide (DMF) with an excess of an aldehyde and an α-aminoacid while heated for several days. The so-modified carbon nanotubes were found to be soluble in most common organic solvents, such as chloroform, dichloromethane or acetone, and insoluble in less polar solvents, such as hexane or diethyl ether. The functionalized and thus solubilized nanotubes were also found to be stable in solution as no precipitation was observed upon standing for several weeks. The presence of the added functional groups on the nanotubes was confirmed by 1H–NMR, Raman, IR and UV–Vis spectroscopic characterization. In addition, Transmission Electron Microscopy (TEM) images unambiguously revealed the presence of carbon nanotubes in the functionalized materials.
A general scheme for the 1,3-dipolar cycloaddition of azomethine ylides functionalization of nanotubes is depicted in Fig. 4. As already mentioned previously, variation on the substituent of either the aminoacid or the aldehyde can lead to numerous structurally different functionalized carbon nanotube materials potentially useful in diverse nanotechnological applications.

Functionalization of nanotubes via 1,3-dipolar cycloaddition of azomethine ylides.
Modified SWNTs are associated in bundles or ropes of 50–100 nm of diameter and several micrometers of length. Each of these ropes consists of small bundles of two or three nanotubes or even an individual isolated nanotube. In contrast, pristine SWNTs are usually associated in bundles with a mean diameter of 10 nm (Fig. 5).

TEM images of functionalized nanotubes (left) as compared with pristine ones (right).
There is also great interest in using SWNTs as nanoscale probes in biological electronics because the electronic properties of SWNTs are extremely sensitive to the surrounding environments [35]. The exploration in this exciting area is not yet in full blossom, partially due to the difficulty of preparing water-soluble carbon nanotubes [36]. Very recently, it has been shown that nanotubes can be solubilized in aqueous solution by wrapping process using starch [37] or poly-vinylpyrrolidone [38].
A two-step reaction based on the 1,3-dipolar cycloaddition of azomethine ylides was recently applied to obtain water-soluble functionalized nanotubes, in our laboratories [39]. Thus, when N-substituted aminoacid and paraformaldehyde were added to a suspension of SWNTs (or MWNTs) in DMF and the mixture was heated for several days, properly functionalized nanotubes were isolated by precipitation with diethyl ether. Upon treatment with gaseous hydrochloric acid (HCl) the free ammonium salt on the added N-alkyl chain of the pyrrolidine was released and thus, water-soluble functionalized nanotubes were obtained. Furthermore, the free amino groups of the latter were easily derivatized with protected glycine aminoacid (Fig. 6). In such a way, water-soluble functionalized nanotubes have been obtained and served as intermediate synthons to covalently attach peptides for future biological studies.

Amino acid functionalization of water-soluble nanotubes.
The production of pure single-walled carbon nanotubes (SWNTs) is a matter of great importance. So far, all the approaches led to materials that contain major impurities (amorphous carbon or metal nanoparticles) [40, 41]. We propose [42] a new method for the purification of HipCO SWNTs [41]. The latter consists of the following sequence: (a) organic functionalization of pristine nanotubes, (b) purification of soluble functionalized nanotubes, and (c) removal of the functional groups by thermal treatment at 350 °C. This procedure has the advantage of not using oxidants for purification.
In summary, the development of architectural superstructures in the nanometer level has become possible aided by the spontaneous self-assembly of structurally different fulleropyrrolidines. Numerous novel materials such as the above-mentioned organic functionalized nanotube derivatives or nanocomposites can be synthesized, with a wide variety of properties related to the attached functional group.
Acknowledgements
This work was carried out with partial support from the European Union, Human Potential Network FUNCARS (HPRN-CT-1999-00011), the University of Trieste (Fondi ex 60%) and MIUR (PRIN 2002, prot. 2002032171). The authors thank Dr Fabio Hollan (CSPA, University of Trieste) for mass spectra.