1 Introduction
In the course of our investigations of routes for general and convenient synthesis of amphiphiles derived from sugars, we reported synthesis of catanionic analogs of glycolipids using unprotected carbohydrates. Moreover we developed also amphiphilic polymers and catanionic dendrimers bearing sugar polar heads.
Catanionic surfactants have gathered the attention of researchers because of their various aggregates microstructures obtained spontaneously in water (vesicles, nanodics, icosaedras, helicoidal fibers, liquid crystals) [1].
Catanionic sugar-based amphiphiles enhance biocompatibility of these supramolecular assemblies.
Our synthetic methods for the preparation of such products enabled us to obtain molecules having:
- • sugar heads derived from glucose, lactose;
- • two or three hydrophobic chains;
- • dendritic or polynorbornene cores.
2 Catanionic sugar surfactants
2.1 Anti-HIV catanionic analogs of galactosylceramide
Amongst the variety of glycolipids involved in molecular recognition of pathogenic agents [2] galactosylceramide was identified as an alternative receptor of HIV-1 [3] having a highly specific affinity for the viral glycoprotein gp120 [4]. This discovery opened new perspectives in the field of anti-HIV polytherapies. Recently, we developed and successfully tested in vitro galactosylceramide analogs as chimeras for the virus [5]. The acidobasic reactions performed in water between aminolactitol moieties [6] and fatty acids leads to water-soluble ion pair surfactants the so-called catanionic surfactants (Scheme 1).
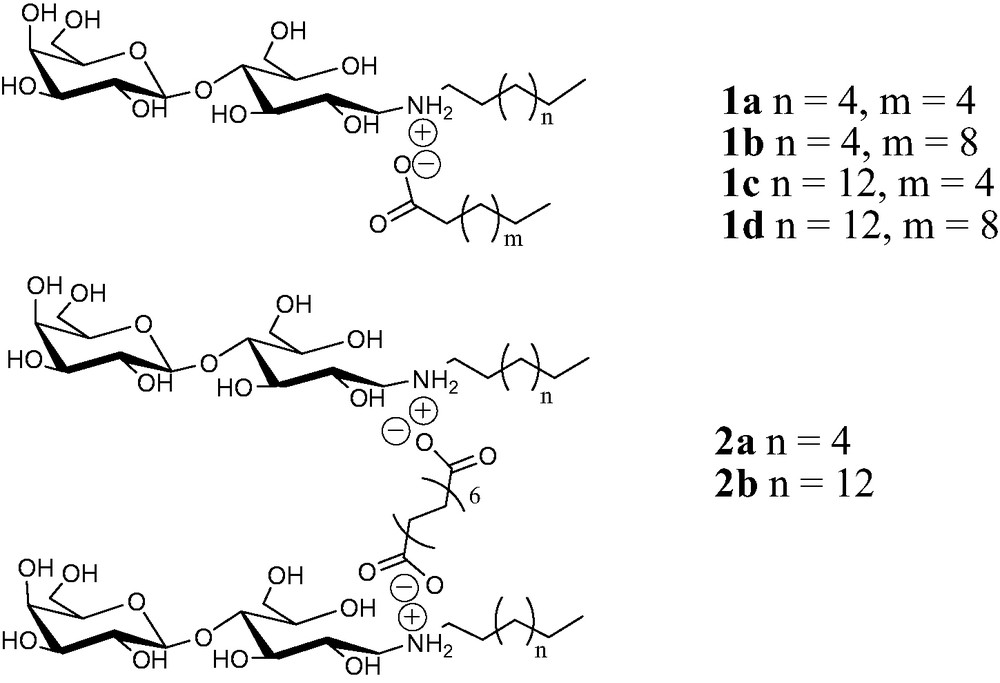
Structure of two-chain 1 and gemini 2 catanionic analogs of galactosylceramide.
Biological in vitro tests are summarized in the Table 1
Activity against HIV and toxicity on lymphocytes CEM
Product | n | m | EC50 (μM) | CC50 (μM) | CAC (μM) |
1a | 4 | 4 | >1000 | >1000 | 50 000 |
1b | 4 | 8 | 100 | > 100 | 2100 |
1c | 12 | 4 | 16 | 38 | 170 |
1d | 12 | 8 | 0.9 | 2.5 | 10 |
2a | 4 | – | 500 | 600 | 2500 |
2b | 12 | – | 0.5 | > 100 | 10 |
All the compounds exhibited an antiviral activity (EC50) efficient concentration for 50% of HIV-infected cells) below their critical aggregation concentrations (CAC), this means in their ion-pair form (compounds 1 and 2), whereas their cellular toxicity (CC50: cytotoxic concentration for non-infected cells) was principally associated to their aggregation state. The highly hydrophobic gemini (2b) exhibited a high anti-HIV activity with a low toxicity for non-infected cells. Within this family of catanionic analogs, this gemini is the only one to have a CC50 above its CAC, indicating that the compound is toxic only in its aggregated state. Catanionic surfactants, and in particular derivatives 1 and 2 are known to spontaneously self-assemble in aqueous solutions. The microscopic structures of these aggregates were elucidated by freeze-fracture electron microscopy [7] (Fig. 1).
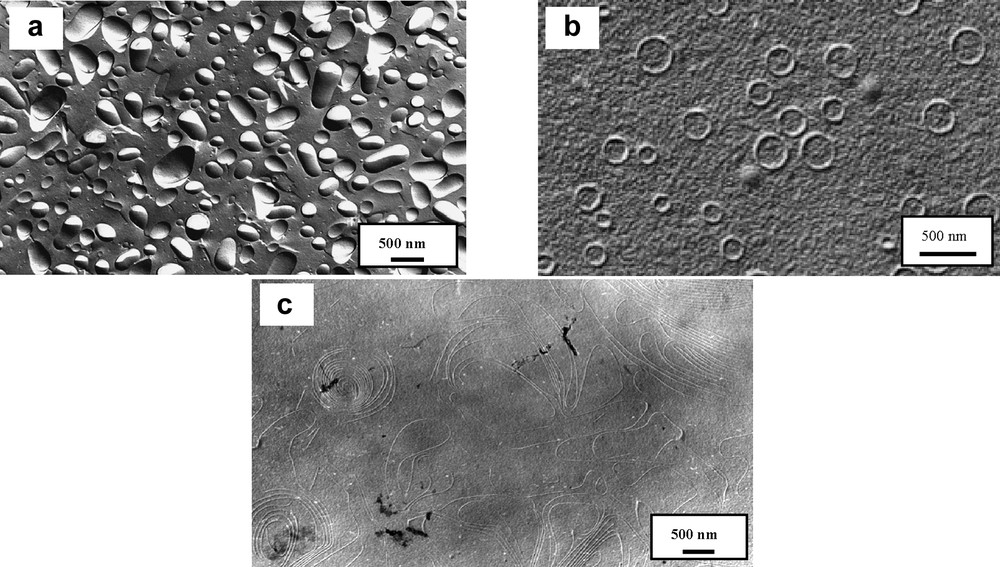
Freeze-fracture images of catanionic amphiphiles (a) two-chain analog 1b (b) gemini 2a and (c) gemini 2b.
It is noteworthy that the aggregation behavior of the gemini 2b is very different from the other compounds, which form vesicles. Gemini 2b gives only long filaments responsible for its low toxicity (this type of aggregates avoid the integration of Gemini 2b in cells membranes [8]). This gemini compound has a very good activity against HIV in vitro and work is now in progress to develop this compound in anti-HIV formulations.
2.2 Vesicles of catanionic analog of galactocylceramide as potential vectors of AZT
The design of drug delivery systems, which require certain physico-chemical and biological properties, has been a continuous challenge for many years. Various microstructures such as liposomes, microgels, and colloids have been widely employed for this purpose. Drugs entrapped into microstructures are expected to be temporarily protected against enzymatic and immunologic reactions of the human body, and may be both decreased toxicity and prolonged time of activity. Furthermore, these microcarriers can be properly modified to deliver the drugs to a specific site.
The microencapsulation technology based on vesicles has been also established. These structures can be loaded with diverse molecules, and constitute promising drug carriers and delivery systems.
We used vesicles obtained with catanionic analogs of galactosylceramide for targeted delivery of anti-HIV drugs like AZT, commonly used in tritherapies. Nevertheless compounds 1 lead to vesicles not enough stable to encapsulate AZT. Therefore, we prepare new families of catanionic amphiphiles bearing a galactose-derived polar head and various phosphinic acids. Modulations were performed on the acid structure (fluorocarbon or hydrocarbon tail bearing a single or double chain) to study aggregate morphologies and stabilities in order to obtain suitable vectors for anti-HIV drugs (Scheme 2).
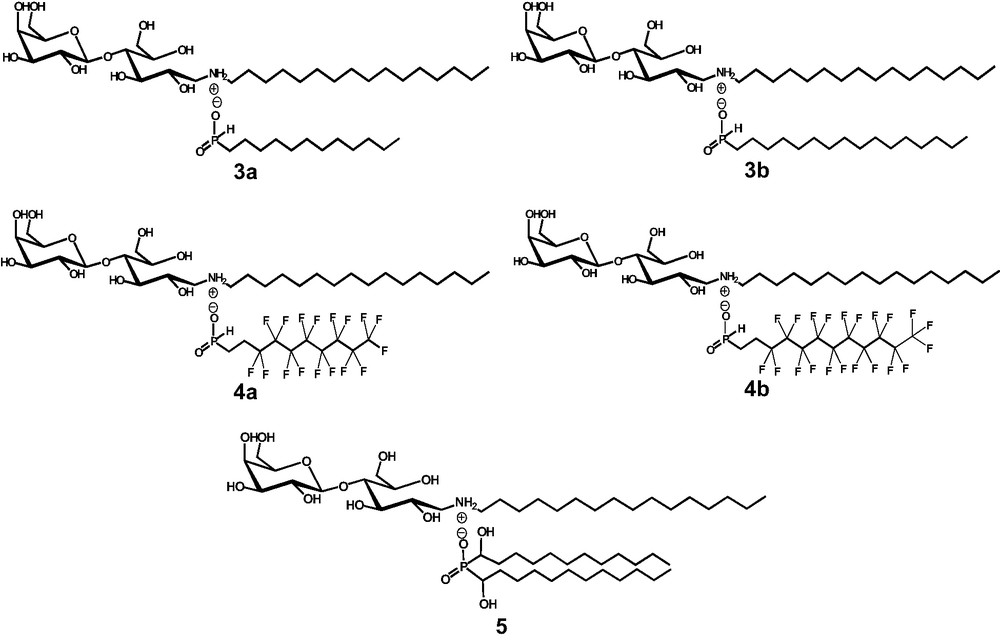
Structure of the various catanionic amphiphilic analogs of galactosylceramide derived from phosphinic acids.
The aggregate morphologies of all surfactants were determined by freeze-fracture TEM. In all cases, spherical vesicles were observed in a wide diameter range. In addition, almost all derivatives form other supramolecular structures. For the compound 3a for example, twisted structures such as helices were obtained (Fig. 2).
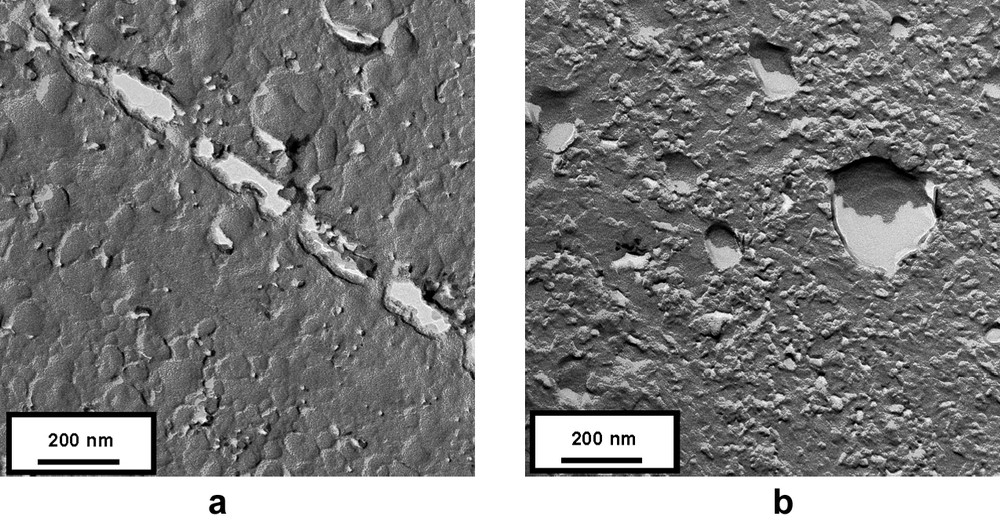
TEM micrographs of freeze-fractured solutions of 3a.
The introduction of a fluorinated hydrophobic part [9] within the bilayer membrane of the vesicle was expected to enhance the homogeneity of the formed aggregate and moreover to increase stability and reduce permeability to drugs, compared to their hydrogenated analogs.
Fig. 3 shows the coexistence or multilayers and small unilamellar vesicles with a diameter of about 20 nm, which might be the result of partial segregation between the hydrophobic tails (fluorocarbon and hydrocarbon). The excess of fluorinated chains in one type of aggregates enhances the rigidity of the membrane giving rise to straight multilayers.
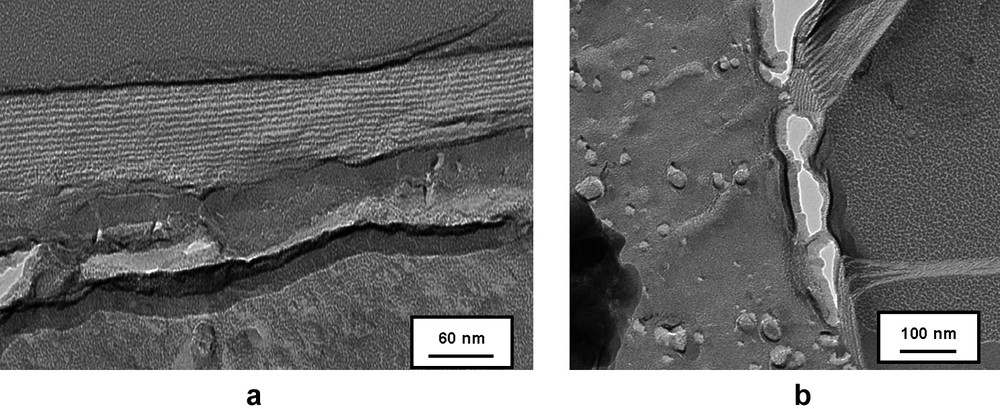
TEM micrographs of freeze-fractured solutions of 4b.
Due to the fact that all theses compounds present such an aggregate complexity, rather than simply vesicles as desired, it did not fulfil the criteria for the proposed encapsulation purpose.
To enhance the hydrophobic effect and the rigidity of the bilayer in the vesicle, a very hydrophobic tricatenar compound 5 was finally prepared. An averaged population of vesicles with a diameter ranging form 20 to 200 nm were observed by freeze-fracture TEM (Fig. 4a). Some images might also display some vesicle fusion phenomenon (Fig. 4b).
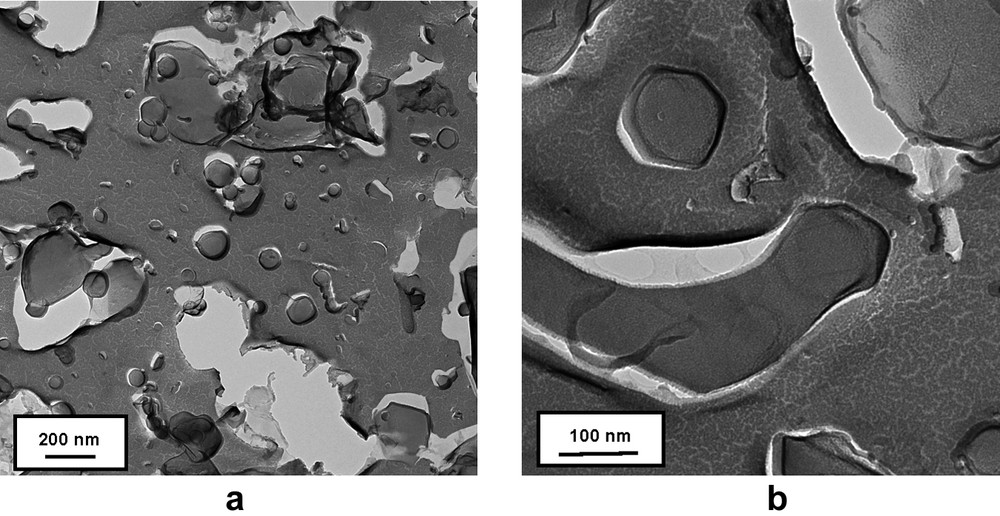
TEM micrographs of freeze-fractured solutions of 5.
From its self-assembling morphology analyses, it seemed that compound 5 formed exclusively vesicles in water and so it appeared to be the good candidate for the encapsulation studies.
It was, therefore, necessary to ascertain the stability of these aggregates, by studying the behavior of solutions at 37°C (human body temperature) in time. The evolution in time of the size distribution for the vesicles formed by compound 5 was studied by dynamic light scattering (DLS). As shown in Fig. 5, the vesicle size distribution appeared to be stable within at least 48 h.
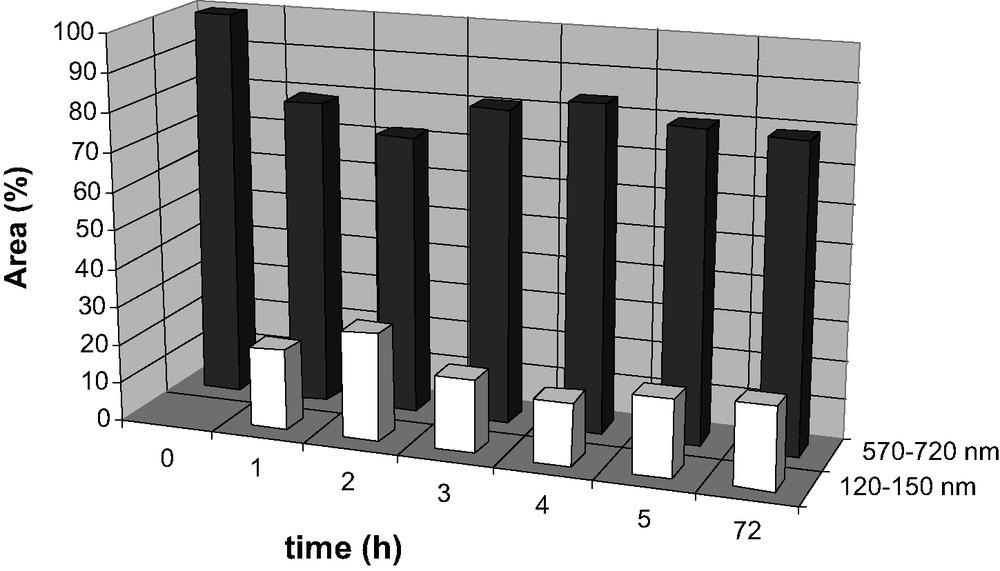
Size distribution of 5 at 37 °C as a function of time.
The most represented population (~700 nm) is quite stable, with an area % (% one-size aggregates) slightly decreasing from 100% at time ‘0’, to 80–70% at 24, 48 or 72 h. This phenomenon might be explained by the tendency of re-aggregation in smaller sized aggregates (~100 nm) until the thermodynamically stable state is reached.
Finally, to quantify the ability of compound 5 to encapsulate AZT, dialysis experiments were carried out. This study was performed at 37 °C so as to predict the behavior of our vectors in physiological conditions. Molar ratio of 5/AZT of 10/1 was employed to quantify the encapsulated AZT. When the dialysis equilibrium (3 h) is reached, the encapsulation percentage of AZT was of ~3.4%, and it seemed to increase up to 24 h (~5.8%). The last measurements at 48 h showed that almost 2/3 of the encapsulated drug has been released by the vesicles (Table 2).
Encapsulation for 5 at different time of dialysis at 37 °C
Time (h) | Free AZT (mmol) | Encapsulated AZT (mmol) | Encapsulation (%) |
3 | 2.65 × 10–4 | 9.3 × 10–6 | 3.4 |
7 | 2.64 × 10–4 | 1.1 × 10–5 | 3.8 |
24 | 2.56 × 10–4 | 1.6 × 10–5 | 5.8 |
48 | 2.71 × 10–4 | 3.5 × 10–6 | 1.3 |
These preliminary results are encouraging and compound 5 displays good properties to be a candidate for anti-HIV drugs vectorization.
3 Catanionic dendrimers bearing galactosylceramide analogs
We decided to investigate the possibility of using dendrimers to build monodisperse catanionic hyperbranched architectures bearing a finite number of amphiphilic galactosylceramide analogs on the surface, namely the aminolactitol moieties. These species could act both as multivalent anti-HIV and as drug carriers thanks to their self-assembly properties. Herein we report a quite unusual stoichiometric self-assembly of galactosylceramide analogs at the surface of acid terminated phosphorous-containing dendrimers as previously synthesized [10]. An example of such catanionic dendrimers is given in Scheme 3.
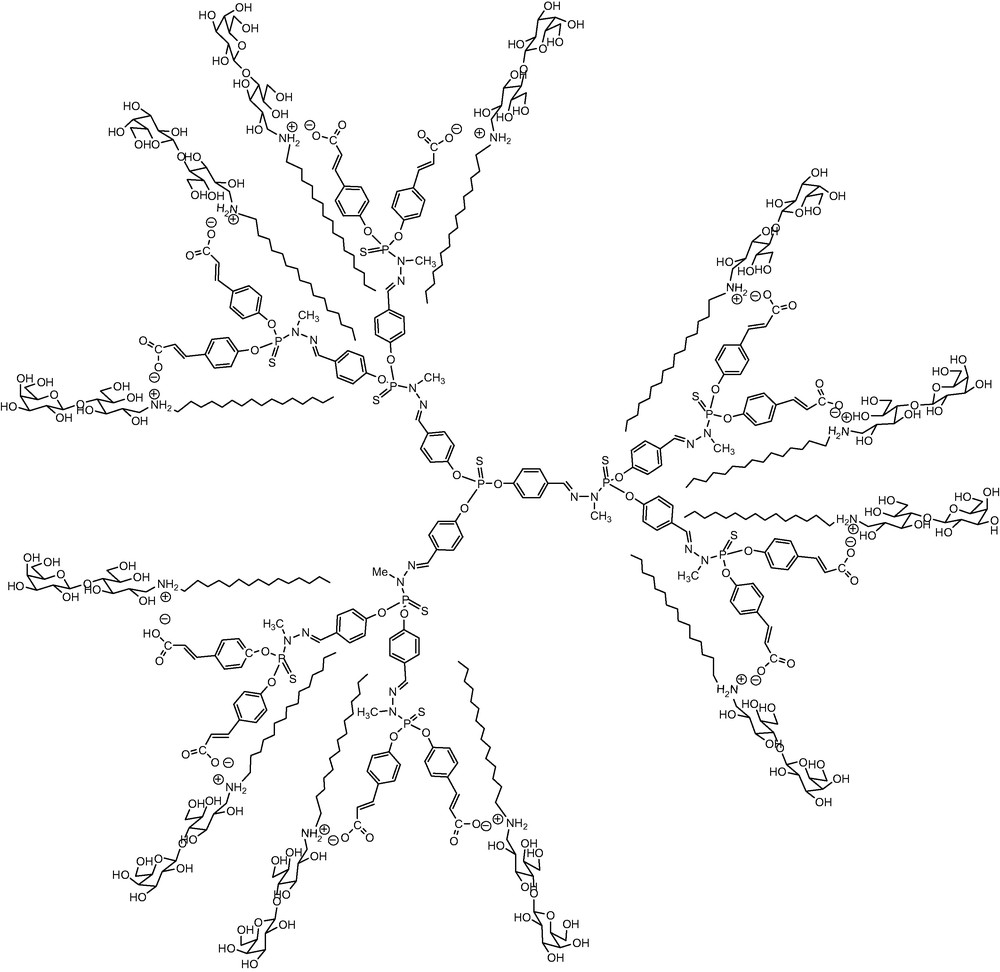
Catanionic dendrimer bearing 6 galactosylceramide analogs.
DLS studies on samples directly prepared by dissolution of the catanionic dendrimer in water show spontaneous aggregates and TEM images show the presence of vesicles (Fig. 6).
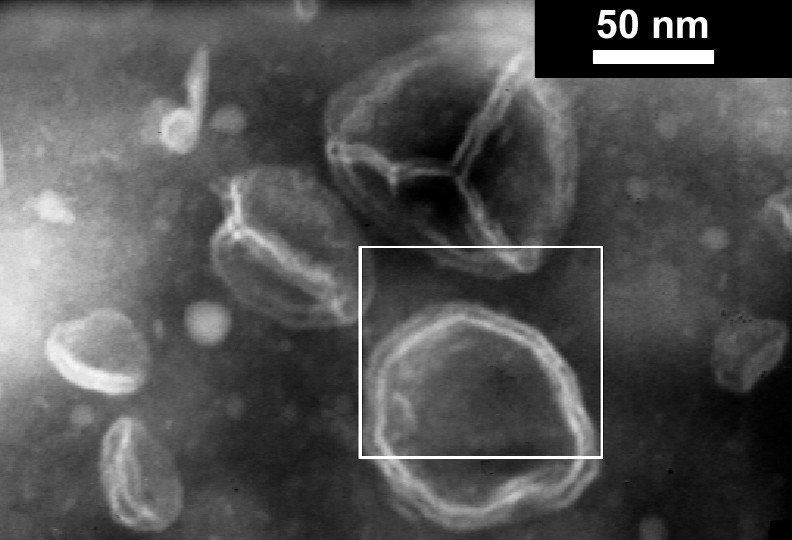
TEM image of 6, catanionic dendrimer bearing 12 galactosylceramide analogs.
The cauliflower-like shape of the hydrophobic dendrimers surrounded by the alkyl chains could be responsible for the original aggregation behavior: catanionic dendritic supra-assemblies organize into spontaneous vesicles. Current investigations involve studies on anti-HIV activity of these catanionic systems to validate our polytherapeutic approach using catanionic dendrimers analogs of galactosylceramide.
4 Amphiphilic polymers bearing lactose moieties
Recently, we described the aggregation properties of a new linear polycationic polynorbornene with acetate counterions [11]. This polymer was formed by the coupling of acetic acid and a neutralized ammonium chloride polynorbornene [12]. It spontaneously forms stable latexes in water and compacts DNA into very small aggregates because of the counterion effect on the hydrophobic packing of the norbornane units.
Receptor-mediated gene transfer is an attractive method to target DNA to specific cellular receptors and/or tissues. Membrane lectins (sugar-specific receptors) have been found at the surface of many cells such as hepatocytes, monocytes, macrophages, lymphocytes, etc. These lectins are good candidates for gene transfer by DNA glycoconjugate complexes as specific carriers [13,14]. Hepatocytes are interesting targets for gene therapy applications because these cells express galactose-specific membrane lectins that may be used as selective receptors to deliver genes into the liver [15,16].
To try to improve the compaction of DNA, but also to elaborate a new vehicle for targeted gene transfer into hepatocytes, we investigated the influence of a sugar counterion like lactobionate, a disaccharide with a galactose subunit.
Therefore, we described an example of sugar counterion, electrostatically bonded to the polymeric backbone. The way of synthesis was summarized in Scheme 4.
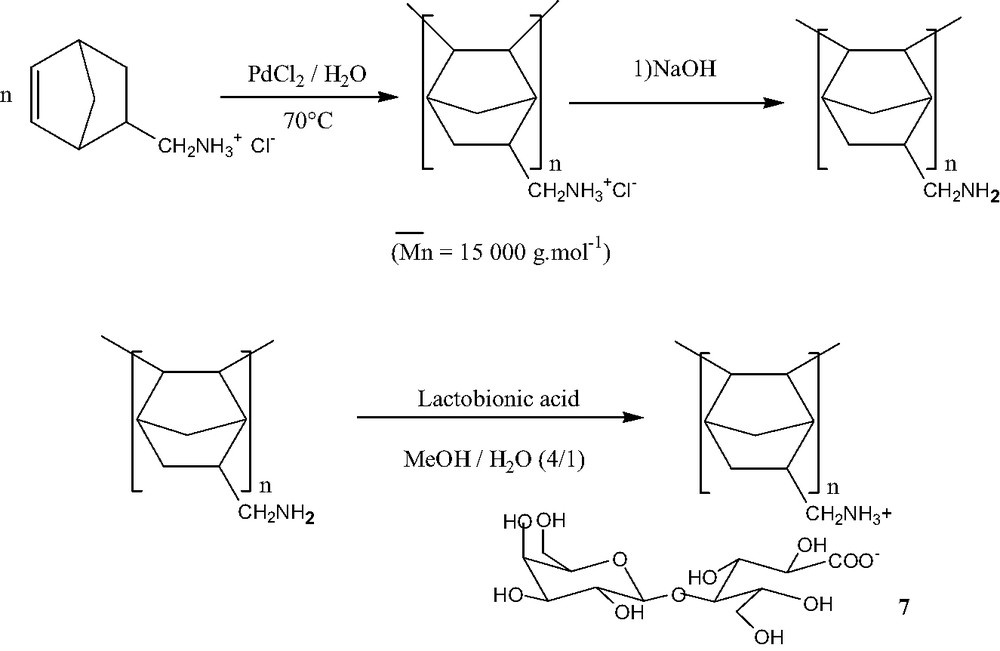
Synthesis of polycationic polynorbornene with lactobionate counterions.
This polymer was observed in water by TEM and uniformaly latex particles were obtained. The latex particles were very small with a diameter of approximatively 20–40 nm for the major part of the population as shown in Fig. 7. And these sizes are in accordance with results obtained by light scattering [17].
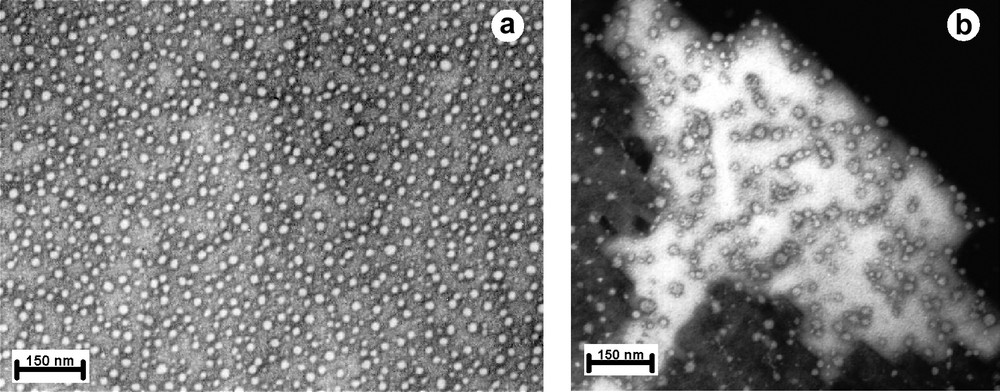
TEM micrographs of lactobionate polynorbornene (A) and DNA–polymer complexes at a NH3+/PO4– ratio of 3 (B).
These latex are therefore able to complex and compact DNA [11]. Lactobionate polymer–DNA complexes were characterized using TEM at NH3+/PO4– rations varying from 0.5 to 3.0. No changes in the size or the shape of the particles were observed. Spherical dense particles with highly compacted structures were obtained with diameters between 10 and 20 nm. At this time, these complexes are the smallest to be obtained without having to use any salt effect.
Compaction of DNA in very small particles is fundamental for in-vivo targeted gene transfer and efficient internalization into cells. It was observed that when the size of the DNA-complex particles exceeded the limit for efficient transport through the capillary system, the DNA was taken up by macrophages from the spleen and the liver. Also, the size of the DNA-complex is critical in the design of a DNA delivery vehicle for receptor-mediated endocytosis, because receptors are not able to internalize ligands above certain size limits [16].
In the case of the polymer–DNA complex, it is difficult to explain the role of the lactobionate ion on the size of the particles.
Before complexation with DNA, the diameter of the particles is approximately 20–40 nm and decreases to 10–20 nm after complexation. This phenomenon may be partly explained by the fact that lactobionate is a large and hydrated counterion weakly attached to the polymer and can be easily exchanged, and consequently the interaction with DNA was more direct then facilitating the reorganization of the complex into small particles. Work is now in progress to investigate DNA vectorization in various type of cells.
5 Conclusion
Original sugar-based amphiphiles (catanionic surfactants, catanionic dendrimers and polymers) were designed and prepared in the aim to obtain new bioactive and/or new formulations of bioactive compound.
Therefore, an amphiphilic gemini compound derived from lactose with an high activity against HIV was obtained. Moreover a tricatenar analog of galactosylceramide was synthesized, leading to stable vesicles that would be useful in vectorization of other anti-HIV drugs. New catanionic dendrimers and amphiphilic polymers also derived from lactose were finally described with different ways of aggregation (vesicles and latex formation depending of the compounds). The polynorbornene latexes are particularly original in the way of their preparation, in their functionalization by sugar (by an ionic way) and finally in their size which can be very small. The biological applications of these new latexes were evaluated and very promising results were obtained in DNA targeting.