1 Introduction
Visible-light active TiO2 films and powder samples have been prepared by nitrogen-doping. According to Asahi et al. [1], TiO2–xNx films were prepared by sputtering the TiO2 target in an N2/Ar gas mixture, and TiO2–xNx powder samples were prepared by treating anatase TiO2 powder in the NH3/Ar atmosphere at 600 °C for 3 h. The TiO2–xNx films and powder samples show visible-light activity. The active sites of N for photocatalysis under visible-light are the substitutional ones that can be identified with the atomic β-N states peaking at 396 eV in the X-ray photoemission spectroscopy spectra. N-doped TiO2 powder samples were also prepared by oxidative annealing of TiN powder for 90 min in an oxygen gas flow at 400 or 550 °C [2]. The substitutional doping of N into the TiO2 lattice was also formed and a significant shift of the absorption edge of a lower energy in the visible-light region was observed.
According to Takeuchi et al. [3], the reduction of TiO2 by the hydrogen plasma treatment created a new absorption band in the visible-light region and showed photocatalytic activity in this region. TiO2–x powder samples were also prepared by reduction treatment using hydrogen plasma with elevate temperatures, and showed visible-light activity [4].
According to Irie et al. [5], TiO2–xNx powders were prepared by annealing anatase TiO2 power under NH3 flow at 550, 575, and 600 °C for 3 h. The prepared powders had nitrogen substituted at some of the oxygen sites in TiO2, which formed a narrow N2p band above the valence band of TiO2. It was determined that the narrow N2p band was responsible for the visible-light sensitivity. When irradiating with visible-light, the quantum yield values decreased as x increased, indicating that the doping sites could also serve as recombination sites.
In this work, a film composed of TiO2 particles are treated with argon plasma and then nitrogen plasma, in series, for making nitrogen-doping to realize appearance of visible-light activity. The nitrogen-doping state of the film is investigated with X-ray photoelectron spectroscopy and X-ray diffraction analyses. Relationship between the nitrogen-doping state and visible-light activity is discussed.
2 Experimental
2.1 Preparation of TiO2 film on glass substrate
Anatase-type TiO2 particles ST01 (Ishihara Sangyo Co., Tokyo, Japan) were used in this work. TiO2 particles were dispersed in 1 M acetic acid solution in concentration of 30 wt.% under ultrasonic irradiation, including polyethylene glycol 40 wt.% vs. TiO2 to make TiO2 sol. The TiO2 sol was spin coated at 4000 r.p.m. on a glass substrate and then was sintered in air at 673 K for 60 min.
2.2 Plasma treatment of TiO2 film
Fig. 1 shows schematic representation of a bell jar-type plasma reactor used for plasma-treating in this work. The glass substrate on which the film composed of the particles was formed was attached on the substrate stage. The stage was rotated at 20 rpm. during the plasma-treating. The film was treated with argon and nitrogen plasmas, in series. The discharge power was in the range of 100–400 W and the discharge time was in the range of 2–20 min. The discharge time of nitrogen plasma was the same as that of argon plasma. The argon plasma treatment was conducted to realize a cleaned surface of the film through removing absorbed water molecules. The reactor was equipped with plasma-monitoring system composed of triple probe monitoring system to measure electron temperature.
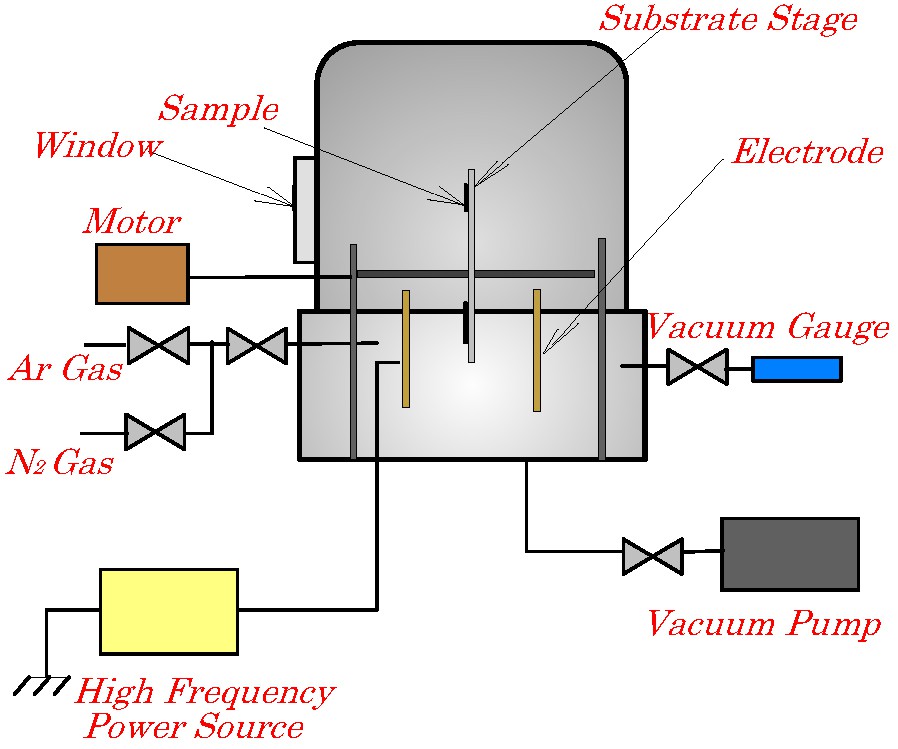
Schematic representation of bell jar-type plasma reactor used for plasma-treating.
2.3 Surface analyses of TiO2 film
An UV–vis diffused reflectance spectrum was measured with a JASCO V-500 UV–vis spectrophotometer (JASCO International Co., Tokyo, Japan) equipped with integral-sphere attachment to clarify a change in light absorbance behavior after the plasma treatments. X-ray photoelectron spectroscopy (hereafter abbreviated XPS) measurement was carried out with a Shimadzu ESCA750 X-ray photoelectron spectroscopy (Shimadzu Co., Kyoto, Japan) to clarify a change in the surface structure after the plasma treatments.
X-ray diffraction (hereafter abbreviated XRD) intensity curves were measured with a Rigaku Rint1200 X-ray diffractometer (Rigaku Denki Co., Tokyo, Japan). After the instrumental broadening was corrected with a quartz standard, the half-value width of the diffraction peak of anatase (101) plane was evaluated to estimate crystal lattice distortion of TiO2 caused by the plasma treatments.
3 Results and discussion
3.1 Analysis of plasma state
Radicals, ions, and electrons are formed in plasma state of non-polymer-forming gases, such as argon and nitrogen, and theses active species are attacked on the surface of the TiO2 film to make chemical reactions. Figs. 2a and b show electron temperatures as a function of discharge power in argon plasma and nitrogen plasma, respectively. The electron temperature of the argon plasma was in the range of 3.0 through 3.2 eV and that of the nitrogen plasma was in the range of 5.0 through 5.9 eV. It can be presumed from the electron temperatures of the argon plasma that the water molecules adsorbed on the surface of the TiO2 film would be removed by the argon plasma treating. The scission of the chemical bond in TiO2 takes place in the nitrogen plasma state of such high electron temperatures. Thus Ti–O bond scission will take place in the surface of the film and N ions will be easily attacked to the surface because of an ion sheath formed in the vicinity of the surface of the film. Therefore, N-doping will take place in the surface layer of the film during the nitrogen plasma treating.
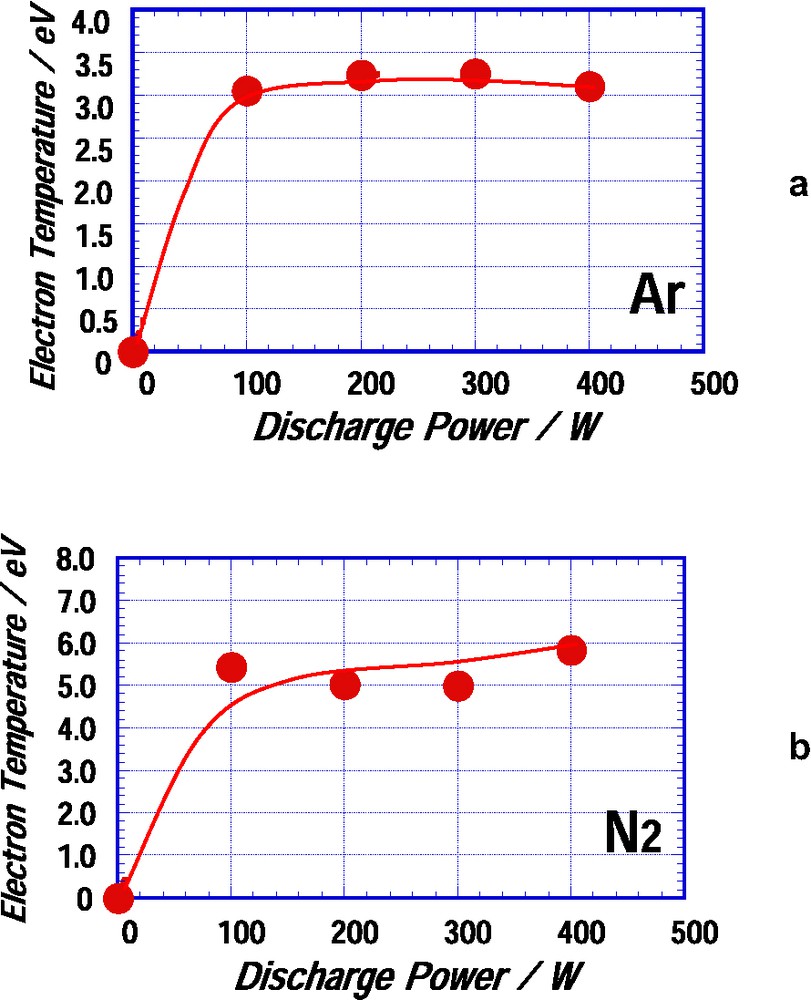
Electron temperatures as a function of discharge power (a) in argon plasma and (b) in nitrogen plasma.
3.2 Surface analysis of TiO2 film
Fig. 3 shows plasma irradiation time dependence of UV–vis diffused reflectance spectrum of the TiO2 film at discharge power of 400 W. The plasma-treated TiO2 films show visible-light absorption in the range of about 400–600 nm and the absorption strength was increased with increasing plasma irradiation time.

Plasma irradiation time dependence of UV–vis diffused reflectance spectrum of TiO2 film at discharge power of 400 W.
Fig. 4 shows the ratio of XPS peak strength, N1s/Ti2p as a function of plasma irradiation time at discharge power of 400 W in the TiO2 film. The ratio was increased with increasing plasma irradiation time and then was decreased above 15 min. This result shows that nitrogen atoms can be doped on the surface layer of the TiO2 film by plasma-treating. A decrease of the ratio in the region of prolonged irradiation time may be originated from formation of surface structure different from TiO2 structure, in which N-doping may be depressed. Fig. 5 shows the ratio of peak strength, N1s/Ti2p as a function of argon etching time in the TiO2 film plasma-treated at 400 W for 5 min. The ratio was abruptly decreased above about 12 min. The argon etching was conducted in the XPS apparatus. The argon etching condition was argon gas pressure of 0.5 mPa, emission current of 20 mA, beam voltage of 2 keV, and ion beam current of 10 μA. Argon etching rate of aluminum was 10 nm/min under the above etching condition, which was observed with a scanning electron microscope. Since sputter etching rates of aluminum and titanium are 1.9 atoms/argon-ion and 1.1 atom/argon-ion, respectively, it is estimated that argon etching rate of TiO2 is about 5.8 nm/min. Therefore the thickness of the N-doped layer would be approximately 70 nm, if porous structure of the film did not take into account. It becomes apparent that N-doping layer of a certain thickness is formed on the surface of the plasma-treated TiO2 film. Furthermore, the film plasma-treated at 400 W for 5 min was annealed at 673 K in air for 60 min to clarify the stability of the N-doped layer. The film lost the visible-light absorption after the annealing. Nitrogen atoms doped into the surface layer of the film were removed by the annealing, since N1s XPS peak was little observed after annealing.
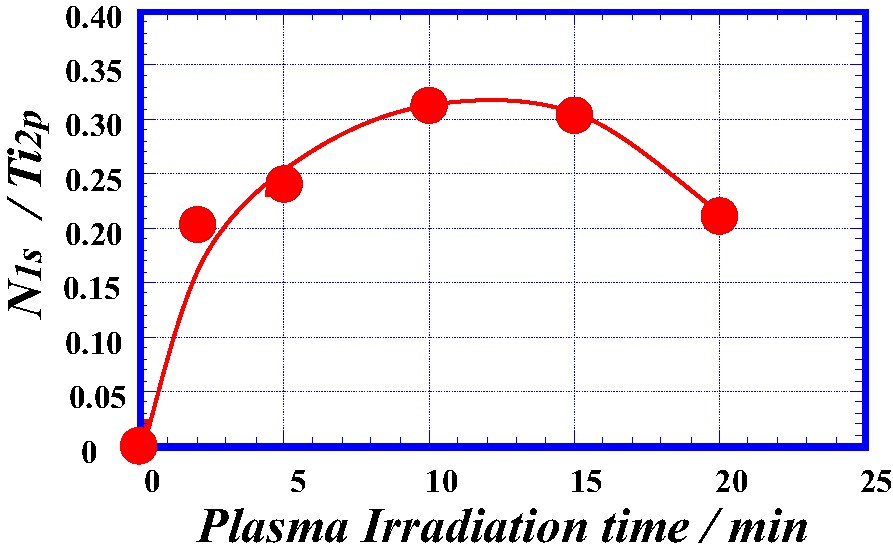
The ratio of XPS peak strength, N1s/Ti2p, as a function of plasma irradiation time in TiO2 film plasma-treated at 400 W.
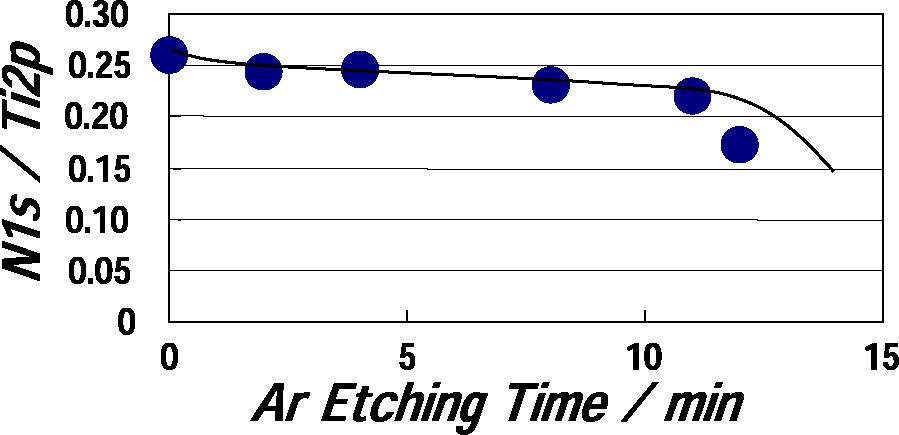
The ratio of peak strength, N1s/Ti2p as a function of argon etching time in TiO2 film plasma-treated at 400 W for 5 min.
Fig. 6 shows N1s spectrum of the TiO2 film plasma-treated at 400 W for 15 min. The peak appeared around 396 eV after plasma-treating is associated with the formation of Ti–N bond. On the other hand, the peaks in the range of 399–400 eV will be associated with N–N and N–O bonds. It is presumed that nitrogen atoms are substituted for oxygen atoms of TiO2 to form Ti–N bonds, or they are introduced in TiO2 to form N–N and N–O bonds. Fig. 7 shows the ratio of the decomposed area of the peak around 396 eV, N1s(Ti–N) to the total area of N1s, N1s(Total) as a function of plasma irradiation time at 400 W. The ratio was decreased with increasing plasma irradiation time. This result means that the substitutional N-doping will preferentially take place in the initial stage of plasma-treating, whereas the interstitial N-doping will preferentially take place in prolonged plasma irradiation time.
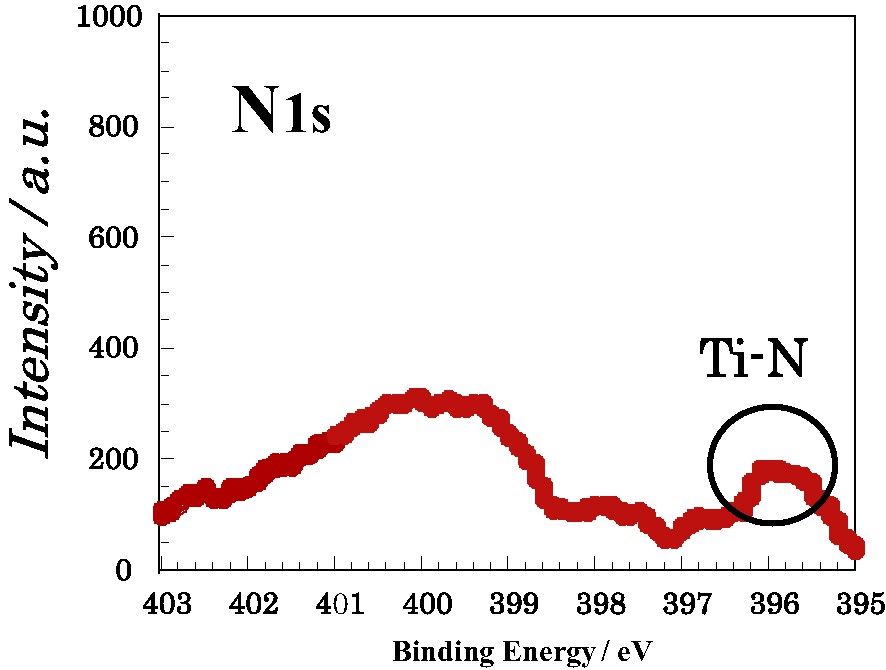
N1s spectrum of the TiO2 film plasma-treated at 400 W for 15 min.

The ratio of the decomposed area of the peak around 396 eV, N1s(Ti–N) to the total area of N1s, N1s(total) as a function of plasma irradiation time at 400 W.
3.3 XRD analyses of TiO2 film
Fig. 8 shows XRD curves of the TiO2 film before and after plasma-treating at 400 W for 20 min. Peak positions of the curves were not changed and the crystal structure which is anatase-type was not changed by plasma-treating. Fig. 9 shows half-value width of anatase (101) peak as a function of discharge time in the TiO2 film plasma-treated at 400 W. The half-value width was increased with increasing discharge time, but was little done above about 10 min. An increase in half-value width will be originated by crystal lattice distortion caused by N-doping.

XRD curves of TiO2 film (a) before and (b) after plasma-treating at 400 W for 20 min.
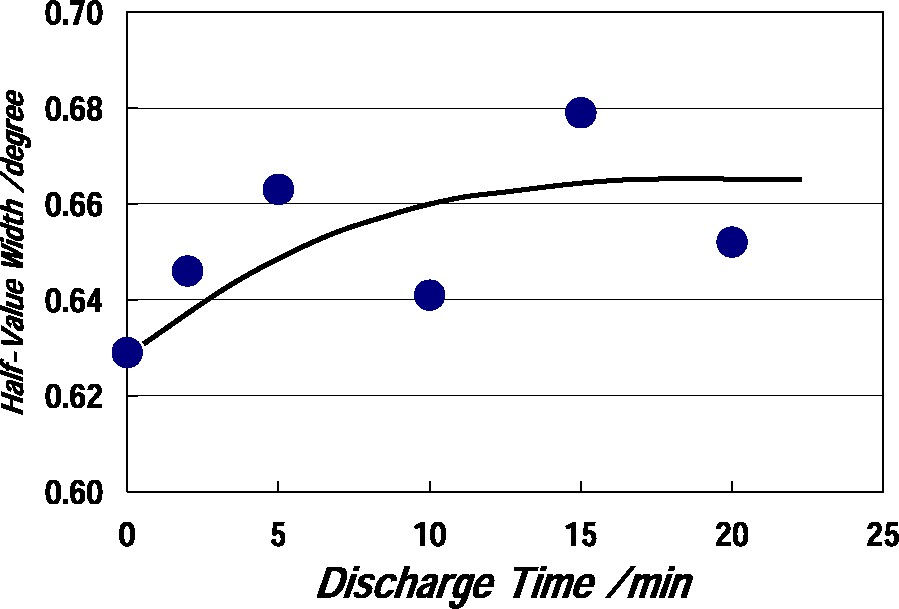
Half-value width of anatase (101) peak as a function of discharge time in TiO2 film plasma-treated at 400 W.
3.4 Photocatalytic properties of plasma-treated TiO2
Fig. 10 shows a decrease in absorbance at 664 nm, Δabs of methylene blue adsorbed on the surface of the TiO2 film as a function of irradiation time of visible-light. A 500 W Xe lamp as the light source and the two cutoff filters for UV region (wavelength λ < 420 nm) and IR region (λ > 750 nm) were used to obtain the visible-light. The Δabs after plasma-treating was more largely decreased with irradiation time of the visible-light, compared with that before plasma-treating. It becomes apparent that visible-light active TiO2 can be prepared by the plasma treatment. Fig. 11 shows the Δabs of methylene blue adsorbed on the surface of the TiO2 film as a function of plasma irradiation time at 400 W. The methylene blue adsorbed on the film surface was irradiated with the visible-light for 3 h. The Δabs was increased by plasma-treating. However the Δabs became larger in the initial stage of plasma-treating.
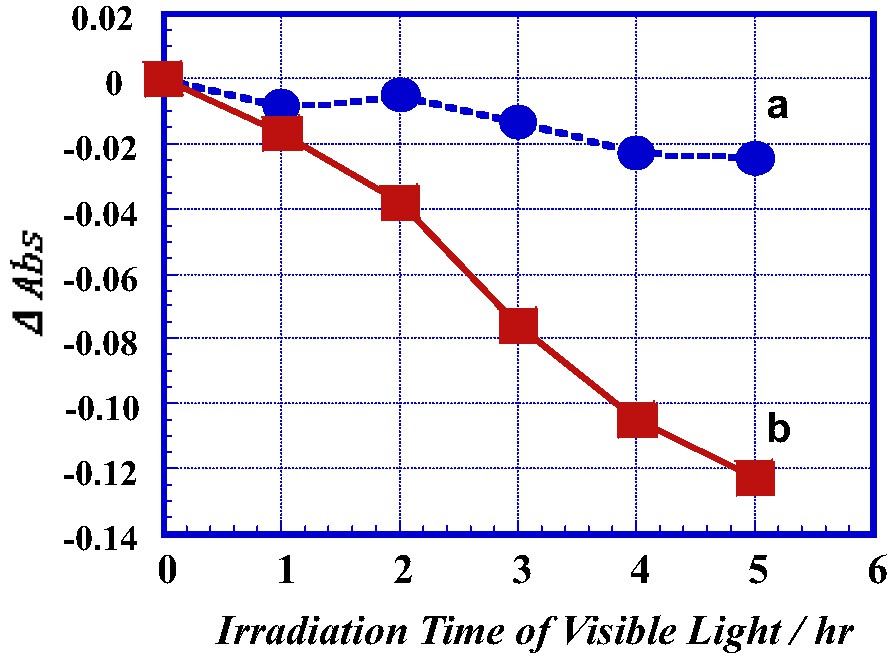
A decrease in absorbance at 664 nm, Δabs of methylene blue adsorbed on the surface of TiO2 film as a function of irradiation time of visible-light (a) before and (b) after plasma-treating at 400 W for 10 min.

Δabs of methylene blue adsorbed on the surface of TiO2 film as a function of plasma irradiation time at 400 W. The irradiation time of visible-light was 3 h.
Fig. 12 shows the Δabs of methylene blue adsorbed on the surface of the TiO2 film as a function of the decomposed area of the peak around 396 eV to the total area of N1s, N1s(Ti–N)/N1s(total) at discharge power of 400 W. The value of Δabs was inclined to increase with increasing the ratio, N1s(Ti–N)/N1s(total). This result means that the decomposition of methylene blue under the visible-light will be mainly originated by substitutional N-doping.
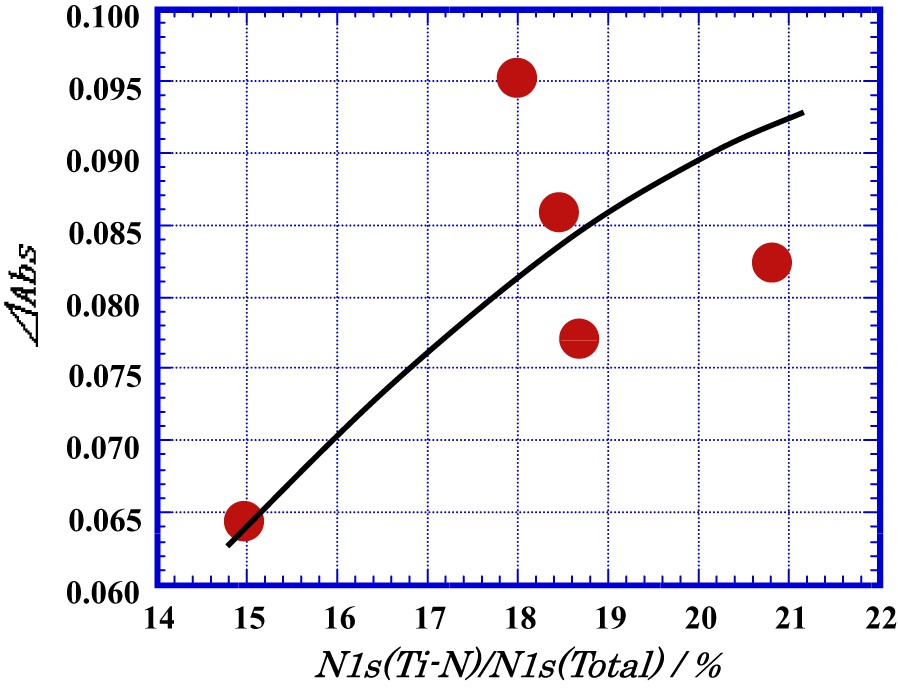
Δabs of methylene blue adsorbed on the surface of TiO2 film as a function of the decomposed area of the peak around 396 eV to the total area of N1s, N1s(Ti–N)/N1s(total) at discharge power of 400 W.
4 Conclusion
N-doped TiO2 particles are prepared with argon and nitrogen plasmas, in series. The amount of nitrogen doped in the film composed of TiO2 particles depends on plasma irradiation time at discharge power of 400 W. The substitutional N-doping and the interstitional one will take place preferentially in the initial stage and in the prolonged stage of plasma-treating, respectively. The TiO2 film having Ti–N bonds formed by the substitutional N-doping shows visible-light activity.
Acknowledgments
This work was supported by a Grant-in-Aid for Scientific Research on Priority Area (417) from the Ministry of Education, Culture, Sports, Science and Technology (MEXT) of the Japanese Government.