1 Introduction
Cassava and maize are the major sources of natural starch products. Starch is a morphologically complex polymer substance, consisting of loose amorphous regions that are interspersed with highly regular crystalline regions, resulting from the formation of hydrogen bonds between the starch molecules. The crystalline composition consists of around 15–45% of the starch granules [1,2]. The scope of starch application is both enhanced and limited by its unique molecular structure. For instance, the compact arrangements of molecules in the crystalline regions inhibit water or chemical reagents from making contact with the molecules in the crystalline region. As a result, the gelatinization temperature is higher and chemical reactivity of starch is decreased. For many applications, the market prefers starch with less extensive crystalline regions, resulting in improved physicochemical properties and increased reactivity for planned applications. Therefore, there is great interest in methods destined to modify the structure in the crystalline region, or decrease the size of crystalline regions [3,4]. The major methods of decreasing starch crystalline regularity include chemical processes [5–7], such as acidolysis, oxidation, enzymatic degradation processes and physical processes [8–11], such as heat-moisture treatment, radioactive degradation, microwave degradation, ultrasonic degradation and extrusive degradation processes. In addition, ball milling refers to the use of friction, collision, impingement, shear or other mechanical actions to modify the crystalline structures and properties of the solid granules. During ball-mill treatment, the chemical reactivity is enhanced because some mechanical energy is converted into internal energy of the substance [12]. Currently, ball milling is used in a variety of applications, including nanomatrix composites, dispersed alloy materials, metallic refinery, ore treatment, waste disposal, and synthesis of organic materials [13–17].
In this paper, we report the application of ball milling in modification of starch. Cassava and maize starches were mechanically treated with a customized stirring-type ball mill. The key research contents are as follows:
- (1) the crystal structure and thermal properties of ball-milled cassava starch (BMCS) and maize starch (BMMS) were respectively investigated by using X-ray diffractometry and differential scanning calorimetry;
- (2) the ball-milling treatment effects on physicochemical properties of cassava and maize starches were investigated by analyzing the influence of ball-milling treatment on the apparent amylose content, cold-water solubility and transparency.
2 Experimental section
2.1 Ball-milling treatment of starch
The ball-milling treatment in this study was achieved with a customized stirring mill driven by a commercially available drill press equipped with a variable speed motor (Fig. 1). The motor turned a shaft that was fitted with four paddles for mixing starch (acquired from Mingyang Biological Inc. Ltd., Guangxi, China) and 6-mm mill balls inside a stainless 1200 ml steel chamber, whose temperature was maintained at a constant level. The mill was stopped and the balls removed after the designated time. The resulting samples were sealed for storage and analyzed immediately.
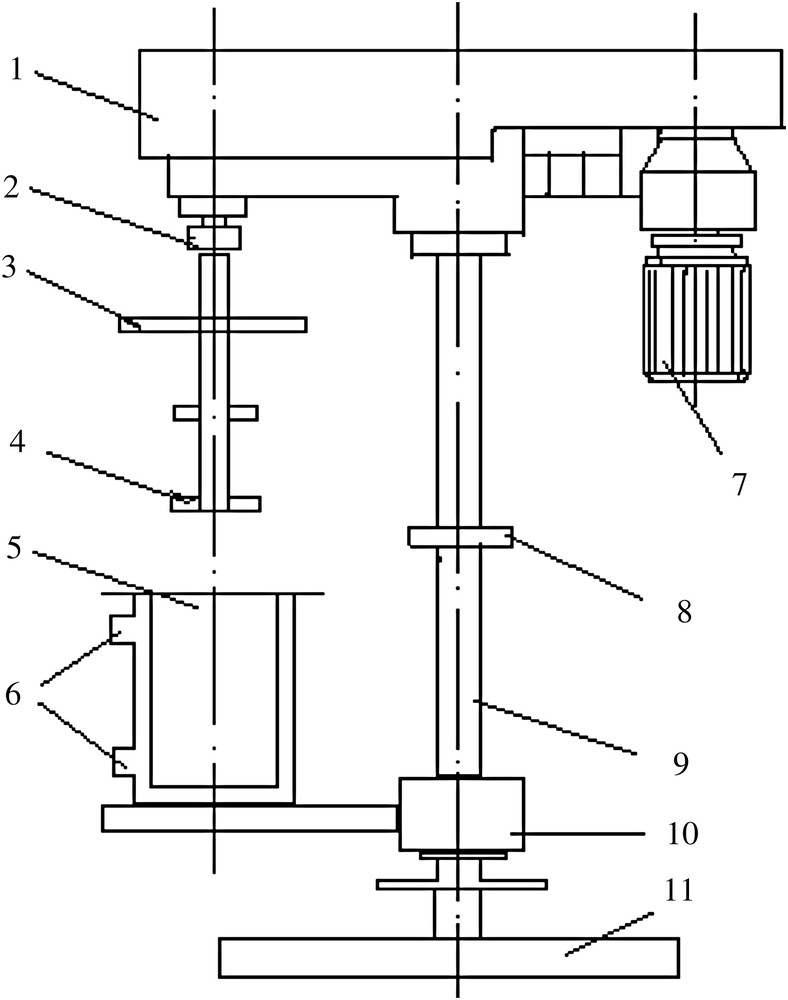
Scheme of the ball mill apparatus. (1) Headstock, (2) shaft coupling, (3) cover board, (4) stirring paddle, (5) grinding canister, (6) entrance and exit of constant temperature water, (7) adjustable-speed motor, (8) stop collar, (9) pillar, (10) hoisting device and (11) pedestal.
2.2 X-ray diffractometry
The X-ray diffraction patterns of the starch samples were measured using a Rigaku D/MAX 2500 V diffractometer (Japan) under the following conditions: Cu Kα radiation, Ni filter disk, 40 kV, 30 mA and measurement range 2θ = 3–40°.
2.3 Differential scanning calorimetry
The starch samples were studied using a DSC-6200 differential scanning calorimetry (PerkinElmer Co., USA). A 4.0-mg (dry weight basis) sample was placed in an aluminum case and deionized water added to obtain a starch/water ratio of 1:4 by weight. The sample was sealed and equilibrated for 4 h. Then it was scanned from 30 °C to 150 °C using a scanning rate of 10 °C/min, while nitrogen gas flowed through the sample chamber at the rate of 30 ml/min. The characteristic temperatures of the transitions were recorded as onset temperature (To), peak temperature (Tp), and conclusion temperature (Tc).
2.4 Apparent amylose content
Apparent amylose of starch samples was determined by the method given by Williams et al. [18].
2.5 Cold-water solubility
An approximately 2.0000-g (dry weight basis) sample was dissolved in 100 ml deionized water. Then the solution was heated to a constant temperature (30 °C or 40 °C) for 20 min, with continuous stirring in order to avoid agglomeration. The solution was centrifuged at 3000 rpm for 20 min. The supernatant was removed and evaporated to dryness at room temperature. The resulting residue was placed in a drying oven at 110 °C until constant mass was obtained. The starch solubility was calculated by measuring the weight of insoluble residue.
2.6 Transparency
The transparency of native and milled starch was measured as described by Perera and Hoover [19]. Aqueous suspensions (1%) of samples were heated in a water bath at different temperatures for 20 min with constant stirring. The suspension was cooled for 1 h at room temperature. The transparency was determined by measuring translucent rate at 650 nm against water blank with 721-spectrophotometer (Precise Scientific Instrument Co. Ltd., Shanghai, China).
3 Results and discussion
3.1 X-ray diffractometry
X-ray diffraction spectra of BMCS and BMMS with various milling times are presented in Figs. 2 and 3.
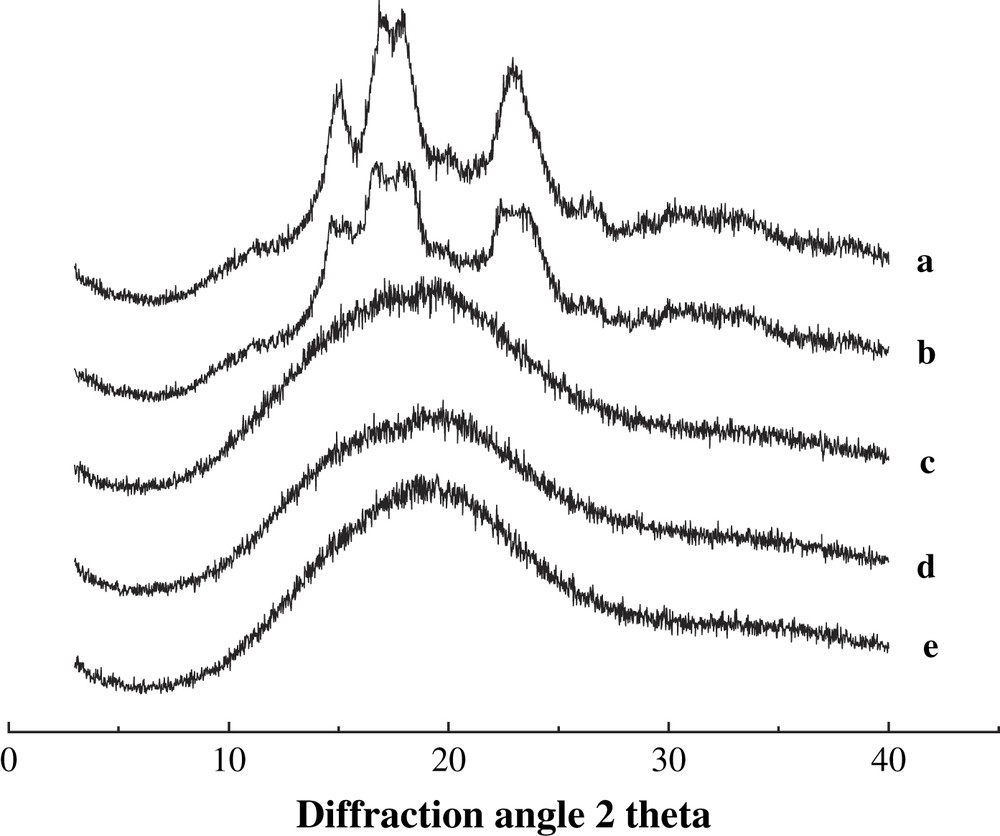
X-ray diffraction patterns of BMCS with different milling times. (a) 0 h, (b) 0.5 h, (c) 1.0 h, (d) 2.0 h and (e) 3.0 h.
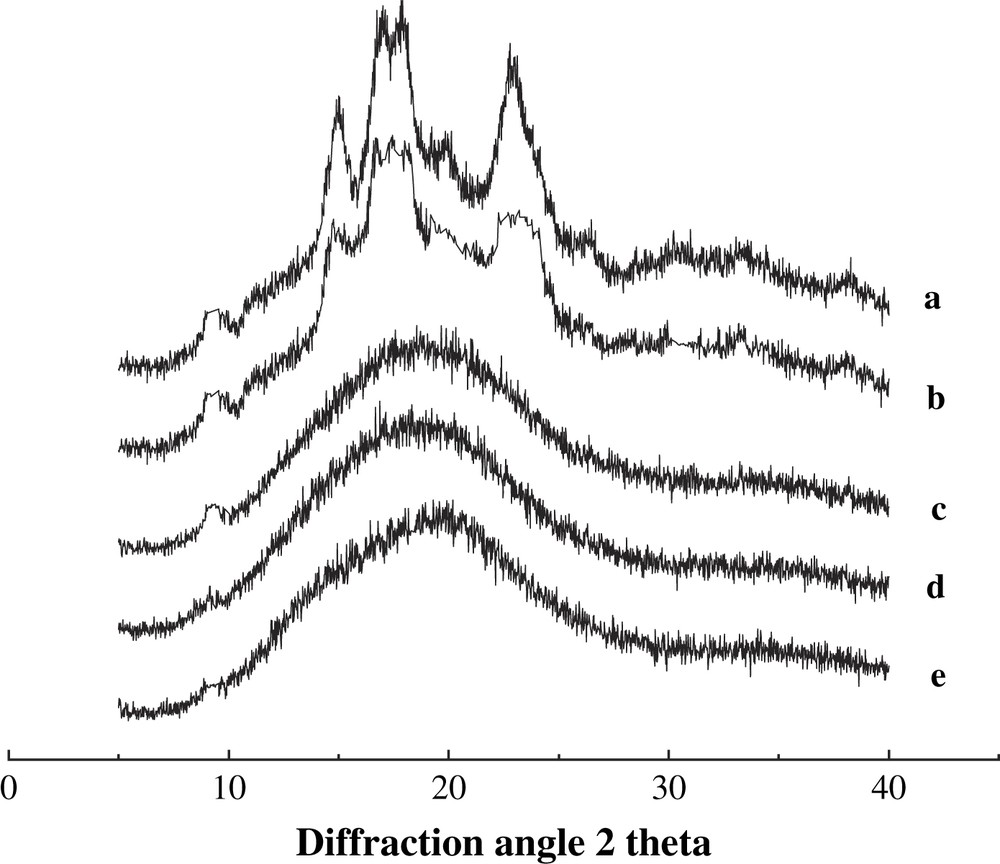
X-ray diffraction patterns of BMMS with different milling times. (a) 0 h, (b) 0.5 h, (c) 1.0 h, (d) 2.0 h and (e) 3.0 h.
The spectrum of the untreated starch sample shows definite diffraction peaks that presumably reflect crystalline regions in starch. The structures of cassava and maize starches are patterns c and a, respectively. As the milling time increases, the regions of amorphism are becoming larger and larger at the expense of the crystalline regions, and the diffraction pattern decreases. After 2 h, the diffraction peak disappears completely, implying that cassava and maize starches have been converted largely into non-crystalline states. Consequently, the diffraction spectrum shows a broad, featureless peak that is a typical spectrum of amorphism. The degree of conversion to the non-crystalline state by the ball-milling method is greater than that observed through heat-moisture treatment [9], radiation degradation [20], microwave degradation [10], and ultrasonic degradation [21]. This work just finished the basic characterization of crystal structure for milled starch. However, the effect of ball-milling treatment on starch crystal structure and degradation at molecular level still needs to be explored.
3.2 Thermal properties
The DSC curves of BMCS and BMMS samples shown in Figs. 4 and 5 are consistent with the foregoing interpretation of the X-ray diffraction data. There are two endothermic peaks between 30 °C and 150 °C, whose parameters are listed in Table 1. To represents onset temperature, Tp peak temperature, Tc conclusion temperature, ΔH endothermic enthalpy of gelatinization and SG the degree of gelatinization [SG (%) = (1 − ΔH1M/ΔH1raw) × 100], where ΔH1M is the gelatinization enthalpy of BMCS and BMMS, ΔH1raw is the gelatinization enthalpy of untreated cassava and maize starches [22]. The endothermic peak 1 appears to reflect the transformations of both from polycrystalline states to non-crystalline states and from granule to gelatinized states when starch was heated. Peak 2, with a high endothermic ΔH value, appears around 100 °C, the boiling point of water, and presumably reflects the vaporization of water in the starch sample and the destruction of the crystalline structure between water and starch.

Differential scanning calorimetry thermograms of BMCS with different milling times. (a) 0 h, (b) 0.5 h, (c) 1.0 h, (d) 2.0 h and (e) 3.0 h.
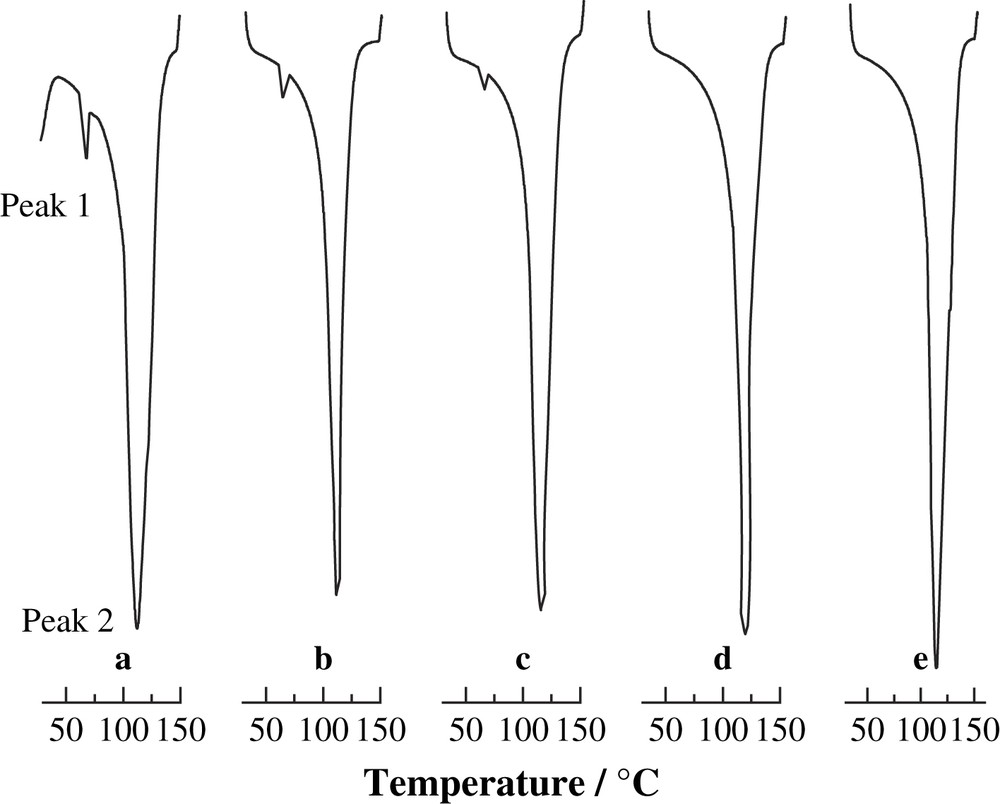
Differential scanning calorimetry thermograms of BMMS with different milling times. (a) 0 h, (b) 0.5 h, (c) 1.0 h, (d) 2.0 h and (e) 3.0 h.
DSC thermal properties of BMCS and BMMS with different milling times
Starch species | Milling time (h) | Peak 1 | Peak 2 | SGa (%) | ||||||
To1 (°C) | Tp1 (°C) | Tc1 (°C) | ΔH1 (J/g) | To2 (°C) | Tp2 (°C) | Tc2 (°C) | ΔH2 (J/g) | |||
BMCS | 0 | 57.9 | 66.5 | 69.9 | 11.5 | 100.3 | 113.5 | 125.6 | 1036 | |
0.5 | 55.1 | 64.1 | 66.0 | 5.8 | 100.6 | 109.8 | 127.9 | 1567 | 49.6 | |
1.0 | 54.8 | 61.2 | 63.5 | 1.8 | 99.8 | 110.0 | 124.5 | 1457 | 84.3 | |
2.0 | No endotherm detected | 101.0 | 113.3 | 129.6 | 1521 | 100 | ||||
3.0 | No endotherm detected | 100.4 | 110.9 | 132.2 | 1542 | 100 | ||||
BMMS | 0 | 62.0 | 69.5 | 72.3 | 10.9 | 99.8 | 114.4 | 133.5 | 1145 | |
0.5 | 60.5 | 64.6 | 70.2 | 4.9 | 98.7 | 110.5 | 127.6 | 1518 | 55.0 | |
1.0 | 58.3 | 63.9 | 67.2 | 1.7 | 99.6 | 112.4 | 128.0 | 1584 | 84.4 | |
2.0 | No endotherm detected | 101.9 | 114.5 | 129.7 | 1602 | 100 | ||||
3.0 | No endotherm detected | 101.8 | 112.0 | 132.7 | 1637 | 100 |
a SG, degree of gelatinization of ball-milled starch.
As revealed by the data in Table 1, as the milling time increases, three temperatures of BMCS' and BMMS' To, Tp and Tc for peak 1 decrease continuously, corresponding to a decrease in the enthalpy of gelatinization and an increase in SG. These data are consistent with the cassava and maize starches' crystalline structure being damaged by the mechanical strength from milling process. After 2 h of the milling time, the endothermic peak 1 from the gelatinization phase transformation disappeared. The SG reaches 100%, indicating that cassava and maize starches have become a completely non-crystalline state. This result is in accordance with that observed in XRD. The somewhat erratic increase in the intensity of peak 2 can be related to the increasing ease with which water molecules can penetrate into starch molecules when the starch crystalline structure is reduced by ball milling.
3.3 Apparent amylose content
The effects of ball-milling treatment on the apparent amylose content of cassava and maize starches are shown in Table 2. The data show that the apparent amylose contents of the BMCS and BMMS were significantly larger than those of their native starches, and the longer the milling time, the more the apparent amylose content. After 1 h, the growing speed of apparent amylose content decreases, indicating that ball-milling preferentially destroys amylopectin. The amount of amylose increases at the expense of destruction of amylopectin during the ball-milling processing, since starch is defined as a mix of two distinct polysaccharide fractions, amylose and amylopectin, both composed of glucose, but differing in size and shape. The amylopectin, consisting of dehydrated glucose unit with α-1,6 glucans connection, is easier destroyed than amylose with α-1,4 glucans connection [1]. It was observed from other researches that the amount of amylose increases through physical or chemical processing, such as heat-moisture treatment [9] and acid degradation [23], which further supports the idea that the increase of the amount of amylose in the processing originates from molecular degradation, the branched structure of starch being destroyed and converted into more amylose. In addition, the extent of the increase in apparent amylose content was larger in cassava starch than that in maize starch during ball-milling process. This may be attributed to the fragile nature of the cassava starch granules. The BMCS had a smaller apparent amylose content than BMMS, which might be due to the differences in the amylose contents of their native starches and granule morphologies, which in turn depend on their biological origin [24].
Apparent amylose content (%) of ball-milled cassava and maize starches with different milling times
Starch species | Milling time (h) | ||||
0 | 0.5 | 1.0 | 2.0 | 3.0 | |
BMCS | 17.01 | 26.38 | 31.26 | 33.70 | 34.83 |
BMMS | 27.24 | 32.56 | 37.18 | 37.94 | 38.31 |
3.4 Cold-water solubility
The effects of ball-milling treatment on the cold-water solubility of cassava and maize starch are shown in Figs. 6 and 7. The data show that the longer the milling time, the greater the cold-water solubility. As revealed in Figs. 6 and 7, the influence of the milling time on the solubility is much larger than that of temperature. The solubilities of the untreated cassava and maize starches are 0.48%, 0.97% and 0.32%, 0.89% when the temperatures are 30 °C and 40 °C, respectively. However, the solubilities at these temperatures of starch activated for 2 h are 93.76%, 93.86% and 38.37%, 42.36%, respectively. Interestingly, milling beyond 2 h had little further effect on the solubility. The observed increase in cold-water solubility of starch with increased duration of milling time is consistent with the model according to which mechanical agitation is capable of degrading the crystalline regions of the starch and allowing greater entry of water into the interior of the starch granule.

Solubility of BMCS with different milling times at different dissolving temperatures. (a) 30 °C and (b) 40 °C.

Solubility of BMMS with different milling times at different dissolving temperatures. (a) 30 °C and (b) 40 °C.
The cold-water solubility of BMCS and BMMS differed significantly. The lower cold-water solubility of BMMS could be attributed to the more rigid structure of the maize starch granules and more amylose content [25]. Cassava starch is fragile in nature and the ball-milling treatment may have affected it to greater extent as compared to maize starch.
3.5 Transparency
The transparencies of BMCS and BMMS with different milling times at gelatinization temperatures of 30 °C, 70 °C and 100 °C are shown in Figs. 8 and 9.

Transparency of BMCS with different milling times at different gelatinization temperatures. (a) 30 °C, (b) 70 °C and (c) 100 °C.
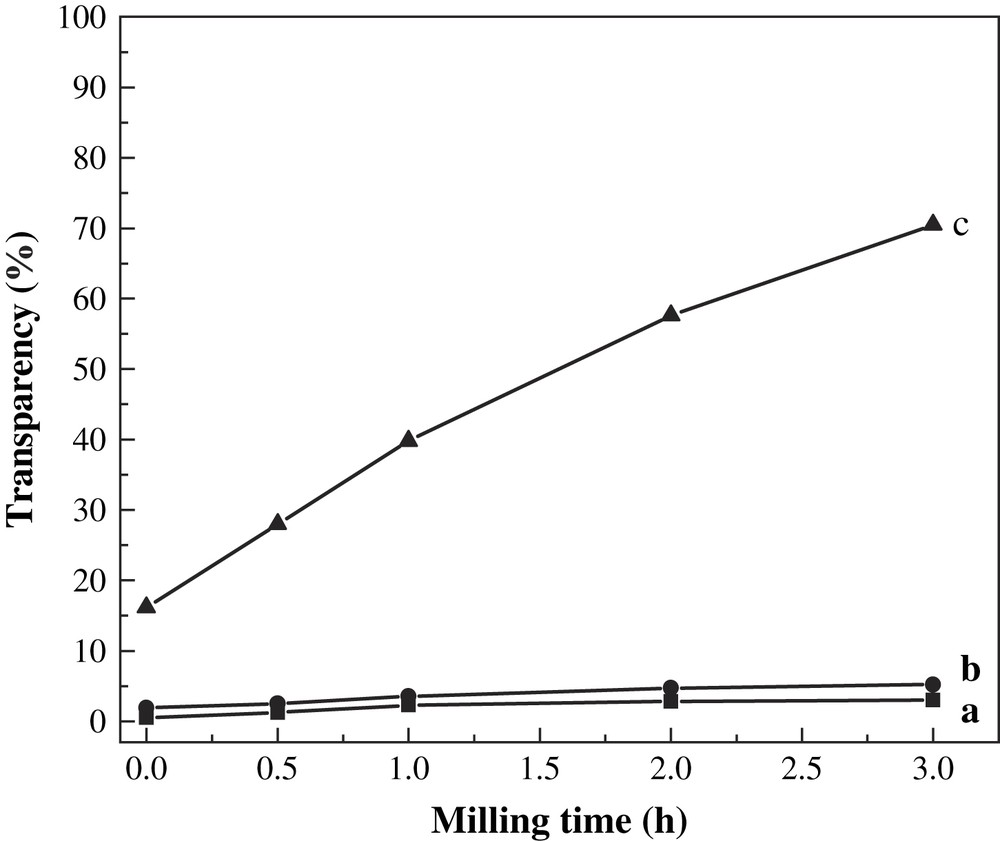
Transparency of BMMS with different milling times at different gelatinization temperatures. (a) 30 °C, (b) 70 °C and (c) 100 °C.
The transparency of gelatinized starch suspension from native cassava and maize starches measured as translucent rate at 650 nm differed significantly. The BMCS and BMMS showed higher transparency than that of their native starch, the longer the milling time, the greater the transparency (Figs. 8 and 9). This may be due to that the crystal structure of cassava and maize starches could be destroyed by ball milling, and then the crystallinity decreased from polycrystalline to amorphous. BMCS showed higher transparency than BMMS under the same treatment conditions, which may be attributed to the differences in granular rigidity, size and amylose of the starches, because cassava starch is of larger size, has a lower amylose content, and is more fragile than maize starch [25].
4 Conclusion
Ball-milling treatment decreases the gelatinization temperature, enthalpy of gelatinization of cassava and maize starches, and increases their apparent amylose content, cold-water solubility and transparency, but the milled cassava starch showed less apparent amylose content, greater cold-water solubility and transparency than that of milled maize starch. Such behavior is consistent with ball milling's increasing the amorphous regions of the starch granules while weakening and decreasing the crystalline regions of the starch. Compared with other methods of bringing about such changes, ball milling has the advantages of being a simple process, presenting minimal environmental problems, and subject to convenient operation. These features make it possible to envision different degrees of degradations for starch for different applications.
Acknowledgements
This work was financed by the National Natural Science Foundation of China (Grant No. 20666001) and the Natural Science Foundation of Guangxi (Grant No. 0640006), China. The authors wish to express their gratitude to Dr. Donald Barnes, guest professor of the Guangxi University, for helpful discussion and grammatical editing.