1 Introduction
Cyanometallates are well known to serve as ligands for Lewis acidic metal centers [1–4]; this area dates back to the synthesis of Prussian Blue and the Hofmann clathrates [5–9]. Inspired by these precedents, researchers have recently learned how to generate molecular cubiodal cages via condensations of cyanometallates and the Lewis acids wherein both bear blocking coligands [10,11]. With certain coligands, one can obtain molecular cages that display distinctive host–guest or magnetic properties [12–15]. The invention of new cyanometallate cages depends on the development of new cyanometallate building blocks [16–19].
In evaluating new cyanometallate building blocks, we focused on the dianions [Fe2(SR)2(CN)2(CO)4]2−, which have been of intense interest as models for the active site of the [FeFe]-hydrogenases [20–22]. Such species exhibit Bronsted basicity, redox activity, and an ambidentate cyanide ligand. In addition to the possibility of incorporated functionality into multimetallic ensembles, such species are attractive building blocks for several reasons: (i) they are readily prepared with a range of thiolate bridges and counter cations [23], (ii) the Fe(CN)(CO)2 vertices are fluxional via a turn-stile rotation (Scheme 1) [24] which allows the cyanide ligands to readily reorient to accommodate the geometric preference of templating Lewis acids, and possibly to correct non-optimally folded cages of the annealing structure and (iii) the Bronsted basicity of the Fe–Fe bond in these dianionic species has been demonstrated. We were particularly intrigued by the prospect of elucidating pathways to potentially multifunctional ensembles that could open the door to the realm of catalytically active coordination cages.

2 Results and discussion
We initially surveyed the ability of [Fe2(S2C3H6)(CN)2(CO)4]2− to serve as a ligand by screening its interaction with several metal ions, using FT-IR spectroscopy as an assay.1 Solutions of [Fe2(S2C3H6)(CN)2(CO)4]2− were found to react with MeCN solutions of Lewis acids such as [Cu(NCMe)(bipy)]PF6 and [Cu(NCMe)4]PF6 in a 1:1 ratio to give soluble adducts. In each case, adduct formation was signaled by shifts in the νCN band, 37 cm−1, to higher energy. A shift in the νCO bands to higher energy was also observed, indicative of a Lewis acid adduct formation [25]. Similarly, the highly lipophilic salt (PPN)2[Fe2(S2C3H6)(CN)2(CO)4] (PPN+ = Ph3PNPPh3+) was found to form 1:2 adducts with ZnTPP (TPP = meso-tetraphenylporphyrin dianion). IR spectra for [Fe2(S2C3H6)(CN)2(CO)4][ZnTPP]22− exhibited νCN band at 2100 cm−1, a shift of 25 cm−1 vs. (PPN)2[Fe2(S2C3H6)(CN)2(CO)4], consistent with the formation of FeCN–Zn linkages.
The preceding condensations clearly indicated the ability of this dimetallic dicyanide as a supramolecular tecton. We therefore directed our attention to the more challenging possibility of generating three-dimensional ensembles. In this case, we turned to the use of Cp∗Rh2+, which is a versatile reagent in stabilizing high molecular weight coordination cages [26]. In the diaxial orientation, the two cyanide vectors in [Fe2(S2C3H6)(CN)2(CO)4]2− define an angle of ∼115°, which should favor the formation of adamantoid or extended tetrahedral cages when reacted with the half-sandwich [Cp∗Rh(NCMe)3](PF6)2 [27].
IR spectral analysis demonstrated that 1.5 equiv [Fe2(S2C3H6)(CN)2(CO)4]2− condensed readily with [Cp∗Rh(NCMe)3](PF6)2 in acetonitrile solution (Eq. (1)). The IR spectrum of the product solution featured a band assigned to νμ-CN at 2108 cm−1, shifted to higher energy vs. starting [Fe2(S2C3H6)(CN)2(CO)4]2− (2075 cm−1). The three νCO bands (1979, 1935, 1913 cm−1) were also shifted to higher energy vs. starting [Fe2(S2C3H6)(CN)2(CO)4]2− (1963, 1922, 1884 cm−1). Titrations indicated that cage formation was strongly favored since the treatment of (NEt4)2[Fe2(S2C3H6)(CN)2(CO)4] with 0.5 equiv of [Cp∗Rh(NCMe)3](PF6)2 gave aggregates and unreacted (NEt4)2[Fe2(S2C3H6)(CN)2(CO)4]. At 1/0.67 ratio of (NEt4)2[Fe2(S2C3H6)(CN)2(CO)4] to [Cp∗Rh(NCMe)3](PF6)2, the IR spectrum indicated the presence of a single condensed aggregate (Fig. 1). ESI-MS analysis of the reaction (PPN)2[Fe2(S2C3H6)(CN)2(CO)4] + 0.67[Cp∗Rh(NCMe)3](PF6)2 showed a strong current at m/z = 811 and 1261, corresponding to {[Fe2(S2C3H6)(CN)2(CO)4]6[Cp∗Rh]4}4− and {PPN{[Fe2(S2C3H6)(CN)2(CO)4]6[Cp∗Rh]4}}3−, respectively.
6[Fe2(S2C3H6)(CN)2(CO)4]2− + 4[Cp∗Rh(NCMe)3]2+ → {[Fe2(S2C3H6)(CN)2(CO)4]6[Cp∗Rh]4}4− | (1) |
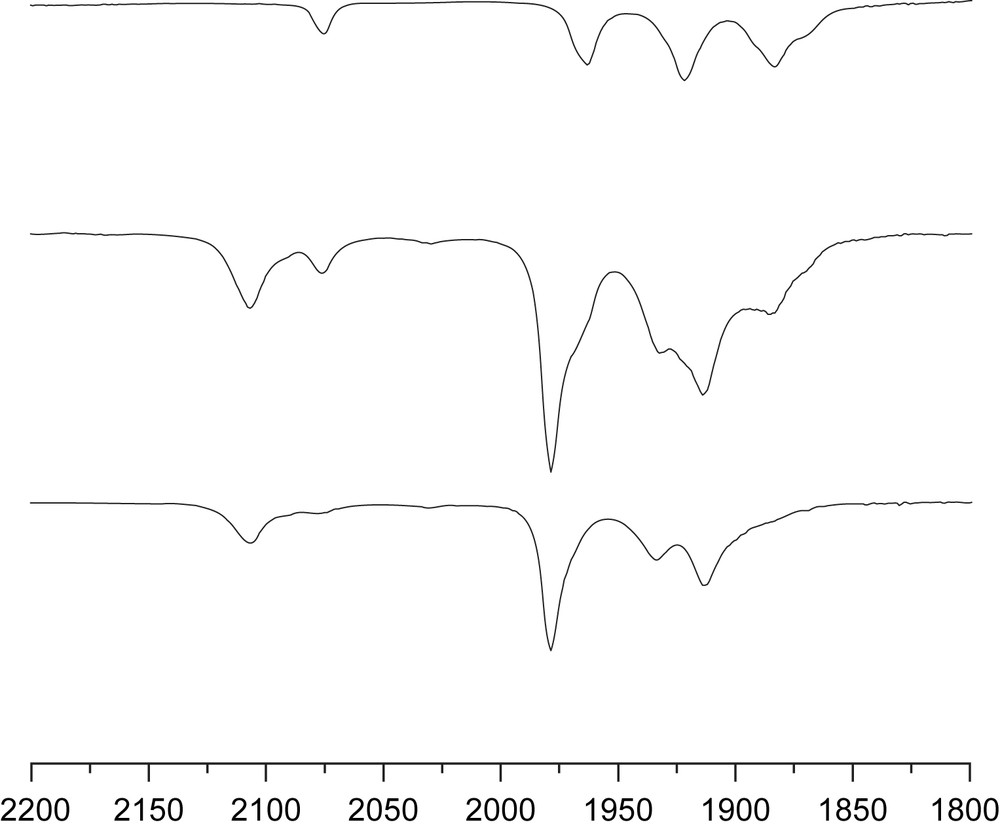
IR spectrum of (NEt4)2[Fe2(S2C3H6)(CN)2(CO)4] (top), (NEt4)2[Fe2(S2C3H6)(CN)2(CO)4] + 0.5[Cp∗Rh(NCMe)3](PF6)2 (middle), (NEt4)2[Fe2(S2C3H6)(CN)2(CO)4] + 0.67[Cp∗Rh(NCMe)3](PF6)2 (bottom). All spectra were obtained in MeCN solution.
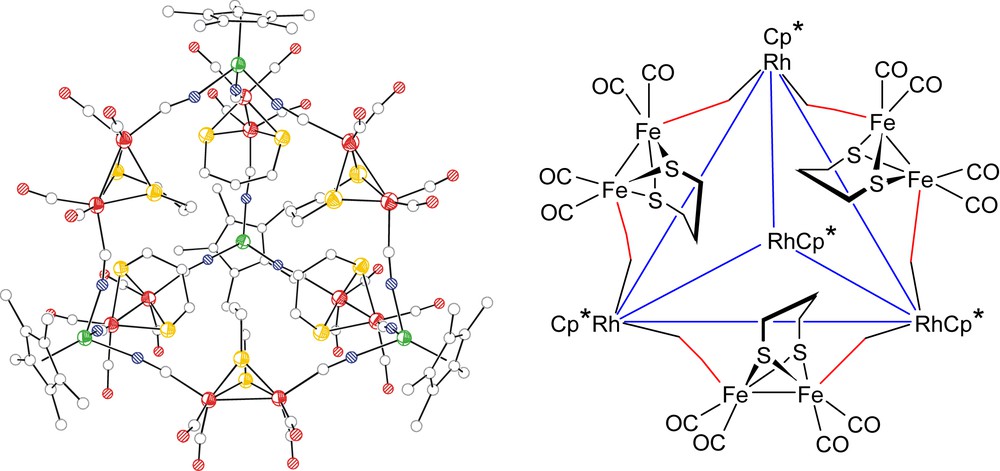
Molecular structure of the anion {[Fe2(S2C3H6)(CN)2(CO)4]6[Cp∗Rh]4}4− (left) and schematic representation of the tetrahedron cage with [Fe2(S2C3H6)(CN)2(CO)4] shown on only one face for clarity. (Right) hydrogen atoms are omitted for clarity. N = blue, C = gray, O = red, S = yellow, Fe = red, and Rh = green. (For interpretation of the references to color in this figure legend, the reader is referred to the web version of this article.)
The 1H NMR spectrum of MeCN solution of (PPN)4{[Fe2(S2C3H6)(CN)2(CO)4]6[Cp∗Rh]4} displayed a large upfield shift (0.5–0.75 ppm) for the methylene protons, consistent with the encapsulation of the dithiolate straps within the tetrahedral cage. The spectrum also indicates the presence of multiple rotatamers, which reinforces the rotational flexibility and complexity of the [Fe2(S2C3H6)(CN)2(CO)4]2− building block.
The condensation of dimetallic dicyanides to give supertetrahedra enjoys some generality as IR spectroscopy indicated that both (NEt4)2[Ru2(S2C3H6)(CN)2(CO)4] and (NEt4)2[Fe2(S2C2H4)(CN)2(CO)4] form 3:2 adducts with [Cp∗Rh(NCMe)3](PF6)2. While [M2(SR)2(CN)2(CO)4]2− has shown to be a verstile building block, the condensed aggregrates were metastable, depositing insoluble materials upon either standing or concentration. The fragility of the aggregrates precluded protonation and catalysis studies.
3 Conclusions
Although cages containing metal–metal bonds are very rare, it is interesting to note that Pd–Pd bonds are found in the very first reported molecular cyanometallate cage, [(C5H4Me)Mn(CO)2(CN)Pd(CO)]4 [30]. Thus, both the present result and the historical record suggest that dimetallic cyanides are promising precursors to new classes of molecular cyanometallate cages. In principle, such cages, provided that they are sufficiently robust, would combine both catalytic and host-guest properties.
4 Experimental section
Reactions and manipulations were performed under a nitrogen atmosphere, using standard Schlenk-line techniques. Electrospray ionization-mass spectra (ESI-MS) were acquired using a Micromass Quattro QHQ quadrupole–hexapole–quadrupole instrument. Infrared spectra were obtained on a Mattson Galaxy Series FT-IR 3000.
4.1 (PPN)2[Fe2(S2C3H6)(CN)2(CO)4]
A solution of 1.44 g (0.0023 mol) (NEt4)2[Fe2(S2C3H6)(CN)2(CO)4] in 7 mL MeOH was added dropwise to a stirred solution of 2.65 g (0.0046 mol) PPNCl in 5 mL MeOH. Addition of 20 mL of degassed H2O precipitated a red oily solid which was recrystallized from MeCN by the addition of Et2O. Yield: 3.026 g (92%). IR (MeCN, cm−1): νCN = 2075; νCO = 1963, 1921, 1883. 1H NMR (CD3CN): δ 1.648 (m, 2H, S2C2H4CH2), 1.839 (m, 4H, S2C2H4CH2), 7.468–7.652 (m, 60H, Ph). Anal. Calcd for C81H66Fe2N4O4P4S2·CH3CN (found): C, 66.44 (66.35); H, 4.64 (4.60); N, 4.67 (4.86).
4.2 (PPN)4{[Fe2(S2C3H6)(CN)2(CO)4]6[Cp∗Rh]4}
A solution of 0.0305 g (0.047 mmol) [Cp∗Rh(NCMe)3](PF6)2 in 10 mL MeCN was added dropwise to a stirred solution of 0.102 g (0.07 mmol) (PPN)2[Fe2(S2C3H6)(CN)2(CO)4] in 15 mL MeCN. Dropwise addition of 50 mL of degassed H2O precipitated a red solid. The deep red product was extracted into 20 mL of MeCN. IR (MeCN, cm−1): νCN = 2107; νCO = 1978, 1934, 1912. ESI-MS (m/z): 811 {[Fe2(S2C3H6)(CN)2(CO)4]6[Cp∗Rh]4}4−, 1261 {PPN{[Fe2(S2C3H6)(CN)2(CO)4]6[Cp∗Rh]4}}3− Crystals were grown in the course of one week at −11 °C from layering a solution of MeCN with hexane and Et2O. Solutions of (PPN)4{[Fe2(S2C3H6)(CN)2(CO)4]6[Cp∗Rh]4} proved metastable with respect to depositing insoluble material upon either concentration or standing. The instability of the tetrahedron cage prohibited the quantitative removal of the PPNPF6 contaminant.
Acknowledgements
This research was supported by the US Department of Energy (DEFG02-90ER 14146). We thank Teresa Prussak-Wieckowska for assistance on X-ray crystallography.
1 IR spectra of the titration of [Cu(NCMe)4]PF6 and [bipyCu(NCMe)]PF6 to [Fe2(S2C3H6)(CN)2(CO)4]2− are given in the supplementary material.