1 Introduction
Catalytic combustion is one of the main processes for the destruction of volatile organic compounds (VOCs) [1,2], using either noble metals [3–5] or metal oxides [6,7] as catalysts. Both families of catalysts have been studied extensively for catalytic combustion applications. Although noble metals are the most active catalysts for VOC abatement [8,9], they have some disadvantages like high sintering rates, volatility, poisoning in the presence of water or sulfur compounds and high price [10]. On the other hand, metal oxides have certain advantages, like price, high thermal stability, higher resistance to poisoning and the easy way of preparation, and they appear to be promising materials. Perovskites are extensively studied for this purpose in particular due to their thermal, chemical and structural stability and in many cases improved catalytic activity [11–13]. Furthermore, their dispersion on supports may lead to increased exposed surface areas [14]. In the present work, we report on results obtained in methane catalytic combustion on unsupported and γ-Al2O3-supported BaTiO3 and PbTiO3 perovskites. To our knowledge, none of the BaTiO3 and PbTiO3 perovskites has been yet studied as catalysts for methane combustion. The difficulty in destroying methane by catalytic combustion, since it is very difficult to activate, makes it a good model compound to test the efficiency of catalysts for VOC combustion, as the catalytic combustion of most organic compounds would be ensured if methane is quantitatively abated.
2 Experimental
2.1 Catalyst preparation
BaTiO3 and PbTiO3 catalysts were prepared from the starting materials Ba(NO3)2, PbO and TiO2, through solid state reactions. Raw materials were weighed out in stoichiometric proportion to form the desired composition and mechanically mixed for 1 h. Then the mixtures were calcined in air at 200 and 400 °C for 1 h at each temperature, at 600 °C for 2 h, and, finally, at 1100 °C for 5 h, for BaTiO3 perovskite and at 800 °C for 6 h, for PbTiO3.
The BaTiO3/Al2O3 and PbTiO3/Al2O3 catalysts, containing 5 wt% perovskite, were obtained by dispersion of BaTiO3 and PbTiO3, respectively, on alumina support. The method of dispersion consists in an original sol–gel process: finely grounded particles of perovskite were added in an aqueous solution of Al(NO3)3 precursor, followed by precipitation with ammonia under intense stirring, at room temperature, up to a pH equal to 5.5. The obtained samples were dried at 100 °C for 1 h and calcined under air at 200, 400 and 600 °C for 1 h at each temperature, and, finally, at 800 °C for 6 h.
2.2 Catalyst characterization
The samples’ structure was investigated by the X-ray diffraction (XRD) method. XRD patterns were recorded with a DRON-2 diffractometer equipped with a Cu Kα source (λ = 1.54 Å). They were recorded over the 10–70° angular range with a 0.02° step and an acquisition time of 1 s per point.
The analysis of elemental surface compositions of BaTiO3 and PbTiO3 was performed by means of X-ray photoelectron spectroscopy (XPS). XPS spectra were recorded at room temperature and under vacuum of 10−9 torr on a VG Scientific ESCA-3 Mk-II spectrometer using monochromatized Al Kα radiation (1486.7 eV). XPS data were analyzed with S_Probe ESCA and XI_SDP32 (XPS International) software programs.
BET specific surface areas of the samples were measured on a Micromeritics ASAP 2020 system under liquid-nitrogen temperature using N2 adsorption.
The morphology of the samples and the dispersion of perovskites on Al2O3 support was investigated using a Philips XL 30 ESEM (Environmental Scanning Electron Microscope) having EDX (Energy Dispersive X-ray) analyzer. An accelerating voltage of 20 kV was used. The powder samples were fixed on a holder and sample compartment was evacuated in order to prevent the electrical charging. Then the surface of particle was photographed and simultaneously quantitative and qualitative electron probe microanalyses were performed. Electron beams were very finely focused. Therefore, elemental analysis of very small area on specimen surface was done in so-called spot mode.
2.3 Catalytic testing
The combustion of methane was carried out in a fixed bed quartz tube down-flow reactor operated at atmospheric pressure. The internal diameter of the reactor tube was 28 mm. The catalyst was supported by quartz wool. The axial temperature profile was measured using an electronic thermometer placed in a thermowell centered in the catalyst bed. Ceramic rings were used to fill the dead volumes before and after the catalyst bed to minimize potential gas-phase reactions at higher reaction temperatures. The reaction mixture consisted of 5% (vol.) methane in air. Flow rates were controlled by fine needle valves and were measured by capillary flow-meters. The volume hourly space velocity (VHSV) was maintained at 16,000 h−1.
In a typical reaction run, the reactor was heated to the desired temperature in the flow of reactants. The system was allowed to stabilize for about 30 min at the reaction temperature before the first product analysis was made. Each run was carried out over a period of 2–3 h, until two consecutive analyses were identical. The reaction products were analyzed in a Clarus 500 Gas-Chromatograph equipped with a thermal conductivity detector (TCD), using two packed columns in series (6 ft Hayesep and a 10 ft molecular sieve 5 Å).
The conversion was calculated as the amount of raw material transformed in reaction divided by the amount that was fed to the reactor. Complete selectivity to CO2 and H2O was always observed.
3 Results and discussion
3.1 Physico-chemical properties of the catalysts
The XRD patterns of the catalysts are displayed in Fig. 1. The alumina support shows the characteristic reflections of γ-Al2O3. For BaTiO3 and PbTiO3 samples the XRD patterns indicate only the formation of perovskite phases with tetragonal symmetry, with no other crystalline compounds. After dispersion on γ-Al2O3, Ba and Pb titanates maintain their structural integrity. No characteristic peaks corresponding to the BaAl2O4 and PbAl2O4 spinel phases were found.
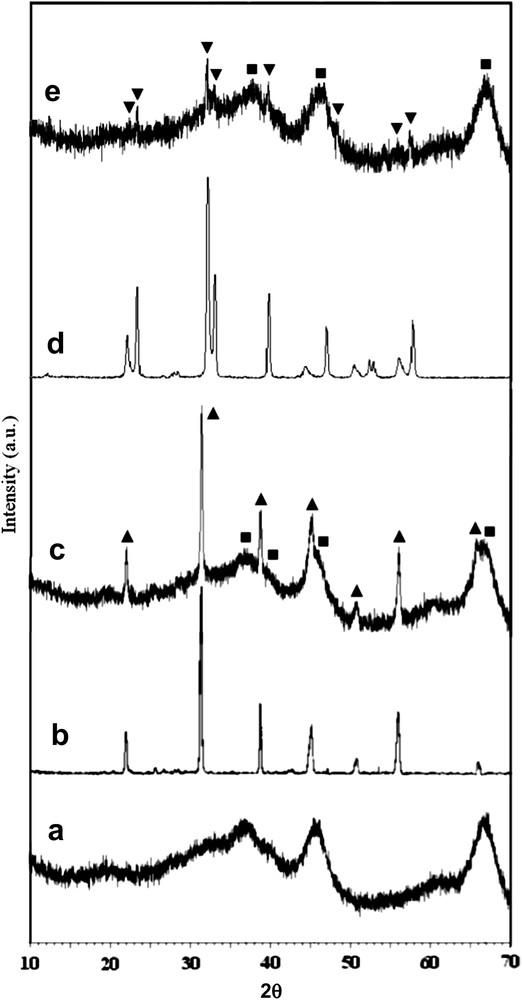
XRD patterns of the catalysts: a – γ-Al2O3, b – BaTiO3, c – BaTiO3/Al2O3, d – PbTiO3, e – PbTiO3/Al2O3 (■ – γ-Al2O3 phase, ▴ – BaTiO3 phase, ▾ – PbTiO3 phase).
Textural properties of the unsupported and supported perovskites and of the γ-Al2O3 support are summarized in Table 1. The specific surface areas of the unsupported perovskites are low, but normal for perovskite-type oxides, especially for those prepared through solid state reaction. At the same time, the specific surface areas of the BaTiO3/Al2O3 and PbTiO3/Al2O3 systems are very high and similar to γ-Al2O3 support. Pore size distributions of the supported samples and γ-Al2O3 support are displayed in Fig. 2. They indicate that all the samples show uniform and narrow pore size distribution with average peak pore diameter for both supported samples at 4 nm and for alumina support at 6 nm.
Textural properties and surface Ti/M atomic ratios of the catalysts (M = Pb or Ba).
Sample | BET surface area (m2/g) | Average pore diameter (nm) | Pore 4volume (cm3/g) | XPS surface Ti/M atomic ratio |
γ-Al2O3 | 191.0 | 5.1 | 0.350 | – |
BaTiO3 | 0.4 | 60.2 | 0.006 | 1.1 |
BaTiO3/Al2O3 | 193.0 | 4.6 | 0.350 | – |
PbTiO3 | 0.5 | 15.1 | 0.002 | 0.5 |
PbTiO3/Al2O3 | 175.0 | 6.4 | 0.270 | – |
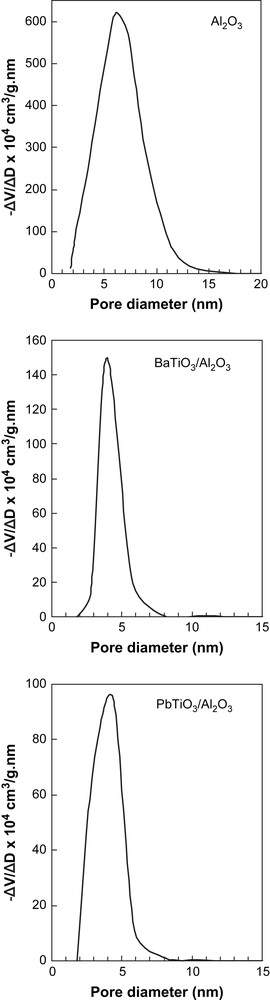
Pore size distributions of the supported samples and γ-Al2O3 support.
The XPS spectra of bulk BaTiO3 and PbTiO3 samples are displayed in Fig. 3. Surface atomic concentrations of the elements in the investigated samples, presented in Table 1 as surface atomic ratios, were determined from the photoemission peaks areas. The peak corresponding to Ba (3d5/2) has two components, as observed by curve fitting, one at about 778.7 eV and another at about 780.6 eV. This indicates the existence of Ba in two different chemical environments at the surface of BaTiO3, related to the ion displacements in the tetragonal structure leading to two types of O2− positions and, consequently, two types of nearest neighbors for Ba2+. This is a characteristic feature of BaTiO3 surfaces [15] associated to ferroelectric domains and c domains, respectively. While for BaTiO3 the surface atomic ratio was roughly equal to the stoichiometric ratio, for PbTiO3 an important superficial excess of lead was observed, but without the segregation of a single lead oxide phase.
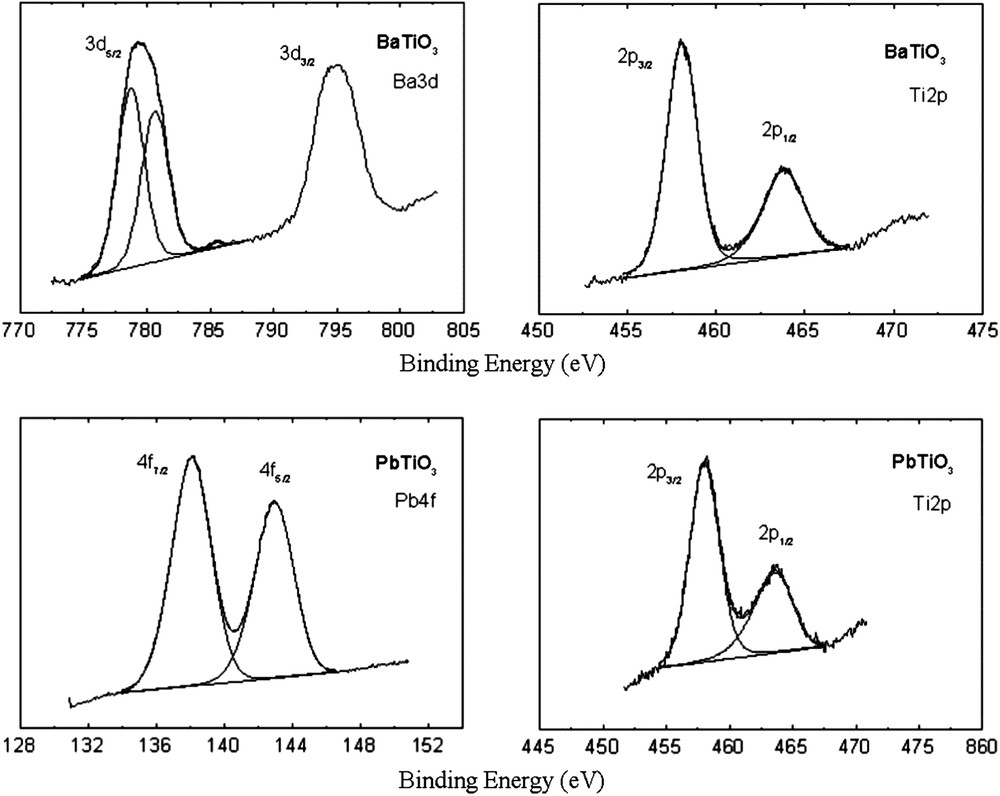
XPS spectra of unsupported BaTiO3 and PbTiO3 samples.
The SEM micrographs for BaTiO3/Al2O3 and PbTiO3/Al2O3 systems are shown in Fig. 4. Islands (bright points) of perovskite with particle size of 1–5 μm covering the alumina surface are observed in both samples. This is confirmed by EDX microprobe analysis focused on “islands” and on the support, the results obtained being presented in Table 2. It can be seen that Ti and Ba or Pb content decreases drastically in points S (the support) with respect to the “islands”. On the other hand, while the Ti/Pb atomic ratio in the PbTiO3 “islands” is close to 1, the Ti/Ba atomic ratio in the BaTiO3 “islands” is close to 2, suggesting that Ba probably migrates from the perovskite to the alumina support to locally form barium aluminate.
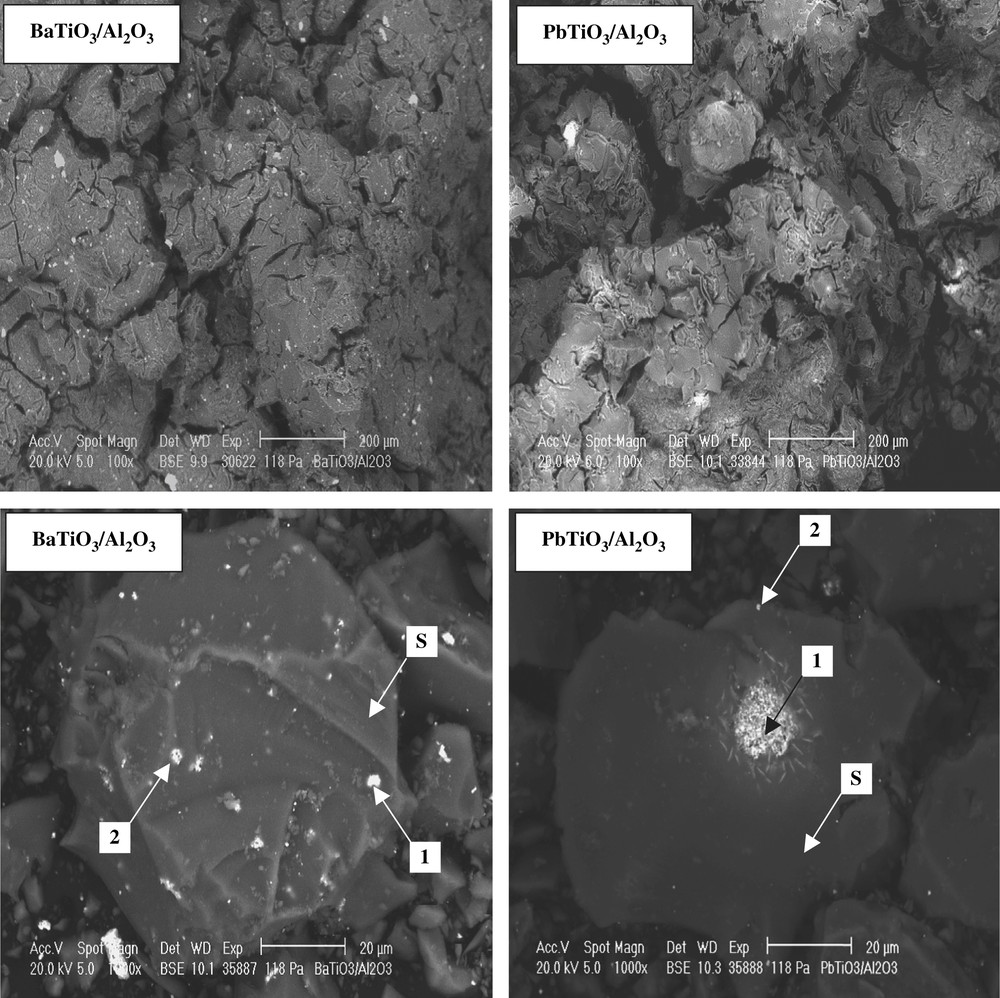
SEM micrographs of BaTiO3/Al2O3 and PbTiO3/Al2O3 systems.
EDX microprobe analysis of the supported catalysts in the points indicated in Fig. 4.
Element | Atom % in | |||||
BaTiO3/Al2O3 | PbTiO3/Al2O3 | |||||
Point 1 | Point 2 | Point S | Point 1 | Point 2 | Point S | |
O | 54.2 | 55.9 | 61.1 | 60.7 | 63.0 | 54.8 |
Al | 35.5 | 34.0 | 38.5 | 27.3 | 30.4 | 43.8 |
Ti | 6.4 | 7.3 | 0.3 | 6.5 | 2.9 | 0.5 |
Ba | 3.9 | 2.8 | 0.1 | – | – | – |
Pb | – | – | – | 5.5 | 3.7 | 0.9 |
3.2 Catalytic properties
The catalytic activities in methane combustion of unsupported and supported perovskites as well as of the alumina support have been determined in the temperature range of 550–800 °C. The only reaction product detected on unsupported and supported perovskites was CO2, while with γ-Al2O3 support a significant amount of CO was found in the products in line with what was reported in Ref. [16].
The results obtained are shown in Fig. 5, where the measured values of methane conversion are plotted as a function of the reaction temperature. At the same time, the apparent activation energies corresponding to the transformation on the different catalysts have been calculated (Table 3) and the Arrhenius plots obtained (Fig. 6). The values obtained for the activation energies are comparable with those presented in the literature [16]. As clearly shown in Fig. 6 and by values of T50 (temperature corresponding to 50% conversion) and of the specific reaction rate in Table 3, unsupported PbTiO3 perovskite is more active than BaTiO3, the last being, surprisingly, less active than alumina. In terms of the specific activity, both perovskites seem to be less active than alumina. This may be due to the very high surface area of alumina compared to unsupported perovskites. On the other hand, both supported catalysts are more active than the corresponding unsupported perovskites, both in terms of T50 values and of specific reaction rates. Moreover, an important increase in the reaction rate per unit mass of active component was observed after dispersion of the perovskite phase: 25 times for BaTiO3/γ-Al2O3 and 30 times for PbTiO3/γ-Al2O3 compared with the unsupported perovskite phases. At the same time, even though a decrease of the apparent activation energy was observed for the supported catalysts, it was insignificant for PbTiO3 perovskite. These observations suggest that dispersion of perovskites on γ-Al2O3 results in an increased exposed surface area of the active component, responsible for the observed increase of the catalytic activity.
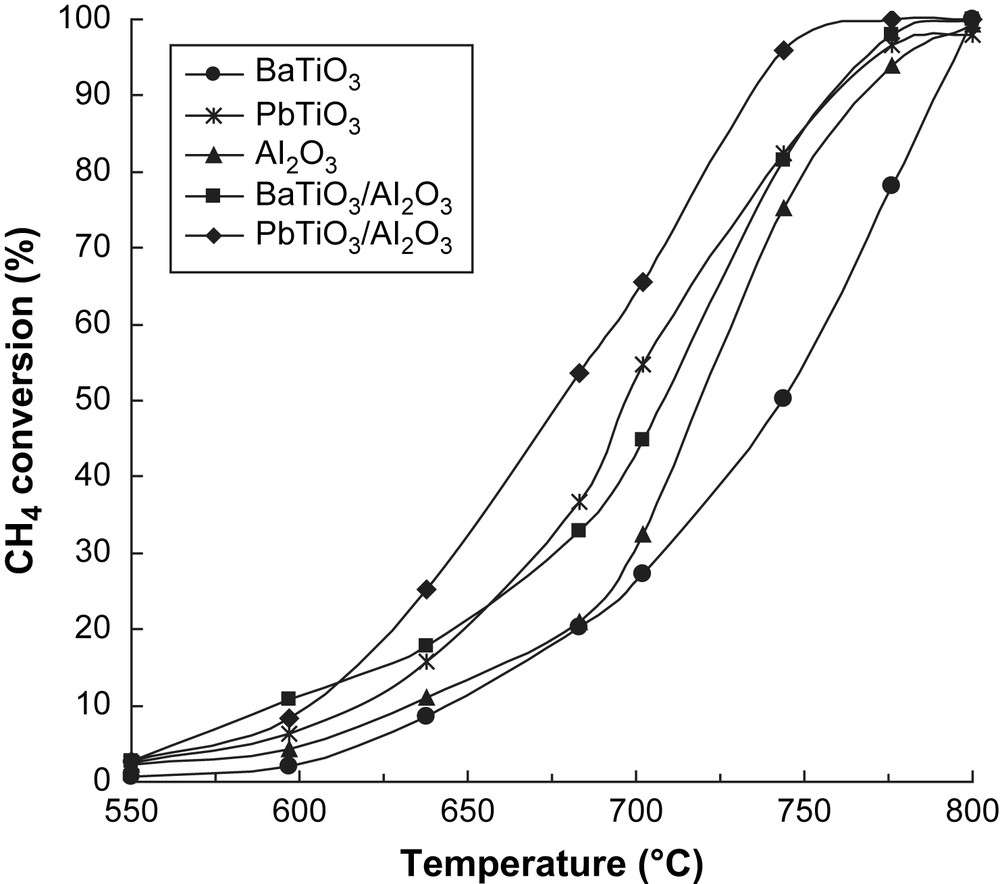
Methane conversion versus reaction temperature for the five catalysts.
Catalytic performances of the catalysts in methane combustion.
Catalyst | T50 (°C) | Ea (kcal/mol) | Reaction rate at 700 °C (106 mol/g s) | Reaction rate over perovskite phase at 700 °C (106 mol/g s) |
γ-Al2O3 | 719 | 29.7 | 5.57 | – |
BaTiO3 | 744 | 38.1 | 1.95 | 1.95 |
BaTiO3/Al2O3 | 708 | 27.4 | 7.69 | 47.78 |
PbTiO3 | 697 | 31.1 | 3.94 | 3.94 |
PbTiO3/Al2O3 | 678 | 29.1 | 11.25 | 118.98 |
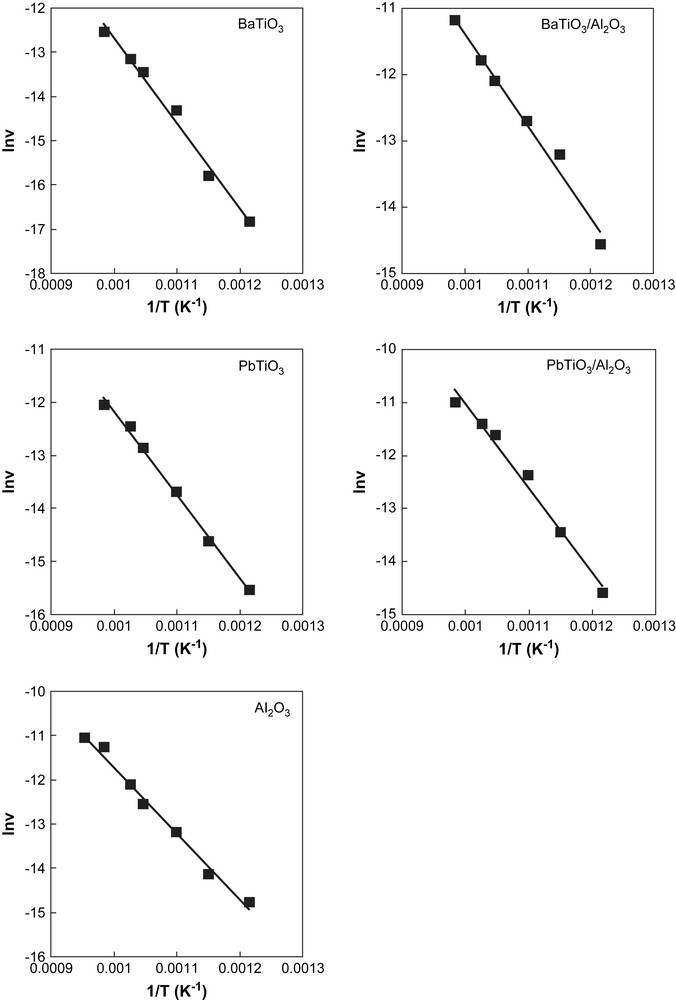
Arrhenius plots for methane combustion on the five catalysts.
We note that the specific activity of the supported perovskite phase (the active component) in the supported catalyst, a, was calculated from the difference between the overall specific activity of the supported catalyst, a1, and the specific activity of alumina support, a0, as follows:
a = (a1 − 0.95a0)/0.05 |
4 Conclusion
BaTiO3 and PbTiO3 perovskites have been obtained by means of the solid state reaction method. The catalysts are single perovskite phases with an important surface enrichment in lead for PbTiO3. They exhibit good activity in methane catalytic combustion, BaTiO3 being less active than PbTiO3. Perovskites were supported on γ-Al2O3 by incorporation of perovskite powders in the alumina support during its precipitation. From the structural point of view no formation of new phases has been detected after dispersion. Dispersion of perovskites on γ-Al2O3 results in an increase of the catalytic activity due to the increasing of the exposed surface area.
Acknowledgements
The authors are grateful to Mr. Oravetz Dezső for his expertise and for technical assistance with scanning electron micrographs recording and EDX analysis.