1 Introduction
Today, sol-gel thin films are used for various applications such as optics, energy conversion, electronics or sensing. For many years, CEA/DAM is strongly involved in sol-gel research and applications, and has developed expertise in functional sol-gel coatings. The main application involves addressing optical coatings for lasers, based on the fact that the CEA in 1985 chose to employ the sol-gel process for coating large-sized lenses and windows of its high-power laser facilities. Since then, the CEA's sol-gel laboratory has developed novel sol-gel coatings and has extended their scope to membrane materials and coatings for energy conversion, electric coatings for microelectronic devices and thin films for gas sensing. The present paper describes, by way of examples, the research performed on sol-gel functional coatings, which spans over nanomaterial synthesis, organic-inorganic hybrid-based solution preparation as well as deposition process development and prototyping.
2 Sol-gel synthesis for optical coatings: antireflective and mirror coatings
Just like the National Laser Facilities in the US, developed and operated in the past and present by LLNL [1,2], the CEA/DAM megajoule-class pulsed Nd:glass laser devoted to Inertial Confinement Fusion (ICF) research requires thousands of square meters of optical coated area deposited onto 10,000 large-sized glass components. Thanks to the well-known advantages of the sol-gel route (i.e., high-performance, room-temperature and low-cost process), this smooth chemistry has been selected at an early stage to prepare and develop tailor-made materials and coatings for laser optics. Thus, since 1985, CEA's sol-gel laboratory is involved in research and development of sol-gel antireflective (AR) and highly-reflective (HR) laser-damage-resistant coatings, for current and future high power laser systems [3].
In addition to the high optical transparency of sol-gel materials used in such thin films, the preparation of antireflective and mirror high-power laser coatings needs to fulfill two extra requirements. As mentioned by several authors [4,5] the first requirement is that the coatings should endure high laser peak fluences. This could only be achieved using dielectric-based materials such as silicon and other metallic oxides. Since the architecture of the future megajoule-class laser driver (LMJ) in France or the National Ignition Facility (NIF) in the US [6] includes large-area square glass substrates, another requirement to fulfill is the development of an associated scaleable deposition technique enabling a highly homogeneous and reproducible deposition onto substrates that are 44 cm in length [7,8,9]. For this purpose, a specific liquid deposition method, so-called meniscus-coating, has been developed and qualified at the CEA.
Oxide-based materials are the most widely investigated for dielectric layers used in optics and, therefore represent the best opportunity for the promotion of the sol-gel chemistry in an applied research field [10]. Two kinds of sol-gel solutions can be chosen for the preparation of sol-gel coatings: polymeric or colloidal (Fig. 1). Both these coating solutions involve using the hydrolysis and the condensation of metallic precursors (salts or alkoxides): the inorganic polymerization reaction (nucleation and growth) is controlled by varying the chemical conditions (pH, hydrolysis ratio, solid content…) and renders it possible to prepare colloidal particles (1 nm to a few hundreds of nm in size) or polymeric species dispersed in a liquid medium depending on the catalyst used (respectively basic or acid) [11]. The colloidal route is convenient for preparing unstressed thick films or multilayer coatings, thus preventing crazing and peeling phenomena. Moreover, it generally produces highly laser-damage-resistant optical coatings due to an elevated purity control, but their abrasion resistance after room-deposition is often poor. Depending of the coating use, the colloids may be combined with binders, fillers or coupling agents in order to improve mechanical properties [12]. With the polymeric route, a densification step (UV irradiation or thermal curing) is necessary to finally obtain a dense layer that generally exhibits a good abrasion resistance and a high refractive index [13]. Because of the important internal stress induced by the shrinkage during the densification step, the polymeric materials are usually not utilized for preparing thick films or multilayer stacks [14].
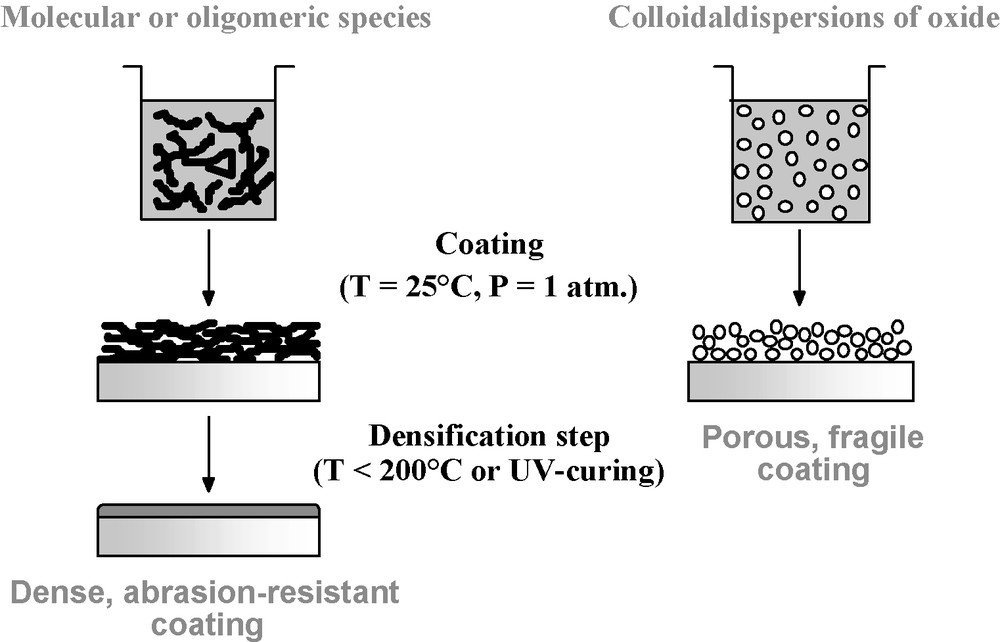
The sol-gel coating solution process: polymeric (left) and colloidal (right) routes.
There are basically three types of antireflective coatings for minimizing specular reflection of incident light: the simplest corresponds to a single-layer coating in which the refractive index is ideally equal to the square root of that of the substrate, assuming that air is the external medium. The other two types are multilayer stacks (two or more layers of different refractive index) and graded-index systems where the index is uniformly and continuously graded from the substrate to the external medium [15]. Among these three, two kinds of antireflective coatings have been specifically developed for high-power lasers, based either on single-layer, or on multilayer designs (not detailed here). The single-layer coating comprises nano-sized spherical particles (10 nm average diameters) of amorphous silica deposited from a colloidal suspension (Fig. 2).
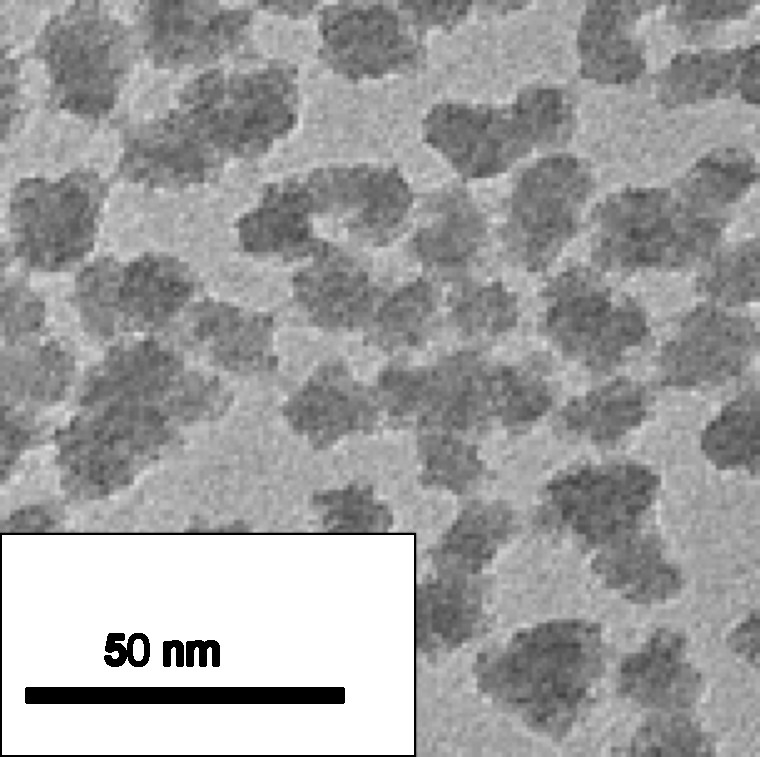
Colloidal nanosilica synthesized using a base-catalysis route.
The silica sol preparation, derived from the Stöber silica synthesis [16], involves base-catalyzed (ammonium hydroxide) hydrolysis of tetrahydroxysilane in ethanol with a Si/H2O molar ratio of 1/2.2 at room temperature. In basic conditions, hydrolysis followed by formation of insoluble silica colloidal particles is favored over condensation of soluble polymers. After deposition and solvent evaporation, the silica nanoparticles do not pack perfectly together and thus give rise to a porous film (∼ 57% porosity). Air present within the space between particles renders it possible to decrease the refractive index of the coating to a value of 1.22. Deposited onto both sides of a silica substrate (refractive index: 1.45), this standard single-layer antireflective (AR) coating enables to reach a transmission value of practically 100% at a single wavelength, which can be shifted by varying the thickness of the layer. The high purity of such nanosized silica coatings contributes to a UV cut-off as low as 200 nm and to a very high laser damage threshold (> 50 J.cm−2 @ 1,053 nm wavelength, R/1 mode and 3 ns pulse duration). The scratch-resistance of a single-layer antireflective coating is poor since no chemical bonds exist between independent silica particles and the substrate surface. A treatment of the deposited porous silica coating with ammonia vapors during a few hours at room-temperature gives rise to a considerable strengthening of the coating without loss of optical properties. Ammonia-curing corresponds to a solid-state ambient sintering with vicinal silica particle bonding induced by a vapor-phase basic catalysis [17]. This causes the coating thickness to shrink slightly (∼ 10–20% of the original thickness), but the antireflective function is retained which means that the porosity and consequently the refractive index remain nearly unchanged. The ammonia-hardening post-treatment renders it possible to alcohol drag-wipe and to physically touch and handle the single-layer AR-coated glass substrates. The scratch-resistance of colloidal silica-based thin films can thus be improved [18] and many authors have consecutively reported on the use of such ammonia-curing on silica coatings [19,20,21,22].
Recent studies have led to a dramatic improvement of the scratch resistance of coatings by using an aqueous silica sol instead of an alcoholic one [23]. Indeed, such an aqueous synthesis route was found to create a water layer physically adsorbed around the silica nanoparticles, which definitely impacted their surface reactivity. All characterizations (NMR, thermal analysis, PM-IRRAS) performed on the colloidal water-based thin films when exposed to catalytic curing (ammonia-hardening) have shown that a particle-to-particle surface base-catalyzed condensation occurs, comparable to that of room-temperature surface sintering. The interparticle bond formation includes solid-state siloxane bridging and hydrogen bonding. The scratch resistance of the film could thus be tremendously upgraded while decent optical properties were maintained, e.g., a high transparency (99.8% transmission) and a low refractive index (1.25).
This improvement is now routinely used for laser antireflective sol-gel coatings since it enables a more convenient handling and an increased lifetime of coated parts. If required, an easy removal of the coating using a soft chemical scrubbing of the surface is still possible without damaging the optical surface finish.
Mirrors and reflectors used in high-power lasers are exclusively made of transparent dielectric materials stacked alternatively as high-index and low-index layers [24]. The reflection factor is a function of the index ratio as well as of the number of layers. Each layer is arranged so that its optical thickness is equal to one-quarter of the wavelength at which maximum reflection is required. In the case of mirrored coating preparation, the use of sol-gel colloidal suspensions of oxides is in fact a real advantage since a simple chemical wiping of coating materials allows a facile substrate recycling and suppresses the tricky and onerous polishing step required in the case of conventionally deposited films.
The colloidal silica used in antireflective coatings plays the role of the low refractive index material in a reflective stack. For the high index material, a specific hybrid system has been developed [25]. It contains metal oxide particles, such as zirconia, embedded in a polymeric binder, e.g., polyvinylpyrrolidone hereafter referred to as PVP. The sol was prepared by urea neutralization of zirconium oxychloride in aqueous medium followed by hydrothermal crystallization similar to the method described by Somiya et al. [26]. The white precipitate was carefully washed, suspended in water by the addition of hydrochloric acid and concentrated before the addition of pure methanol. The organic polymer methanolic solution was introduced into the oxide sol and due to the amphiphilic character of the polymer. Its function was to help maintain colloidal stability and reduce aggregation through a steric stabilization mechanism [27]. By employing such a hybrid system with a polymeric binder in the high-index oxide sol, it was possible to decrease the total number of layers required for the same reflection value.
This work has highlighted the adjustable behavior of nanocomposite solutions with respect to targeted optical properties [28]. Indeed, by tuning the composition of the solution, in particular the polymer/oxide ratio, one can prepare a time-stable solution that gives rise to thin films with a specific refractive index. This way, all refractive index values are available, on demand, in the range of two extrema (Fig. 3). It has been shown that the index variation curve is separated into two zones. In the filling zone, the binder (polymer) concentration progressively increases, filling up the coating porosity and consequently raising the refractive index. In the dilution zone, further addition of binder results in a larger interparticle distance, decreases the packing density of the coating and therefore lowers the refractive index value which tends toward that of the pure polymer. This typical index behavior has been demonstrated for several polymer/oxide systems and is in good agreement with a simple model taking into account a quadratic mixture law of refractive indexes [29]. A large number of labs have followed and adapted such a hybrid organic/inorganic concept for optical coatings [30–33].

The refractive index variation versus the nanocomposite molar composition.
3 Sol-gel materials for energy (PEMFC, solar cells)
During the past few years, a tremendous effort for developing hierarchically structured materials has been observed. Indeed, hierarchical structured hybrid organic-inorganic materials render it possible to accommodate a maximum of elementary functions in a small volume, thereby optimizing complementary possibilities and properties between inorganic and organic components [34]. The usual reported strategies combine sol-gel chemistry, self-assembly routes using templates which tune the pore size, shape and material architecture, with the use of larger inorganic templates, organic templates or biotemplates. As part of this material family, mesostructured materials are traditionally prepared by evaporation-induced self-assembly (EISA) of inorganic or/and hybrid precursors in the presence of surfactant, water, solvent and catalysts [35–37]. In addition to these standard routes [38], an innovative approach to transparent hierarchical hybrid functionalized membranes has been introduced and involves in situ generation of mesostructured hybrid phases inside a non-porogenic polymeric host-matrix. EISA in a non-porogenic hydrophobic polymeric medium is a novel concept allowing to synthesize new hybrid materials characterized by hierarchical structures with macro- and mesoscopic domains. Following this newly proposed route, a large variety of macro-organizations can be obtained under one-pot synthesis conditions, with specific mesostructures, depending on the surfactant/polymer/solvent affinities [39]. It has been demonstrated that the control of the multiple affinities existing between organic and inorganic-components renders it possible to manage the nanobuilding and the length scale partitioning of hybrid nanomaterials with tuned functionalities and desirable size organization from angstrom to centimeters. Thus, the introduction of hydrophobic and solvophobic organic polymers as additional compounds in the one-pot surfactant templated synthesis of meso-organized organo-silicas induces extra hierarchical levels. Moreover, in situ growth of the inorganic or hybrid phase in a polymeric network avoids conventional removed-solvent media and allows a direct shaping of the material.
Following this preparation concept, highly transparent membranes containing mesostructured and homogeneously dispersed inorganic solids can be obtained (Fig. 4). In this case, during the solvent removal and the induced hierarchical domain sizing, the final structure and morphology is managed by affinity between the following keys: inorganic or/and hybrid precursors, surfactant and polymer host-matrix. This way, among all intrinsic compound properties, as-prepared materials offer a well-defined mesostructured inorganic or hybrid network that is homogeneously dispersed as submicronic spheres in the dense transparent polymeric matrix. During solvent evaporation, the mother solution is concentrated, which allows the non-volatile species and surfactant-free molecules to segregate in well-defined nanodomains such as micelles, ideally self-assembled into mono-, bi- or tridimensional mesophases while the inorganic polymerization occurs at the micelle-water interface. The partial solvent exchange with a polymeric solution renders it possible to generate favorable interfacial phenomena between organic and inorganic molecules.
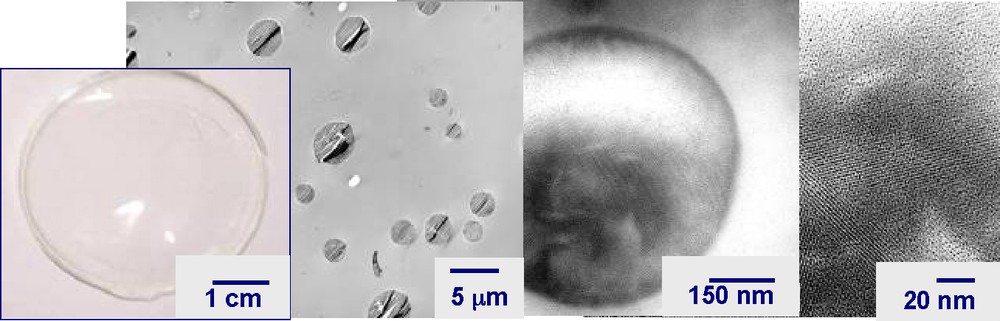
Multiscale photos (full-size and TEM images) of a transparent membrane prepared by EISA in a non-porogenic polymeric matrix, highlighting the silica sphere mesostructure.
This concept has also been applied to the preparation of fuel cell proton exchange membranes [40]. After functionalization of the mesoporous hybrid silica component, the obtained as-prepared membranes exhibited a decent ionic conductivity offering interesting perspectives for the design of solid electrolytes, fuel cells and other ion transport microdevices [41]. It has been highlighted that an elevated porosity of such PVDF hybrid membranes containing large amounts of mesoporous silica (up to 35% wt) is very interesting for water-uptake properties and ionic conduction.
This work demonstrates the important role of the various affinities and the competition between all chemical actors in which the in situ created hybrid interfaces are predominant. Indeed, it can be concluded that the better the molecular compatibility at the silica/polymer, silica/surfactant or polymer/surfactant interface via weak (hydrogen, electrostatic or Van der Waals) or strong (covalent or ionic) chemical bonds, the better are the homogeneity and hierarchical organization of the hybrid materials. Moreover, the liquid-based medium renders it possible to process these materials with various shapes, e.g., spin- or dip-coated thin films, membranes or cast-shaping of monoliths inside moulds.
To the best of our knowledge, multiscaled hybrid materials have yet to be prepared in a one-pot synthesis approach and the present article proposes the successful in situ hybrid organic-inorganic homogeneous growth in one-step synthesis in a multicompound medium only when suitable chemical couples are selected, each of them respecting the hydrophilic-hydrophobic balance. This way, one can reach tunable and nanoscale homogenous compositions, various functionalities as well as embedded nanostructured morphologies.
Alternative energy sources, such as solar and wind power, have received increasing attention over the past decades in order to replace the environmentally damaging and diminishing fossil fuels. Solar power is obviously one of the most attractive renewable energy sources. Up to now, the main photovoltaic (PV) devices have been based on solid-state junctions, usually made of silicon, and take advantage of the development of the semiconductor industry. Presently, a challenging new generation of solar cells is emerging based on interpenetrating networks built from nanocrystalline sensitized oxides and conducting electrolytes [42]. The so-called dye-sensitized solar cells (DSSCs), based on a liquid electrolyte associated with a redox couple, are easy to fabricate and lead to low-cost devices. Therefore, DSSCs constitute a promising alternative to silicon-based p–n junction PV devices. Solar cells containing TiO2 as a semiconductor, ruthenium-based complexes as a dye, and an iodide/iodine redox couple in acetonitrile as an electrolyte, present excellent solar energy conversion performances (> 10%) [43]. Despite that Grätzel's cells are now commercially available, market expansion is limited because of the use of a liquid electrolyte. Indeed, technological fabrication issues of cell sealing, handling, and maintenance, and the difficulty of marketing flexible PV cells with highly corrosive liquid electrolytes, are still a concern. A large number of alternative solutions have been proposed. These include replacing the liquid redox electrolyte with, for instance, molecular or macromolecular organic hole conductors such as triphenyldiamine-, polypyrrole-[44], and p-phenylene vinylene-based copolymers [45] or substituted polythiophenes [46].
This new generation of all-solid-state PV cells is full of potential and highly attractive. Nevertheless, these promising devices still exhibit poor long-term stabilities. A recent report [47] describes a study on the sol–gel elaboration of homogeneous nanoporous TiO2 films in which control of the architecture, porosity, and layer thickness rendered it possible to optimize the solid solar-cell efficiency and stability. A very effective design and processing of all-solid-state solar cells involved the use of high-quality TiO2-based mesoporous films as n-type semiconductor layers, a Ru-based complex (Ru-dye) for visible-light absorption and regioregular poly(3-octylthiophene) (P3OT) as a hole conductor (Fig. 5). A high current-density value and unexpected solar-conversion efficiency (up to 3.90 mAcm–2 and 1.3%, respectively) could be achieved, as well as a very robust device at a low cost. More than 60 cells were prepared and tested at the same time and reproducible results were obtained [48]. Consequently, the industrial production of such solar cells has true potential. These reported results for all-solid-state dye-sensitized nanoporous TiO2 hybrid solar cells demonstrating high energy conversions are pushing forward the development and the fabrication of a challenging new generation of solar cells.
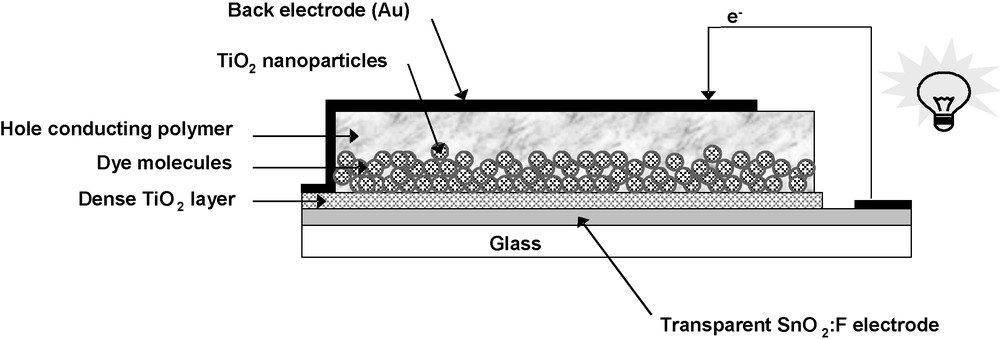
Layer structure of the all-solid-state dye-sensitized hybrid solar cell.
4 Microelectronics devices made from a ceramic sol-gel process: high permittivity and piezoelectric applications
The sol-gel chemistry has also been successfully used to deposit high permittivity thin film capacitor – based on lead-zirconate-titanate (PbZrxTi1–xO3, PZT) – ferroelectric ceramic layers onto 6” platinized silicon wafers through a spin-coating technique. Ferroelectric ceramics with a perovskite structure present much higher permittivity values than any other material [49]. Among ferroelectrics, PZT is one of the most widely studied for applications in electro-optic devices, non-volatile memories, pyroelectric sensors, MEMS and capacitors. Since many years, PZT films have been processed onto silicon wafers through the sol-gel method [50]. The advantages of the sol-gel method as compared to other deposition techniques are well-known: lower processing temperature, homogeneous and high purity materials with controlled stoichiometries, ultimately leading to a low-cost process. Particularly, the influence of experimental parameters on film properties have been studied for a long time: precursor, solvent, complexing agent, heat treatment, composition, lead excess, substrate nature, film thickness [51,52]. However, few investigations have concerned the reliability and reproducibility of the sol-gel process for the preparation of high-performance ferroelectric thin films. Accordingly, chip-makers hesitate to switch to such a liquid deposition process, particularly because of the short solution time stability and non-reproducibility with regard to film deposition which are identified as the main drawbacks.
The purpose of the present work has been to demonstrate the ability of a diol-based sol-gel process for preparing moisture-insensitive, highly stable, long-term shelf-life solutions leading to repeatable coating performance [53]. With the aim of obtaining reproducible high-capacitance PZT thin films, a sol-gel diolbased PZT solution has been specially developed by diluting the high viscosity primary solution with ethylene glycol after a required ripening time. The careful control of each step of the sol synthesis has allowed the preparation of PZT solutions displaying reproducible chemical and physical properties. The deposition process has been optimized to reduce the number of layers as well as the drying time during spinning. By introducing a low-temperature treatment (prepyrolysis at 50 °C) before the pyrolysis (at 360 °C), crack-free 0.2-μm thick coatings can be produced in just a 6-min step per wafer run. Thin films prepared using this reproducible and repeatable sol-gel process exhibit constant physical thicknesses and therefore constant permittivity values, regardless of the age of the solution (up to 1 year pot life). Thus, 200 nm thick PZT layers with permittivity values as high as 900, capacitances as high as 40 nF/mm2 and breakdown voltages up to 30 V have been obtained (Fig. 6).
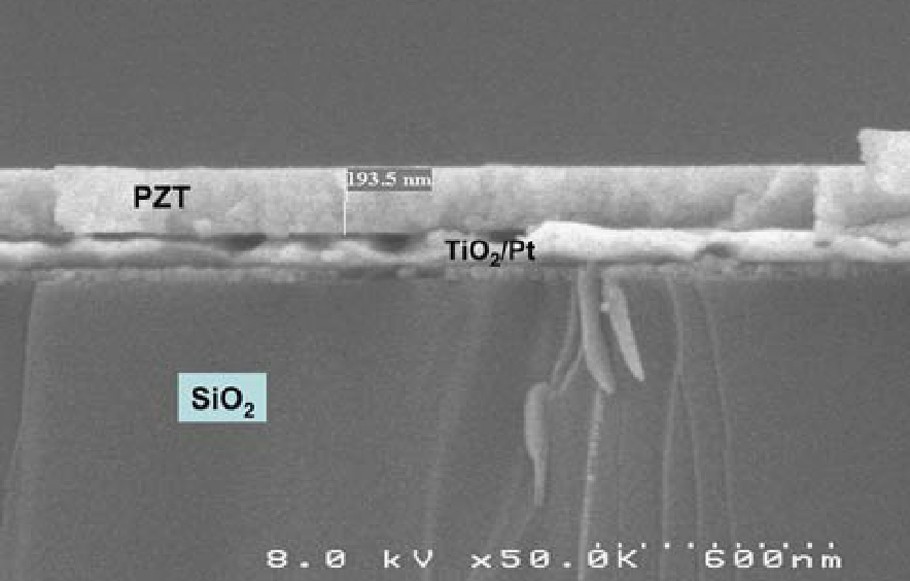
A SEM micrograph of a dense PZT sol-gel « high k » thin film.
In further work [54], piezoelectric PbZr0.52Ti0.48O3 (PZT) thick films were deposited onto silicon or alumina substrates using a specially developed sol-gel nanocomposite slurry. The nanocomposite sol-gel process consisted in mixing a PZT sol with PZT powders (rough or attrited). Such a mixture gave rise to thicker films without cracks. The already-developed time-stable polymeric PZT sol was used twice, as the binder and as a precursor for PZT powder fabrication. Unfortunately, up to now, relatively low piezoelectric properties, as compared to other techniques (i.e., screen-printing, pad-printing or inkjet-printing), have been reported in the literature with the sol-gel method [55]. Nonetheless, the method has numerous advantages for such applications: low-cost equipment, low process temperature, control of stoichiometry and film thickness. Furthermore, the sol-gel process is perfectly appropriate for deposition onto substrates with complex shapes. This is why we have proceeded with the nanocomposite route in order to optimize the sol composition and to improve film microstructure. By focusing on high frequency ultrasonic transducer applications, it was successfully demonstrated that high-performance piezoelectric thick films for high-frequency ultrasonic transducers could be prepared by way of different powder preparation processes. However, in the case of attrited powder, more homogeneous and reproducible thick films were obtained (Fig. 7). The narrower pore size distribution allowed a better control of the electromechanical properties and although the film was clamped to a substrate and could not behave as a free resonator, effective coupling factor values up to 49% were measured. These results were obtained by tuning, for each type of powder, the sol concentration, the powder content, the number of infiltrations and the heat treatment parameters. The liquid deposition technique has allowed the elaboration of transducers on curved substrates. This is a real advance for high resolution imaging since it renders it possible to focus the acoustic waves of an ultrasonic transducer in a biological medium without any acoustic lens being required. New medical applications in microsurgery, ophthalmology or dermatology have recently boosted the interest for high-resolution echographic imaging in the 30–50 MHz frequency range. Consequently, it leads the way toward new prospects for such nanocomposite coatings, which hold, as far as is known today, the best effective coupling factor value ever reported with the sol-gel proces.
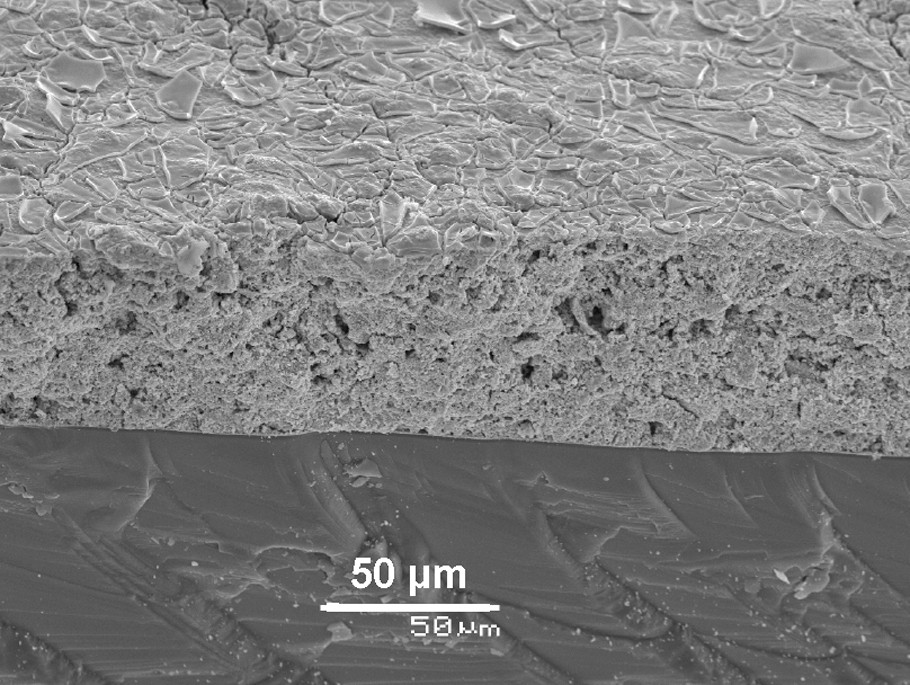
A SEM micrograph of a multilayer piezoelectric nanocomposite PZT thick film.
5 Gas sensors using sol-gel thin films
With the increased use of explosives such as nitroaromatics in terrorist attacks, the development of efficient, portable and low-cost explosive detection devices has become an urgent worldwide necessity. The last 10 years, numerous studies have been performed on the detection of nitroaromatic compounds using chemical gas sensors as highly sensitive and selective detectors of various explosives [56]. Silica-based materials have never been used as chemical sensors for humidity or NO detection [57]. In a recent investigation [58], a new promising material based on a nanostructured high-porosity silica coating has been developed and tested regarding chemical sensing of nitroaromatics. Silica nanoporous coatings exhibit a large sensitivity and selectivity towards nitroaromatics when used as quartz crystal microbalances or fluorescent sensors if grafted with a fluorescent probe. The hydroxyl surface coverage of nano-sized silica particles can be tailored depending on the solvent selected for peptization (water or ethanol) or using surface functionalization induced by further exposition to catalytic vapor-phase curing (ammonia-hardening or hydrophobic grafting). The type of particle surface species coverage was highlighted to directly impact the structural properties of the films and therefore the detection performance. Among the various silica coatings prepared, a fluorescent film has been selected, showing a high sensibility (at 0.1 ppm and at 1 ppm), an excellent reversibility (80% of fluorescent intensity), robustness and good selectivity regarding interfering V.O.C.s (Volatile Organic Compounds). Moreover, a specifically developed hydrophobic silica thin film has shown good sensitivity regardless of the humidity during operation (from 0 to 80%RH)s (Fig. 8).
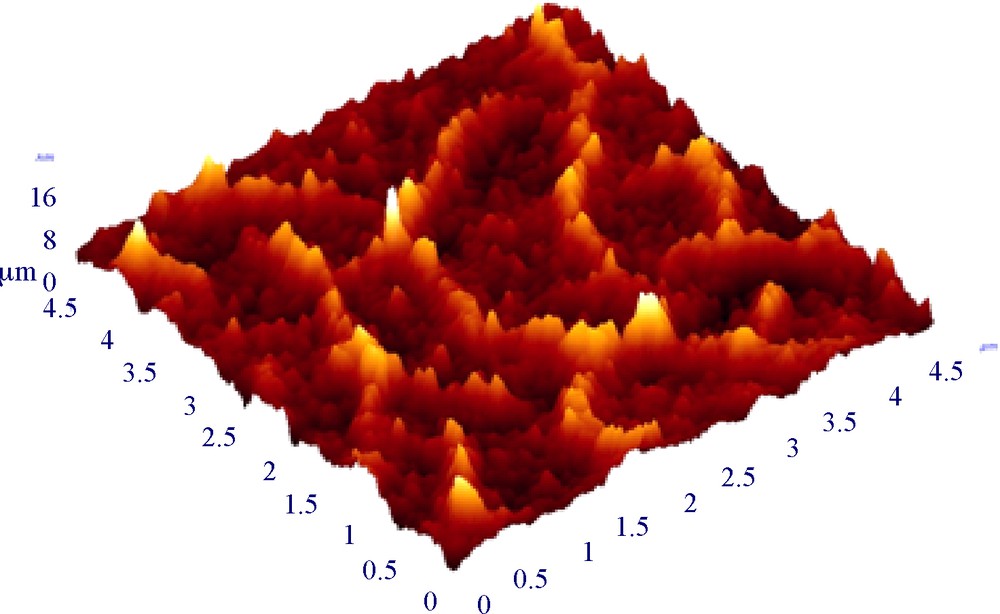
An AFM view of a nanostructured silica coating developed for nitroaromatic sensors. (image scale : 5 μm (x) × 5 μm (y) × 10 nm (z)).
6 Conclusions
The present article is an attempt to illustrate, though examples, some of the research topics in functional hybrid organic-inorganic and ceramic-based materials. During the past decade, certain hybrid materials appeared either as prototypes, or commercial products. However, these only represent the tip of the iceberg. It is our belief that advanced hybrid materials will play a major role in the future and apart from the high level of knowledge and understanding of scientists regarding nanochemistry, another step needs to be passed: making industries aware of these opportunities and benefits. In this context, the objective is to succeed, as far as possible, in the development of not only functional materials but also reliable prototypes, devices and products.