1 Introduction
Insertion reactions constitute one of the classic types of organometallic reactions that define the reactivity of metal carbon bonds [1]. The insertion reactivity of the U-C bond has been extensively studied with (C5Me5)2UMe2 [2] and this has resulted in a wealth of actinide complexes with interesting structural features, coordination environments, and reactivity (e.g. Scheme 1) [3–7].

Insertion reactivity of (C5Me5)2UMe2.
Organoactinide insertion chemistry has also been studied with (C5Me5)2U(CCPh)2 [8]. This complex has been frequently used as an in situ initiator for catalytic processes that involve insertion reactions such as the coupling of nitriles, isocyanides, and terminal alkynes, oligomerization of terminal alkynes, and the hydrosilylation of terminal alkynes [9].
Recently, an analog of (C5Me5)2UMe2 was synthesized, namely (η5:κ1-C5Me4SiMe2CH2)2U, 1, that made it possible to examine U-C bond reactivity of a tethered alkyl [10]. Complex 1 is generated from (C5Me4SiMe3)2UMe2 by metalation of each of the (C5Me4SiMe3)1− ligands, Eq. (1) [10]. The U-C bonds in 1 are in a coordination environment similar tothat of (C5Me5)2UMe2, but variations in reactivity may be observable since the reactive alkyls are tethered to the ancillary cyclopentadienyl ligand [11]. Preliminary studies with carbon monoxide and tert-butyl isocyanide demonstrated that 1 provides both structural data and reactivity modes that are not accessible from (C5Me5)2UMe2 [10]. With these promising results, we continued our study of this tethered system and report here on the reactivity of 1 with tert-butyl nitrile, carbon dioxide, phenylacetylene, and diphenylacetylene.
2 Experimental
The syntheses and manipulations described below were conducted under nitrogen or argon with rigorous exclusion of air and water using glovebox, Schlenk, and vacuum-line techniques. All reactions were performed at room temperature unless otherwise noted. Solvents were dried over columns containing Q-5 and 4A molecular sieves. Benzene-d6 was dried over NaK alloy, degassed using three freeze-pump-thaw cycles, and vacuum transferred before use. Tert-butyl nitrile and PhCCH were dried over 4A molecular sieves and degassed using three freeze-pump-thaw cycles before use. PhCCPh was purchased from Aldrich and used as received. Ultrahigh purity carbon dioxide was purchased from Airgas and used as received. (η5:κ1-C5Me4SiMe2CH2)2U, 1, was prepared as previously reported [10]. 1H NMR spectra were recorded with a Bruker GN 500 MHz spectrometer and 13C NMR spectra were recorded with a Bruker CRYO 500 MHz spectrometer. Infrared spectra were recorded as KBr pellets on a Varian 1000 FT-IR spectrometer. Elemental analysis was performed on a Perkin Elmer 2400 Series II CHN analyzer.
[η5:κ1-C5Me4SiMe2CH2C(tBu)N]2U, 2. In a glovebox, a red solution of 1 (230 mg, 0.37 mmol) in toluene (10 mL) was added to a stirred solution of tBuCN (61 mg, 0.74 mmol) in toluene (5 mL). After 12 h, solvent was removed under vacuum from the red solution to yield 2 as a dark red microcrystalline solid (280 mg, 97%). X-ray quality crystals of 2 were grown from a concentrated hexane solution at −35 °C. 1H NMR (C6D6): δ −36.3 (s, C5Me4SiMe2CH2C(tBu)N, 6H), −24.7 (s, C5Me4SiMe2CH2C(tBu)N, 6H), −6.56 (s, C5Me4SiMe2CH2C(tBu)N, 6H), −4.95 (s, C5Me4SiMe2CH2C(tBu)N, 6H), −2.45 (s, C5Me4SiMe2CH2C(tBu)N, 18H), 7.61 (s, C5Me4SiMe2CH2C(tBu)N, 6H), 20.3 (s, C5Me4SiMe2CH2C(tBu)N, 6H), 32.0 (d, C5Me4SiMe2CH2C(tBu)N, 2H, 2JHH = 11.4 Hz), 32.4 (d, C5Me4SiMe2CH2C(tBu)N, 2H, 2JHH = 11.4 Hz). 13C NMR (125.5 MHz, C6D6): δ −114 (C5Me4SiMe2CH2C(tBu)N), −89.1 (C5Me4SiMe2CH2C(tBu)N), −83.8 (C5Me4SiMe2CH2C(tBu)N), −79.9 (C5Me4SiMe2CH2C(tBu)N), −51.3 (C5Me4SiMe2CH2C(tBu)N), −18.9 (C5Me4SiMe2CH2C(tBu)N), 18.4 (C5Me4SiMe2CH2C(tBu)N), 33.5 (C5Me4SiMe2CH2C(tBu)N). IR: 2958 s, 2901s, 1605s, 1457m, 1383m, 1326m, 1247s, 1160m, 1046m, 1020m, 991m, 833s, 666m cm−1. Anal. Calcd for C34H58Si2N2U: C, 51.75; H, 7.41; N, 3.55. Found: C, 51.22; H, 7.87; N, 3.08.
(C5Me4SiMe2CH2CO2)2U, 3. A red solution of 1 (320 mg, 0.51 mmol) in benzene (25 mL) in a 100 mL Schlenk flask was attached to a high vacuum line and degassed by three freeze-pump-thaw cycles. One atmosphere of CO2 was introduced to the reaction flask, the solution was stirred for 15 min, and the solution became yellow-brown. The reaction was degassed by one freeze-pump-thaw cycle, brought into a glovebox, and the solvent was removed under vacuum to yield 3 as a gold powder (350 mg, 96%). 1H NMR (C6D6): δ −10.0 (s, C5Me4SiMe2CH2CO2, 6H), −5.72 (s, C5Me4SiMe2CH2CO2, 6H), −5.58 (s, C5Me4SiMe2CH2CO2, 2H), −3.46 (s, C5Me4SiMe2CH2CO2, 6H), −3.10 (s, C5Me4SiMe2CH2CO2, 6H), 0.63 (s, C5Me4SiMe2CH2CO2, 6H), 14.7 (s, C5Me4SiMe2CH2CO2, 2H), 15.6 (s, C5Me4SiMe2CH2CO2, 6H). 13C NMR (125.5 MHz, C6D6): δ −40.5 (C5Me4SiMe2CH2CO2), −13.8 (C5Me4SiMe2CH2CO2), −1.69 (C5Me4SiMe2CH2CO2), −0.616 (C5Me4SiMe2CH2CO2), 3.30 (C5Me4SiMe2CH2CO2), 16.5 (C5Me4SiMe2CH2CO2), 60.9 (C5Me4SiMe2CH2CO2). IR: 2955m, 2914m, 1562s, 1492s, 1443m, 1329m, 1284m, 1046m, 966m, 729w, 834m cm−1. Anal. Calcd for C26H40Si2O4U: C, 43.93; H, 5.67. Found: C, 44.20; H, 5.61.
(C5Me4SiMe3)2U(CCPh)2, 4. A solution of 1 (240 mg, 0.39 mmol) in toluene (10 mL) was added to a stirred solution of PhCCH (79 mg, 0.77 mmol) in toluene (5 mL). After 12 h, the solvent was removed from the red solution under vacuum to yield 4 as a red powder (303 mg, 95%). X-ray quality crystals of 4 were grown from a concentrated hexane solution at −35 °C. 1H NMR (C6D6): δ −11.1 (d, CCPh, 4H, 3JHH = 11.4 Hz), −4.96 (s, C5Me4SiMe3, 12H), −2.52 (s, C5Me4SiMe3, 18H), −1.72 (t, CCPh, 4H, 3JHH = 11.4 Hz), 3.79 (t, CCPh, 2H, 3JHH = 11.4 Hz), 17.2 (s, C5Me4SiMe3, 12H). 13C NMR (125.5 MHz, C6D6): δ −41.2 (C5Me4SiMe3), 4.09 (C5Me4SiMe3), 4.53 (C5Me4SiMe3), 51.2 (CCPh), 99.1 (CCPh), 127 (CCPh). IR: 3077w, 2962s, 2950s, 2902s, 2730w, 2055s, 1977w, 1875w, 1800w, 1594m, 1570w, 1485m, 1441m, 1381w, 1347w, 1327m, 1242s, 1198m, 1125m, 1070w, 1024m, 988w, 912w, 846s, 781m, 755s, 690m, 633m, 571w, 533m cm−1. Anal. Calcd for C40H52Si2U: C, 58.09; H, 6.34. Found for three separate samples of crystalline material: C, 55.36–56.63; H, 5.83–6.09. The C:H ratio of each sample; 9.2:1, 9.5:1, and 9.3:1 are consistent with that calculated of 9.2:1. Evidently, incomplete combustion occurs with this sample. Similar problems have been observed in the past [12].
Reaction of 1 and PhCCPh. A solution of 1 (52 mg, 0.08 mmol) in toluene (5 mL) was added to a stirred solution of PhCCPh (29 mg, 0.17 mmol) in toluene (3 mL). After 12 h, the solvent was removed from the red solution under vacuum. No reaction was observed as determined by the 1H NMR spectrum of the isolated solid as only resonances for 1 and PhCCPh were observed.
3 Results and discussion
Reactivity of 1 with tBuCN. The insertion of nitriles into U-C bonds to form uranium ketimides is well-known from studies with the amide metallacycle [(Me3Si)2N]2U(CH2SiMe2NSiMe3) [13] and with (C5Me5)2UR2 metallocenes [6,14]. Actinide ketimides have also been postulated as intermediates in hydroamination reactions with alkynes [9a]. With such a well-established body of work on nitrile insertion into terminal U-C bonds for comparison, it was of interest to investigate the reactivity of 1 with nitriles.
Insertion into both of the tethered U-C bonds of 1 occurs with tBuCN to produce [η5:κ1-C5Me4SiMe2CH2C(tBu)N]2U, 2, as a dark red microcrystalline solid in 97% yield, Eq. (2). Complex 2 has been characterized by NMR and IR spectroscopy, elemental analysis, and
X-ray crystallography (Fig. 1). Complex 2 has two tethered ketimide ligands as a result of a 1,2-insertion of tBuCN into both the tethered alkyl bonds of 1. In contrast, the isocyanide tBuNC inserts by a 1,1-insertion into both U-C bonds of 1, as shown in Eq. (3) [10]. Complex 2 isthe first example of an actinide metallocene complex containing tethered ketimide ligands.
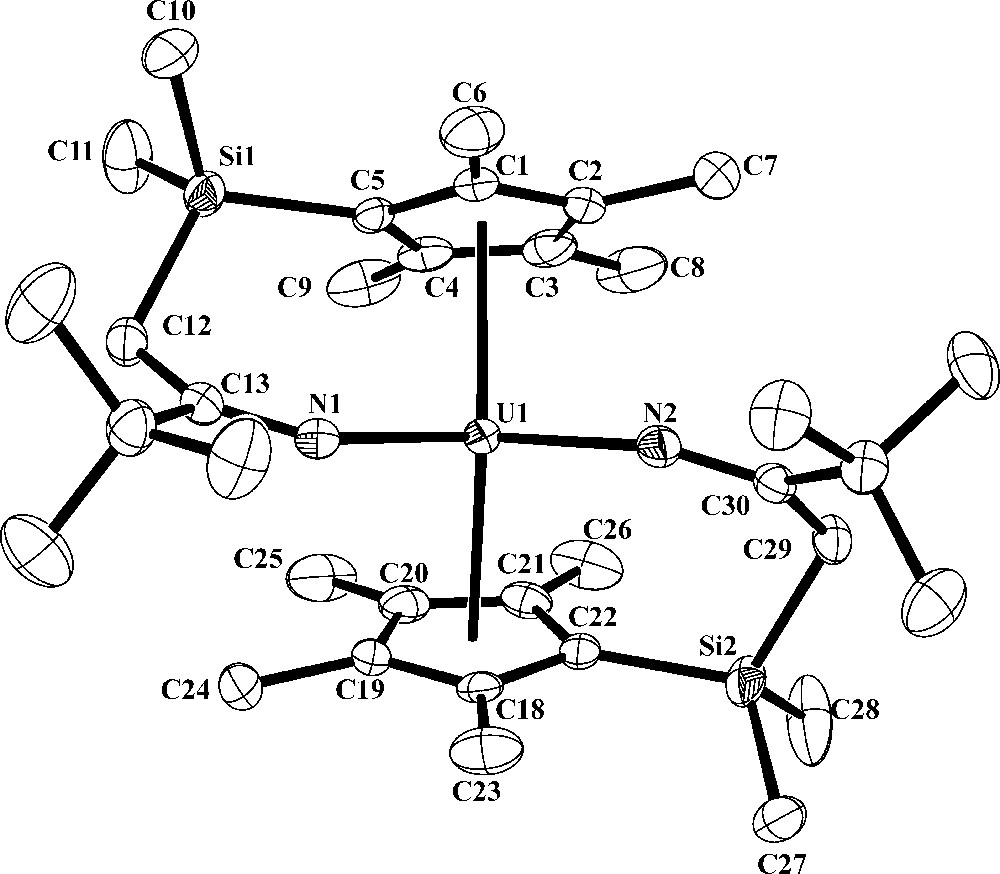
Thermal ellipsoid plot of [η5:κ1-C5Me4SiMe2CH2C(tBu)N]2U, 2, drawn at the 50% probability level. Hydrogen atoms have been omitted for clarity.
The 1H NMR spectrum of 2 contains nine resonances consistent with insertion of one equiv of tBuCN into each of the U-C bonds of 1. The resonances can be assigned to the four unique methyl groups on each cyclopentadienyl ring, two unique methyl groups on each silicon atom, two non-equivalent protons on the methylene groups, and one resonance for the two equivalent tert-butyl groups. Unlike the 1H NMR spectrum of 1, which lacks any resonance assignable to the (C5Me4SiMe2CH2)2− methylene groups bound to the paramagnetic uranium [10], the appearance of resonances for the methylene groups in 2 is consistent with an insertion that moves the methylene groups further from the paramagnetic metal.
The IR spectrum of 2 contained an absorption at 1605 cm−1 attributable to a CN bond stretch that is lower than that of free tBuCN at 2231 cm−1 [15]. This CN absorbance is similar to the 1620 cm−1 absorption observed for the uranium metallacycle [(Me3Si)2N]2U[NC(Me)CH2SiMe2NSiMe3] [13,16], formed from an insertion reaction similar to that shown in Eq. (2).
The metallocene structure of 2 is not unusual, but the uranium ketimide linkages were affected by the tethering. Selected bond distances and angles for 2 are given in Table 2. The 2.473 and 2.482 Å U-(ring centroid) bond distances and the 139.7° (ring centroid)-U-(ring centroid) bond angle are typical of U4+ metallocene complexes with the (C5Me4SiMe3)1− ligand [10]. The 1.264(3) Å N1-C13 and 1.268(3) Å N2-C30 bond distances of the tethered ketimide ligand are similar to those of untethered ketimide ligands [6,14] and are consistent with the IR data that suggested a CN bond. The short U1-N1 and U1-N2 bond distances, both of which are 2.173(2) Å, are also similar to these in other uranium-ketimide complexes [6,14]. In general, U-N ketimide bond distances are typically shorter than those of U-N amide bonds and this has been attributed to π-bonding between uranium and the nitrogen of the ketimide ligand [6,14]. This π-bonding interaction has also been used to explain the near linear U-N-C bond angles present in untethered ketimide ligands [6]. In contrast, the 152.64(2) and 152.47(2)° U-N-C bond angles in 2 deviate significantly from linearity due to the restraints imposed by the tethering. It has previously been shown that short metal-ligand bond distances and linear M-A-B angles are not necessarily correlated [17].
Reactivity of 1 with CO2. Since spectroscopic data on the reaction of (C5Me5)2UMe2 with CO2 to form (C5Me5)2U(O2CMe)2 has been reported [7], it was expected that 1 would also react with this substrate. The reaction of 1 with CO2 (1 atm) formed a complex consistent with the tethered bis(tethered carboxylate), (C5Me4SiMe2CH2CO2)2U, 3, Eq. (4).
Complex 3 was characterized by NMR and IR spectroscopy and by elemental analysis, but like (C5Me5)2U(O2CMe)2 [7], single crystals were not obtained. The spectroscopic data are consistent with CO2 insertion into both U-C bonds of 1 as observed with tBuCN and tBuNC. The 1H NMR spectrum of 3 is similar to that of 2 in that resonances assignable to the (C5Me4SiMe2CH2)2− methylene protons are present. This again indicates that the methylene groups have been moved away from the metal by an insertion reaction. The IR spectrum is also consistent with the insertion of CO2 into the U-C bonds of 1 since an absorption assignable to a carboxylate C-O stretch is present at 1562 cm−1, which is similar to the 1540 cm−1 C-O stretch reported for (C5Me5)2U(O2CMe)2 [7].
Reactivity of 1 with PhCCH. The reaction of 1 with PhCCH was examined to determine whether insertion is possible or if cleavage of the tethered U-C bond would occur to form an alkynide. Straub and co-workers have found that terminal alkynes can display both types of reactivity with uranium metallocenes [9]. The reaction of 1 with two equiv of PhCCH produced (C5Me4SiMe3)2U(CCPh)2, 4, as a red powder in 94% yield, Eq. (5). Complex 4 was characterizedby NMR and IR spectroscopy, elemental analysis and X-ray crystallography (Fig. 2).
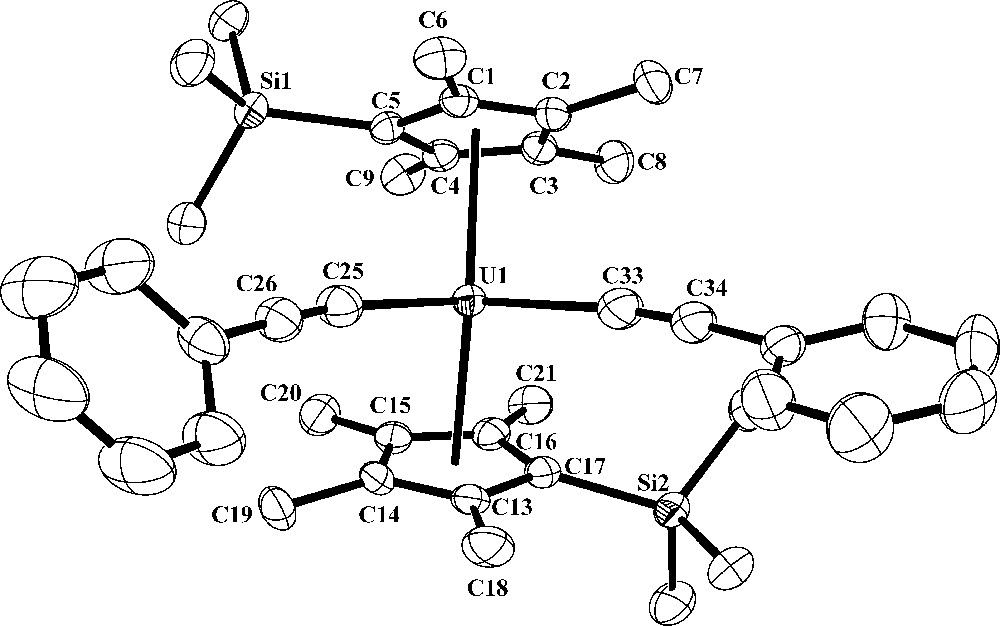
Thermal ellipsoid plot of (C5Me4SiMe3)2U(CCPh)2, 4, drawn at the 50% probability level. Hydrogen atoms have been omitted for clarity.
The cleavage of the tethered U-C bond by PhCCH was evident from the 1H NMR spectrum, which resembled that of (C5Me4SiMe3)2UCl2 [10] rather than the more complicated spectra of 1–3. The IR spectrum of 4 contained a strong absorbance at 2055 cm−1, attributable to the CC bond, which is similar to those previously reported for uranium alkynides such as 2056 cm−1 in (C5Me5)2U(CCPh)2 [8b] and 2062 cm−1 in (C5Me5)2U(NPh2)(CCPh) [18].
X-ray crystallography revealed that the structure of 4 is similar to those of (C5Me4SiMe3)2UCl2 and (C5Me4SiMe3)2UMe2 in terms of the 2.448 and 2.451 Å U1-(ring centroid) bond distances as well as the 143.8° (ring centroid)-U1-(ring centroid) bond angle [10]. As observed in these other complexes, the trimethylsilyl group of 4 is bent away from the metal and therefore does not distort the metallocene structure [10]. The 2.390(2) Å U1-C25 and 2.369(3) Å U1-C33 bond lengths are shorter than the 2.425(2) Å U-C(Me) bond distance of (C5Me4SiMe3)2UMe2 [10], but similar to the U-C bond lengths of 2.398(5) Å in (C5Me5)2U(CCPh)2 [8].
The reaction of 1 with PhCCPh was also investigated to assess the possibility of alkyne insertion into the tethered U-C bonds without the option for sigma bond metathesis of the U-C bonds as occurs with PhCCH. However, no reaction was observed with PhCCPh.
4 Conclusion
Complex 1 provides a platform to study the insertion reactivity of uranium-alkyl bonds in a tethered system. Tert-butyl nitrile undergoes a 1,2-insertion into both tethered U-C bonds of 1 to produce a bis(tethered ketimide), 2, that differs from untethered uranium ketimides in that the U-N-C bond angles are not linear. CO2 also inserts into both U-C bonds to produce a bis(tethered carboxylate). PhCCH does not undergo insertion, but provides a convenient route to cleave the tethered U-C bonds of 1 to form an untethered product with an easily identifiable infrared label.
5 Crystallographic data
CCDC 759467 (2) and CCDC 759466 (4) contain the supplementary crystallographic data for this paper. These data can be obtained free of charge from the Cambridge Crystallographic Data Centre via http://www.ccdc.cam.ac.uk/data_request/cif.
Acknowledgements
We thank the Chemical Sciences, Geosciences, and Biosciences Division of the Office of Basic Energy Sciences of the Department of Energy for support and Michael K. Takase for assistance with the X-ray crystallography.