1 Introduction
Complexes of the early actinides, thorium and uranium, are unique because of their possible distinctive covalency and their f-orbital participation in bonding [1]. The high Lewis acidity of these actinides together with a variety of accessible oxidation states, e.g. U(III)-U(VI), provides unusual reactivities that are complementary in some cases to that observed for other metals in the periodic table [2]. Bonding in f-element complexes has generally been considered to be ionic, however the isolobal comparison between anionic and covalent complexes remains a challenge [3]. Knowing the impact of the oxidation state on the stability of the organoactinide complexes and on the bonding nature allows the design of new types of materials exhibiting tailored reactivities, which are mainly dictated by their coordination spheres [4]. Hence, the stoichiometric insertion of CO [5], CO2 [6], isocyanides [7], ketones [8], diazoalkanes [9], nitriles [7,10], carbodiimide and organic azides [11] into AnC bonds (An=U, Th) has been explored. With regard to catalytic processes, neutral organoactinide complexes have been extensively studied in the past decade for several organic transformations [12]. The polymerization of alkenes [13], the oligomerization of alkynes [14], the inter/intramolecular hydroamination of alkynes [15], the hydrosilylation of alkene and alkynes [16], and the 1,1-insertion of isonitriles into terminal alkynes [17], comprise some of these processes that have been studied in the past. In comparison with more common tetravalent and hexavalent organouranium complexes [18], pentavalent systems are rare. Although the chemistry of uranium is well documented [18,19], the literature on U(V) remains scrawny as compared to that of U(III), U(IV), or U(VI). Presumably this is a result of the tendency of solutions of U(V) to disproportionate to U(IV) and U(VI) as well as of the sensitivity of U(V) towards oxidation. For uranium(V), inorganic compounds of halides, alkoxides, oxides, and oxyhalides [20], have been disclosed. In addition, pentavalent uranium complexes containing metal–nitrogen and metal–oxygen multiple bonds have been reported [21]. Very few examples of organometallic uranium(V) complexes have been documented and thoroughly characterized [22], and the development of new types of organometallic U(V) complexes still remains a challenge.
In the last years, there has been a great interest in the reactivity and coordination chemistry of highly basic imidazolin-2-iminato ligands. The thermally stable imidazolin-2-ylidenes (2), which are prepared from the corresponding imidazolium salts (1), undergo a Staudinger-like reaction upon treatment with trimethylsilyl azide, Me3SiN3, to furnish N-silylated 2-iminoimidazolines 3 (Eq. (1)) [23], which can be used to stabilize complexes containing early transition metals or metals in higher oxidation states. Because of their 2σ,4π valence electron set, the imidazolin-2-iminato ligand class (II) can be regarded as being isolobal to monoanionic cyclopentadienyl systems (I) [24], and also as monoanionic imido ligands [25,26]. The distinct stability of N-heterocyclic carbenes of the imidazolin-2-ylidene type can be attributed inter alia to the capability of the imidazolium ring to effectively stabilize a positive charge, leading to strongly basic and highly nucleophilic ligands. This behaviour can be easily transferred to an exocyclic moiety in the 2-position of the N-heterocycle, so that a strong contribution from the ylidic mesomeric structure IIB must be considered for II (Fig. 1). The resulting build-up of negative charge at the exocyclic nitrogen atom leads to compounds with considerably enhanced basicity and nucleophilicity. In addition, compounds II can also act “only” as 2- or 4-electron donors, which provides a more flexible coordination to metal centres [27].
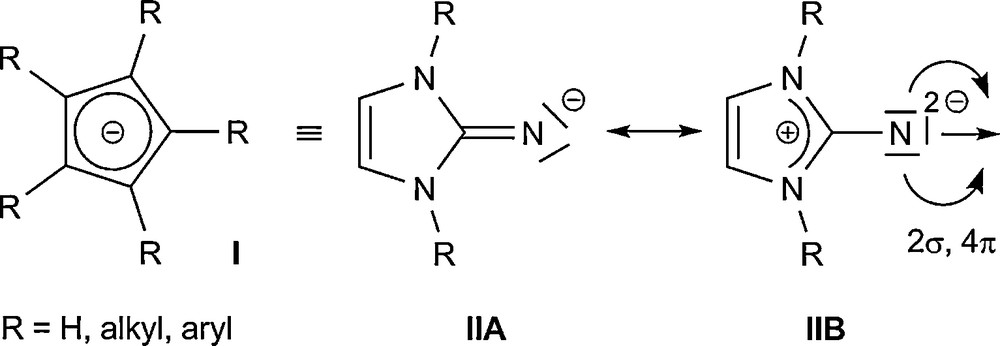
Isolobal analogy between superbasic imidazolin-2-iminato ligands II and cyclopentadienyls I.
In this communication, we present the one-electron oxidation of the organometallic metallocene Cp*2UCl2 with the N-silylated 2-iminoimidazoline 3, producing the imino U(V) dichloride complex 4 (Equation 2). To understand the bonding and other geometrical parameters of this complex, we have additionally prepared an ate complex of the same metallocene by reacting Cp*2UCl2 with the corresponding imidazolium salt, producing the anionic [Cp*2UCl3] complex. The structural analyses of the compounds are presented and compared.
2 Results and discussion
We have shown that the organouranium-silicon bonds are extremely active and their formation is mainly tampered by side reactions [16]. In addition, Evans et al. have shown that U(III) reacts with an N-heterocyclic carbene yielding as a side product an U(IV)-oxo complex [28]. Based on these results, we speculate that the reaction of the metallocene Cp*2UCl2 with N-silylated 2-iminoimidazoline 3 will probably induce a one electron oxidation of the metal complex towards a stable U(V) instead of the formation of the corresponding U(IV) imine, with the concomitant elimination of TMSCl. The reaction of stoichiometric amounts of N-silylated 2-iminoimidazoline 3 with Cp*2UCl2 in refluxing toluene under argon produced, after 24 h 78%, the complex 4 (Eq. (2)).
As expected, in complex 4 the metal is formally a U(V), and the silyl group was eliminated but not as TMSCl, and the remaining N-heterocyclic ring was opened forming the corresponding imine. Since the reaction was performed in toluene, we expected that the additional two hydrogen atoms would be from the solvent. It is noteworthy to address that we were unable to observe any silylated toluene (or benzyl) or hexamethyldisilane.
However, when the reaction was performed in perdeuterated toluene, no reaction towards complex 4 was observed (a myriad of complexes were obtained). This result clearly indicates that the removing of protons from the deuterated solvent allows competing reactions involving possibly also the TMS group of the ligand. The crystal structure of complex 4 is presented in Fig. 2. Crystallographic data, and structure refinement details for complex 4 and selected bond lengths and angles are collected in Tables 1 and 2, respectively.
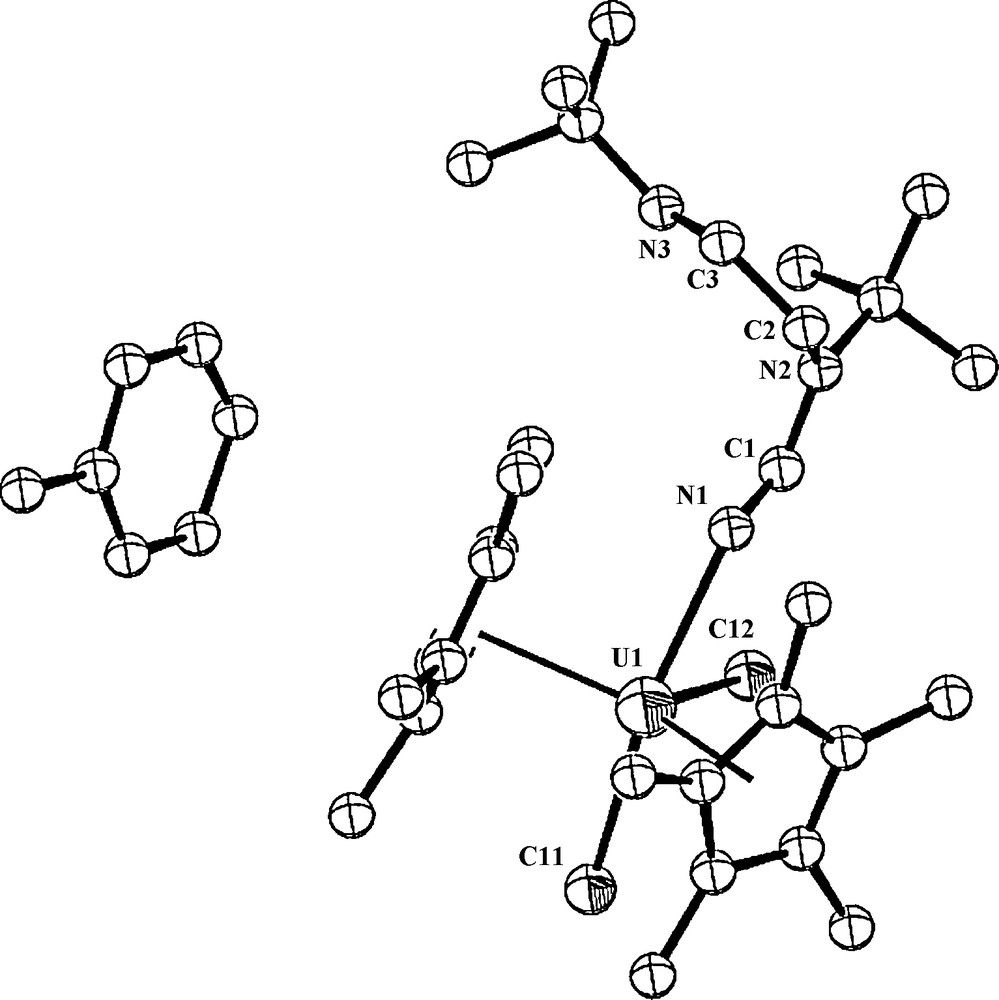
ORTEP diagram of complex 4 showing the toluene adduct (50% thermal ellipsoids).
Crystallographic data for complexes 4 (crystallized with toluene and water) and 5.
Complex | 4 toluene | 4 0.25H2O | 5 |
Empirical formula | C38H60Cl2N3U | C31H53.50Cl2N3O0.25U | C31H50Cl3N2U |
Fw[g/mol] | 867.82 | 781.20 | 796.12 |
T[K] | 293(2) | 240(2) | 240.0(2) |
λ[Å] | 0.71073 | 0.71073 | 0.71073 |
Crystal system | Monoclinic | Monoclinic | Monoclinic |
Space group | Pc | C2/c | P21/c |
a[Å] | 8.438(2) | 43.274(9) | 11.229(2) |
b[Å] | 12.034(2) | 15.723(3) | 19.399(4) |
c[Å] | 19.699(4) | 23.411(4) | 31.135(6) |
β[deg.] | 95.27(2) | 112.82(3) | 90.33(2) |
V[Å3] | 1988.8(7) | 14682(5) | 6782(2) |
Z | 2 | 16 | 8 |
ρ[g/cm3] | 1.449 | 1.414 | 1.559 |
μ(Mo Kα)[mm-1] | 4.243 | 4.589 | 5.044 |
R1,wR2(I > 2σ(I)) | 0.0416, 0.0673 | 0.0647, 0.1475 | 0.0514, 0.0651 |
R1,wR2 (all data) | 0.0727, 0.0731 | 0.1334, 0.1750 | 0.1216, 0.0742 |
GOF on F^2 | 0.789 | 1.002 | 0.906 |
F(000) | 870 | 6216 | 3152 |
θ range for data collection [deg.] | 1.69 to 25.34 | 1.76 to 25.02 | 1.31 to 25.04 |
Limiting indices | −10 ≤ h ≤ 10 −14 ≤ k ≤ 14 −23 ≤ l ≤ 23 | −50 ≤ h ≤ 51 −17 ≤ k ≤ 16 −27 ≤ l ≤ 27 | −12 ≤ h ≤ 12 −23 ≤ k ≤ 18 −36 ≤ l ≤ 37 |
Reflections collected/unique | 19009/6901 | 45585/12488 | 34957/11728 |
R(int) | 0.0947 | 0.0990 | 0.06102 |
Completeness to θmax [%] | 99.9 | 96.2 | 97.6 |
Data/restraints/parameters | 6901/17/386 | 12488/6/614 | 11728/0/705 |
Max. diff. peak & hole[e/Å-3] | 0.523 & −0.709 | 1.883 & −2.119 | 0.987 & −0.630 |
Key bond lengths [Å] and angles [deg.] for complex 4.
Bond lengths [Å] | |||||
Complex 4a | Complex 4 H2Ob | Complex 4 H2Ob | |||
U(1)-N(1) | 2.430(7) | U(1)-N(1) | 2.406(8) | U(1A)-N(1A) | 2.400(8) |
U(1)-Cl(1) | 2.631(4) | U(1)-Cl(1) | 2.663(3) | U(1A)-Cl(1A) | 2.665(4) |
U(1)-Cl(2) | 2.672(5) | U(1)-Cl(2) | 2.640(3) | U(1A)-Cl(2A) | 2.653(4) |
N(1)-C(21) | 1.258(12) | N(1)-C(21) | 1.228(15) | N(1A)-C(21A) | 1.217(15) |
C(21)-N(2) | 1.363(14) | C(21)-N(2) | 1.361(15) | C(21A)-N(2A) | 1.346(15) |
N(2)-C(22) | 1.466(12) | N(2)-C(22) | 1.457(15) | N(2A)-C(22A) | 1.481(16) |
C(22)-C(23) | 1.478(13) | C(22)-C(23) | 1.486(18) | C(22A)-C(23A) | 1.527(18) |
C(23)-N(3) | 1.248(11) | C(23)-N(3) | 1.283(16) | C(23A)-N(3A) | 1.237(16) |
Bond angles [°] | |||||
Complex 4 | 4 0.25 H2O | 4 0.25 H2O | |||
N(1)-U(1)-Cl(1) | 156.89(19) | N(1)-U(1)-Cl(1) | 76.3(2) | N(1A)-U(1A)-Cl(1A) | 75.4(2) |
N(1)-U(1)-Cl(2) | 76.33(19) | N(1)-U(1)-Cl(2) | 157.4(2) | N(1A)-U(1A)-Cl(2A) | 156.4(2) |
Cl(1)-U(1)-Cl(2) | 80.58(13) | Cl(1)-U(1)-Cl(2) | 81.38(11) | Cl(1A)-U(1A)-Cl(2A) | 81.15(13) |
C(1)-N(1)-U(1) | 131.2(8) | C(21)-N(1)-U(1) | 136.6(8) | C(21A)-N(1A)-U(1A) | 136.8(8) |
C(1)-N(2)-C(2) | 121.4(8) | C(21)-N(2)-C(22) | 117.6(10) | C(21A)-N(2A)-C(22A) | 116.3(11) |
N(1)-C(1)-N(2) | 120.6(11) | N(1)-C(21)-N(2) | 126.0(11) | N(1A)-C(21A)-N(2A) | 128.2(11) |
N(3)-C(3)-C(2) | 123.4(9) | N(3)-C(23)-C(22) | 122.7(12) | N(3A)-C(23A)-C(22A) | 125.6(13) |
a 4 is the complex that crystallizes with one molecule of toluene.
b 4 0.25 H2O is the complex with two independent molecules that crystallizes with one water molecule.
Complex 4 crystallizes with one molecule of toluene in the crystal unit cell. The metal coordination can be described as a highly distorted trigonal bipyramid. The central equatorial plane resembles a T-shape with N(1)-U(1)-Cl(1) = 76.3°, N(1)-U(1)-Cl(2) = 203.1° and Cl(1)-U(1)-Cl(2) = 80.56°, and the metal is located at the center of the plane (sum of the angles around the metal = 359.98°). The two equal metal–Cp* distances are 2.47 Å and the angle Cp*(centroid)–U–Cp*(centroid) is 135.8° with a dihedral angle between the Cp* planes of 40.38°. Uranium(V) complexes containing UCl bonds have been previously structurally characterized, with typical bond lengths ranging from 2.4 to 2.7 Å [20,29]. The UCl bond lengths observed for complex 4 fit in this range and also compare well with the metrical parameters observed for other organometallic uranium complexes exhibiting UCl bonds [29].
When comparing the Cp*–U–Cp* angle, U–Cp* and the UCl bond distances for various oxidation states in different organouranium complexes, in all cases, the similarity indicates that these geometrical parameters are insensitive to the oxidation state. Moreover, the U–N(imine) bond length of 2.41 Å and U–N(1)–C(1) angle of 131.2° are in agreement with other uranium–imine bond lengths and angles [9a,30], again showing an insensitivity to the oxidation state. It is important to point out that a difference is indeed encountered when we compare the imine (U–N=R) with the corresponding imido (U=N–R) distances, since the latter bonds are generally shorter (U=N=1.958(6)–1.975(6) Å), and the corresponding angles are closer to linearity (169.6(4)–172.2(9)°) [22g,22h].
To try to get an insight into this peculiar reaction, we have performed the reaction with two equivalents of water (as the proton source) in refluxing toluene for 24 h, resulting in the formation of the same product 4, however, with a lower isolated yield of 55%. Interestingly, this complex co-crystallizes with one molecule of water (Fig. 3).

ORTEP diagram of complex 4 with a co-crystallized water molecule (4·H2O) (50% thermal ellipsoids).
The crystal structure of the complex 4·H2O (with water) is presented in Fig. 3, and the crystallographic data and structure refinement details together with selected bond lengths and angles are collected in Tables 1 and 2, respectively. The metal coordination sphere is disposed similarly, a distorted T-shape bipyramid, as in complex 4 with co-crystallized toluene, however, the asymmetric unit contains two independent molecules. The central equatorial plane resembles again a T-shape with the metal disposed at the center of the plane (sum of the angles around the metal in both molecules are 360.28° and 360.15°). In this complex, 4·H2O, two different distances U–Cp*cent = 2.29 and 2.46 Å are encountered for the first molecule. The Cp*(centroid)–U– Cp*(centroid) angle is 138.0°, and the dihedral angle between the Cp* planes is 40.04 ° for the first independent molecule, whereas for the second molecule, which is more symmetrical, similar geometric parameters are observed: U–Cp*(centroid) = 2.47 Å; the Cp*(centroid)–U– Cp*(centroid) = 137.8° and the angle between the Cp* planes is 41.71°.
It is interesting to note that the water molecule in the crystal does not display any interactions with the metal center, presumably because of the closed coordination shell. In general, both complexes 4 and 4·H2O are isostructural with minor differences due to packing forces.
Since no major differences in bond lengths and angles are observed for similar motifs at several organouranium complexes with various oxidation states, we decided to examine the isolobal relationship, by preparing a similar anionic moiety with a non-coordinating counterion. Hence, the reaction of Cp*2UCl2 with the N-heterocyclic imidazolium chloride salt results in a chloride transfer to form the corresponding organometallic uranate complex 5 ((Eq. (3)).
The crystal structure of complex 5 is presented in Fig. 4. Crystallographic data and structure refinement details for complex 5 and selected bond lengths and angles are collected in Tables 1 and 3, respectively. The complex crystallizes with two independent molecules in the unit cell. The metal coordination can again be described as a distorted T-shaped bipyramid. The central equatorial plane is bisected symmetrically by the vector U-Cl(3) with Cl(1)–U(1)–Cl(2) = 204.89°, Cl(1)–U(1)–Cl(3) = 77.45° and Cl(3)–U(1)–Cl(2) = 77.72° with the metal at the center of the plane (sum of the angles around the metal in both independent molecules = 360.06° and 360.09°, respectively). The metal–Cp* distances in both molecules are the same (2.50 Å), which are somewhat longer than observed for complexes 4 and 4·H2O as expected for the anionic complex, and the Cp*(centroid)–U–Cp*(centroid) angles are 136.8° and 131.8°; for the two independent molecules. The uranium complex 5 contains two identical and one slightly longer U–Cl bonds; U–Cl(1) = 2.684(2), U–Cl(2) = 2.685(2), U–Cl(3) = 2.692(5), and U–Cl(1) = 2.733(2), U–Cl(2) = 2.684(2), U–Cl(3) = 2.680(2)Å, for the two independent molecules, respectively. The U–Cl bond lengths observed for both independent molecules of complex 5 are in good agreement with the metrical parameters observed for other organometallic uranium complexes exhibiting U–Cl bonds [20].
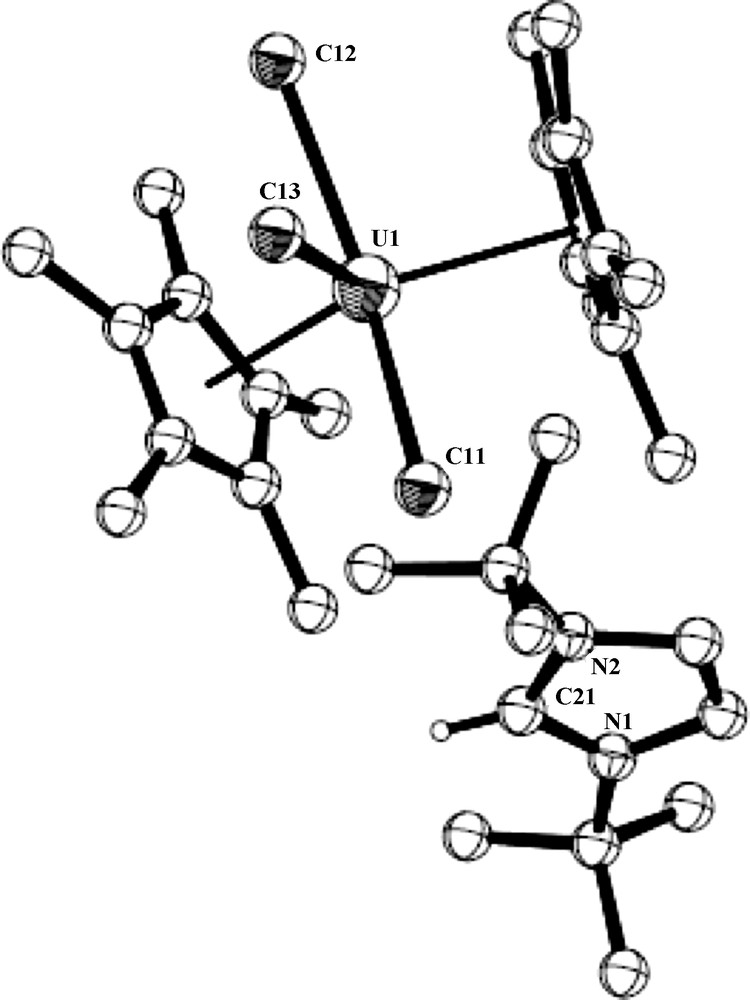
ORTEP diagram of complex 5 (50% thermal ellipsoids).
Key bond lengths [Å] and angles [deg] for complex 5.
Bond lengths [Å] | |||
U(1)-Cl(1) | 2.684(2) | U(1A)-Cl(1A) | 2.733(2) |
U(1)-Cl(2) | 2.692(2) | U(1A)-Cl(2A) | 2.6840(18) |
U(1)-Cl(3) | 2.685(2) | U(1A)-Cl(3A) | 2.6800(19) |
N(1)-C(21)- | 1.324(8) | N(1A)-C(21A) | 1.312(9) |
N(2)-C(21) | 1.339(9) | N(2A)-C(21A) | 1.313(8) |
Bond angles [°] | |||
Cl(1)-U(1)-Cl(2) | 155.11(7) | Cl(1A)-U(1A)-Cl(2A) | 75.56(6) |
Cl(1)-U(1)-Cl(3) | 77.45(8) | Cl(1A)-U(1A)-Cl(3A) | 76.99(7) |
Cl(2)-U(1)-Cl(2) | 77.72(8) | Cl(2A)-U(1A)-Cl(3A) | 152.46(6) |
When comparing the Cp–U–Cp angles, U–Cp and the U–Cl bond distances between the isolobal U(V) and U(IV) complexes 4 and 5, the similarity of these geometrical parameters indicates again the insensitivity to the oxidation state and to the ionic character of the metal. It is important to point out that the T-shape that is obtained between the metal and the equatorial ligands in both complexes is a consequence of the localized empty orbital at the equatorial girdle between the trans chlorine atoms. This behavior is also obtained in other early transition complexes, which is responsible for the electrophilicity of these metals as observed in many U(IV) complexes containing the Cp*2UCl2 unit and a molecule with a Lewis base atom donating a pair of electrons [30,31]. Presently, we are performing experiments to try to gain an insight into the mechanism of the reaction and the final fate of the TMS group.
3 Conclusions
The synthesis of a stable organometallic dichlorouranium(V) imine complex was accomplished by the one electron oxidation of Cp*2UCl2 with the N-silylated 2-iminoimidazoline, leading to an opening of the N-heterocyclic ring. A similar reaction in the presence of two equimolar amounts of water also leads to the formation of the same U(V) complex, which contains an isolated water molecule in the crystal unit cell. The corresponding isolobal ate complex [Cp*2UCl3]− was prepared from the reaction of Cp*2UCl2 with the corresponding imidazolium salt producing the anionic complex. The structural analysis of all the complexes are presented and compared.
4 Experimental section
4.1 General procedures
All manipulations of air-sensitive materials were carried out with the rigorous exclusion of oxygen and moisture in an oven-dried or flamed Schlenk-type glassware on a dual-manifold Schlenk line, or interfaced to a high vacuum (10−5 Torr) line, or in a nitrogen-filled vacuum atmospheres glove-box with a medium-capacity recirculator (1–2 ppm O2). Argon and nitrogen were purified by passage through a MnO oxygen-removal column and a Davison 4 Å molecular sieve column. Analytically pure solvents were distilled under nitrogen from potassium benzophenone ketyl (THF), Na (toluenes), and Na/K alloy (hexane, toluene-d8, THF-d8). Cp*2UCl2, [32] 1,3-di-tert-butylimidazolin-2-ylidene, [33] and 1,3-tert-butyl-2-(trimethylsilylimino)imidazoline were prepared according to literature procedures
4.2 X-ray Crystallographic measurements
The single crystalline material was immersed in Paratone-N oil and was quickly removed with a capillary tube and mounted on the Kappa CCD diffractometer under a cold stream of nitrogen at 230–270 K. Data collection was performed using monochromatized Mo Kα radiation using omega scans and phi scans to cover the Ewald sphere. [34]. Accurate cell parameters were obtained with the amount of indicated reflections (Table 1) [35]. The structure was solved by SHELXS97 direct methods [36], and refined by SHELXL97 program package [37]. The atoms were refined anisotropically. Hydrogen was included using the riding mode. Software used for molecular graphics: ORTEP, TEXRAY Structure Analysis package [38]. Cell parameters and refinement data is presented in Table 1.
4.3 Synthesis of Cp*2UCl2(NCHN(tBu)CH2CHN(tBu)·C7H8)
Inside a glovebox, 100 mg (0.1726 mmol) of Cp*2UCl2 and 46.17 mg (0.1726 mmol) of 1,3-tert-butyl-2-(trimethylsilylimino)imidazoline was loaded in a round bottom flask, which was connected to a condenser fitted with a Teflon valve side arm connector. The system was connected to a high vacuum line, and 25 ml of dry toluene were condensed onto the flask. The reaction mixture was refluxed at 110 °C for 28 h, cooled down under argon, and the solvent was removed under vacuum to obtain a reddish brown compound. Then the system was taken back to the glovebox and connected to a swivel frit. This system was again connected to the high vacuum line, and the reddish brown compound was washed three times with cold hexane giving an isolated solid yield of 78%. The reddish brown compound was dissolved in toluene and allowed to stand at −40 °C to obtain suitable crystals. Rapid cooling of the solution yields analytically pure material that contains no solvent. Analysis was calculated for C31H52Cl2N3U: C 48.00, H 6.76, N 5.42, Cl 9.14; found C 48.12, H 6.93, N 5.77, Cl 9.32.
4.4 Synthesis of Cp*2UCl2(NCHN(tBu)CH2CHN(tBu)·H2O)
Inside a glovebox, 100 mg (0.1726 mmol) of Cp*2UCl2 and 46.17 mg (0.1726 mmol) of 1,3-tert-butyl-2-(trimethylsilylimino)imidazoline was loaded in a round bottom flask, which was connected to a condenser fitted with a Teflon® valve side arm connector. The system was connected to a high vacuum line, and 25 ml of dry toluene containing 6.2 mg (0.3456 mmol) of water was condensed onto the flask. The reaction mixture was heated at 98 °C for 28 h, cooled down under argon, and the solvent was removed under vacuum to obtain a reddish brown compound. Then the system was taken back to a glovebox and connected to a swivel frit. This system was connected to the high vacuum line, and the reddish brown compound was washed three times with cold hexane giving an isolated solid yield of 55%. The reddish brown compound was dissolved in toluene and allowed to stand at −40 °C to obtain suitable crystals. Analysis was calculated for C31H54Cl2N3OU: C 46.91, H 6.86, N 5.29, Cl 8.93; found C 47.02, H 6.92, N 5.37, Cl 8.62.
4.5 Synthesis of (1,3-di-tert-butylimidazolium)-bis(pentamethylcyclopentadienyl)trichlorouranate(IV)
Inside a glovebox, 100 mg (0.1726 mmol) of Cp*2UCl2 and 46.17 mg (0.1726 mmol) of 1,3-di-tert-butylimidazolium chloride was loaded in a round bottom flask, which was connected to a condenser fitted with a Teflon® valve side arm connector. The system was connected to a high vacuum line, and 25 ml of dry toluene was condensed onto the flask. The reaction mixture was refluxed at 110°C for 48 h, cooled down under argon, and the solvent was removed under vacuum to obtain a red-brown compound. Then the system was taken back to glovebox and connected to a swivel frit. This system was again connected to the high vacuum line, and the compound was washed three times with cold hexane, giving an isolated solid yield of 53%. The reddish brown compound was dissolved in toluene and allowed to stand at −40 °C to obtain suitable crystals. Analysis was calculated for C31H51Cl3N2U: C, 46.77; H, 6.46; Cl, 13.36; N, 3.52; found C 46.39, H 6.77, N 3.30, Cl 12.92.
Acknowledgments
This research was partially supported by the Israel Science Foundation, administered by the Israel Academy of Science and Humanities, under Contract No. 518/09 and by the fund of the Lower Saxony Ministry of Science and Culture for Joint Lower Saxony - Israeli Research Projects.