1 Introduction
Over the years, nitrile oxides have become important building blocks in organic synthesis. The nitrile oxides-olefin 1,3-dipolar cycloaddition is a powerful reaction in that it can create as many as two new contiguous stereogenic centres in a single step [1]. In general, a reductive ring opening of Δ2-isoxazolines can result in either complete reduction to an amino alcohol or NO bond reduction/hydrolysis to β-hydroxy ketones through hydroxyimine intermediates [2]. We envisioned that a judicious choice of reducing agent other than the reported catalytic hydrogenolysis [3] to give the vinylogous amide derivatives allowing the synthesis of many products of potential interest [4]. Based on an evaluation of the nitrile oxides cycloaddition, it was felt that the stereochemistry of these new centers could be controlled if the reaction system were properly designed. As a continuation of our effort to utilize heterocyclic compounds as dipolarophiles in 1,3-dipolar cycloaddition reactions [5], we report the cycloaddition of aromatic nitrile oxides with chiral 5-hydroxy-4-methyl-1,5-dihydropyrrol-2-one derivatives.
2 Results and discussion
The synthetic route to the targeted Δ2-isoxazolines 3ae-be is outlined in Scheme 2. The 1,3-dipolar cycloaddition of 5-hydroxy-4-methyl-1,5-dihydropyrrol-2-ones 1a-b with aromatic nitrile oxides 2c-e at 110 °C in toluene solution for 2h, compound 3ae-be was obtained (Scheme 1).
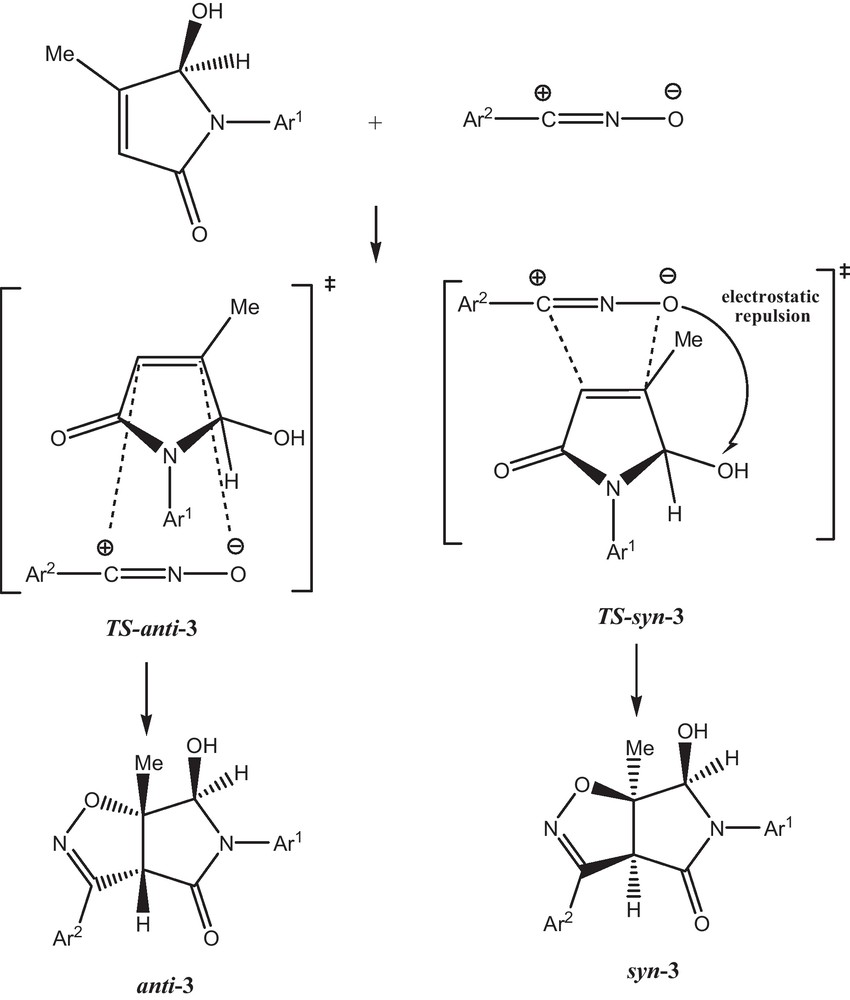

The 1,3-dipolar cycloaddition of arylnitrile oxides is, in each case, regiospecific. The chemical shifts of C-6a (13C NMR) are in excellent agreement with those usually obtained when this quaternary carbon is attached to oxygen atom. The syn or anti stereochemistry of this cycloaddition product was determined from a NOESY spectrum. The trans relationship between protons 3a-H and 6-H was deduced from absence of an NOE effect. The complete anti-selectivity observed in reactions with 5-hydroxy-4-methyl-1,5-dihydropyrrol-2-ones, electrostatic repulsion should account for the observed results. Moreover, syn orientation of the oxygen-containing pyrrolidinones to the oxygen atom of nitrile oxide leads to greater repulsion in the transition state (Scheme 2) [6].
A similar mode of addition has been reported earlier for the reaction of 5-alkoxy-2(5H)-furanone with aromatic nitrile oxides [7] as well as by other dipoles [8]; it is mainly determined by HOMO, LUMO interactions [9].
The Δ2-isoxazolines 3ac-be proved unexpectedly resistant to reduction by a variety of reducing agents, including zinc-acetic acid system [10], sodium borohydride with either NiCl2.6H2O or CoCl2.6H2O [11], lithium aluminium hydride [12], sodium in ethanol, hydrogenation over nickel, palladium, or platinum oxide [13], and Mo(CO)6 [14]. Reduction of 3 to the vinylogous amide derivatives 4 was achieved cleanly and in high yield by using Fe/NH4Cl in the presence of water [15] (Scheme 3).
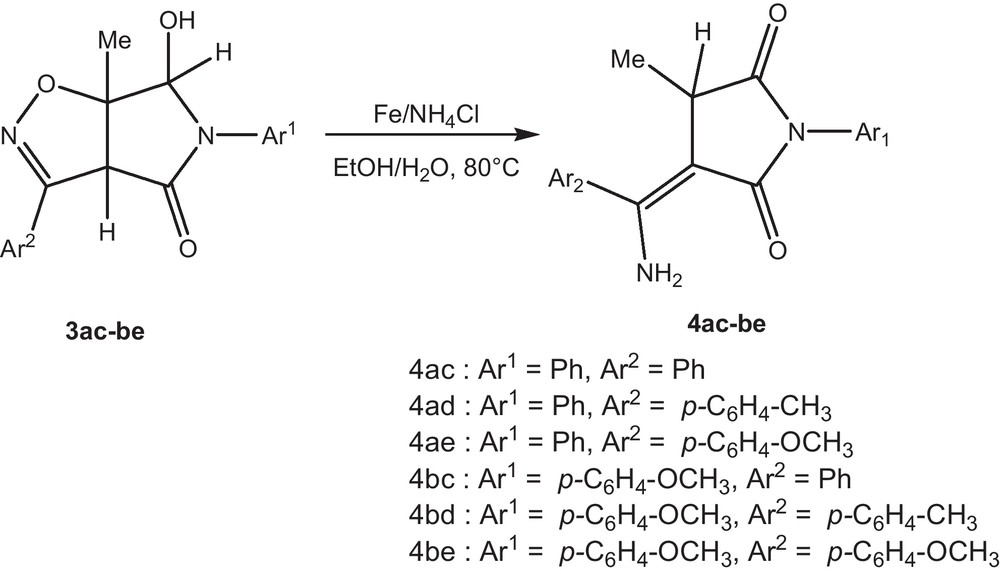
1H NMR, 13C NMR, IR, HRMS, and elemental analyses characterized all the synthesized vinylogous amide derivatives 4. 1H NMR of 4bc showed one doublet at 0.72 ppm corresponding to three methyl protons and showed multiplet at 3.62–3.67 for one proton attached at carbon C-2, three protons correspond to OCH3 group. Two doublet at 7.00 and 7.21 ppm are due to the AA′BB′ system corresponding to aromatic protons. The two NH groups appeared as a broad singulet at 7.39 and 8.22 ppm. The formation of the product was further confirmed by correct elemental analyses. The appearance of the two NH signals at different chemical shifts would be expected, since one of them will be intramolecularly H-bonded to the adjacent CO. Also, the Z configuration was deduced from observation of an NOE effect between ortho-aromatic protons and the methyl protons at C4.
The plausible mechanism for the formation of isoxazolines 4 is given in Scheme 4. The back-donation from a π d filled orbital of Fe to the lumo π* of isoxazoles, which should facilitate the NO bond cleavage give the intermediate 3′. The dehydration of the intermediate 3′ leads to the formation of intermediate 3′′. The final product of the cycloaddition 4 is obtained by isomerization of intermediate 3′′.
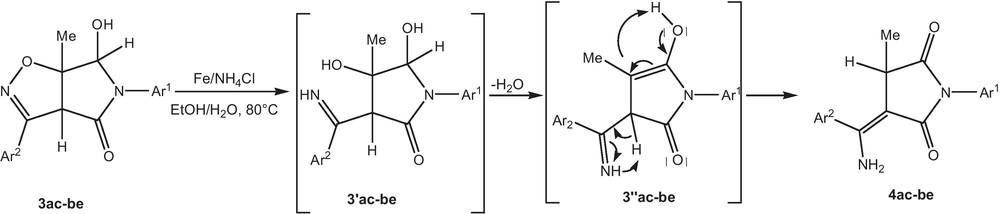
3 Conclusion
In conclusion, our studies have revealed that the 1,3-dipolar cycloaddition of aromatic nitrile oxides with various electron deficient olefins gives bicyclic Δ2-isoxazolines with complete regio- and stereo-selectivity. The methyl group increases the dipolarophilic reactivity of the pyrrolidinones, as well as their regioselectivity. Finally, the electrostatic repulsion between oxygen-containing pyrrolidinones and the oxygen atom of nitrile oxide are the main reasons for the observed anti-selectivity. These heterocycles were reduced to unexpected vinylogous amide derivatives using iron and ammonium chloride.
4 Experimental details
Infrared spectra were recorded on a Perkin-Elmer IR-197 spectrophotometer in KBr discs. NMR spectra were obtained with a Bruker AC 300 spectrometer operating at 300 MHz for 1H and at 75.64 MHz for 13C using TMS as the internal standard. Elemental analysis was performed with a Perkin-Elmer 240B microanalyzer. High-resolution mass spectra (HRMS) were recorded on a Waters Micromass GCT instrument. The melting points, thermal transitions, and mesomorphic textures were determined using an Olympus BX50 microscope equipped with a Mettler Toledo FP-82 hot-stage and a PM-30 exposure control unit. All the reagents were obtained from commercial sources and used without further purification. 5-hydroxy-4-methyl-1,5-dihydropyrrol-2-ones 1a-b were obtained by the reduction of citraconimide derivatives with NaBH4 [16]. The aromatic nitrile oxides 2c-e were prepared according to published procedures [17]. The organic solvents were of commercial grade quality and all were dried by traditional methods. In general, all the compounds were purified by column chromatography on silica gel (60–120 mesh), and crystallization from analytical grade solvents. The purity of the sample was checked by thin-layer chromatography (Merck Kieselgel 60F254).
4.1 General procedure for the addition of aromatic nitrile oxides to 5-hydroxy-1,5-dihydropyrrol-2-one derivatives
A solution of dipolarophiles 1a-b (1 mmol) and chloroximes 2c-e (1.1 mmol) in toluene (10 mL), was stirred at 110 °C. To this solution, trimethylamine (0.2 mL), dissolved in toluene (10 mL), was added dropwise. The precipitated triethylammonium chloride was removed by filtration and the filtrate was concentrated in vacuo, and chromatography (SiO2; ethyl acetate/petroleum ether, 2:1) to afford compounds 3ac-be.
4.1.1 (3aR*,6R*,6aS*)-6-exo-hydroxy-6a-methyl-3,5-diphenyl-3a,5,6,6a-tetrahydro-4H-pyrrolo[3,4-d]isoxazole-4-one 3ac
Yield (214 mg, 70%), white solid. M.p = 224–225 °C. IR (KBr) υmax/cm−1: 1640 (CN); 1740 (CO); 3300 (OH). 1H-NMR (DMSO) δ: 1.62 (s, 3H, CH3), 4.11 (s, 1H, 3a–H), 4.61 (d, 1H, OH, J = 7.8 Hz), 6.49 (d, 1H, 6-H, J = 7.8 Hz), 7.23–7.81 (m, 10H, Harom). 13C{1H}NMR (DMSO) δ: 19.12, 61.04, 89.35, 91.09, 122.90–137.00, 153.97, 169.01. Elemental analysis: C18H16N2O3 requires C, 70.12; H, 5.23; N, 9.09%; Found: C, 70.15; H, 5.35; N, 8.99%.
4.1.2 (3aR*,6R*,6aS*)-6-exo-hydroxy-6a-methyl-3-(4-methylphenyl)-5-phenyl-3a,5,6,6a-tetrahydro-4H-pyrrolo[3,4-d]isoxazole-4-one 3ad
Yield (256 mg, 80%), white solid. M.p = 191–192 °C. IR (KBr) υmax/cm−1: 1635 (CN); 1735 (CO); 3300 (OH). 1H-NMR (DMSO) δ: 1.66 (s, 3H, CH3), 2.52 (s, 3H, CH3), 4.19 (s, 1H, 3a-H), 4.53 (d, 1H, OH, J = 7.5 Hz), 6.61 (d, 1H, 6-H, J = 7.5 Hz), 7.36–7.80 (m, 9H, Harom). 13C{1H}NMR (DMSO) δ: 19.20, 21.01, 60.02, 89.40, 90.93, 123.45–140.89, 154.31, 168.59. Elemental analysis: C19H18N2O3 requires C, 70.79; H, 5.63; N, 8.69%; Found: C, 70.75; H, 5.56; N, 8.54%.
4.1.3 (3aR*,6R*,6aS*)-6-exo-hydroxy-3-(4-methoxyphenyl)-6a-methyl-5-phenyl-3a,5,6,6a-tetrahydro-4H-pyrrolo[3,4-d]isoxazole-4-one 3ae
Yield (236 mg, 70%), white solid. M.p = 202–203 °C. IR (KBr) υmax/cm−1: 1630 (CN); 1740 (CO); 3300 (OH). 1H-NMR (DMSO) δ: 1.69 (s, 3H, CH3), 3.80 (s, 3H, OCH3), 4.18 (s, 1H, 3a-H), 4.56 (d, 1H, OH, J = 7.5 Hz), 6.53 (d, 1H, 6-H, J = 7.5 Hz), 7.07–7.69 (m, 9H, Harom). 13C{1H}NMR (DMSO) δ: 20.24, 55.74, 60.26, 84.45, 89.02, 114.98–161.31, 156.28, 170.45. Elemental analysis: C19H18N2O4 requires C, 67.44; H, 5.36; N, 8.28; Found: C, 67.55; H, 5.45; N, 8.31%.
4.1.4 (3aR*,6R*,6aS*)-6-exo-hydroxy-5-(4-methoxyphenyl)-6a-methyl-3-phenyl-3a,5,6,6a tetrahydro-4H-pyrrolo[3,4-d]isoxazole-4-one 3bc
Yield (276 mg, 90%), white solid. M.p = 178–179 °C. IR (KBr) υmax/cm−1: 1635 (CN); 1737 (CO); 3300 (OH). 1H-NMR (DMSO) δ: 1.44 (s, 3H, CH3), 3.82 (s, 3H, OCH3), 4.70 (s, 1H, 3a–H), 5.56 (d, 1H, OH, J = 7.5 Hz), 6.94 (d, 1H, 6-H, J = 7.5 Hz), 7.02 (d, 2H) and 7.83 (d, 2H): AA′BB′ part. J = 9 Hz, 7.21–7.58 (m, 5H, Harom). 13C{1H}NMR (DMSO) δ: 19.02, 55.70, 59.50, 87.83, 91.71, 114.38–161.18, 154.38, 167.65. Elemental analysis: C19H18N2O4 requires C, 67.44; H, 5.36; N, 8.28%; Found: C, 67.50; H, 5.41; N, 8.33%.
4.1.5 (3aR*,6R*,6aS*)-6-exo-hydroxy-5-(4-methoxyphenyl)-6a-methyl-3-(4-methylphenyl)-3a,5,6,6a-tetrahydro-4H-pyrrolo[3,4-d]isoxazole-4-one 3bd
Yield (212 mg, 60%), white solid. M.p = 169–170 °C. IR (KBr) υmax/cm−1: 1643 (CN); 1744 (CO); 3300 (OH). 1H-NMR (DMSO) δ: 1.45 (s, 3H, CH3), 2.37 (s, 3H, CH3), 3.77 (s, 3H, OCH3), 4.67 (s, 1H, 3a-H), 5.47 (d, 1H, OH, J = 7.8 Hz), 6.91 (d, 1H, 6-H, J = 7.8 Hz), 6.96 (d, 2H) and 7.41 (d, 2H): AA’BB’ part. J = 9 Hz, 7.29 (d, 2H) and 7.80 (d, 2H): AA′BB′ part. J = 7.8 Hz. 13C{1H}NMR (DMSO) δ: 19.06, 21.36, 55.61, 59.15, 88.15, 92.04, 114.11–157.67, 154.76, 167.40. Elemental analysis: C20H20N2O4 requires C, 68.17; H, 5.72; N, 7.95%; Found: C, 68.30; H, 5.67; N, 7.79%.
4.1.6 (3aR*,6R*,6aS*)-6-exo-hydroxy-3,5-di(4-methoxyphenyl)-6a-methyl-3a,5,6,6a-tetrahydro-4H-pyrrolo[3,4-d]isoxazole-4-one 3be
Yield (144 mg, 40%), white solid. M.p = 181–182 °C. IR (KBr) υmax/cm−1: 1638 (CN); 1733 (CO); 3300 (OH). 1H-NMR (DMSO) δ: 1.67 (s, 3H, CH3), 3,74 (s, 3H, OCH3), 3.82 (s, 3H, OCH3), 4.14 (s, 1H, 3a-H), 5.40 (d, 1H, OH, J = 7.8 Hz), 7.15 (d, 1H, 4-H, J = 7.8 Hz), 6.92 (d, 2H) and 7.36 (d, 2H): AA′BB′ part. J = 9 Hz, 7.06 (d, 2H) and 7.66 (d, 2H): AA′BB′ part. J = 8.7 Hz. 13C{1H}NMR (DMSO) δ: 20.31, 55.62, 55.74, 60.36, 84.77, 89.69, 114.36–161.28, 157.79, 170.23. Elemental analysis: C20H20N2O5 requires C, 65.21; H, 5.47; N, 7.60%; Found: C, 65.33; H, 5.45; N, 7.75%.
4.2 General procedure for reduction of isoxazolines
To a 100 mL round-bottomed flask isoxazoline (1 mmol), and NH4Cl (3 mmol) in ethanol and water (1:1, 10 mL) was added Fe powder (3 mmol). The mixture was heated to 80 °C and was allowed to stir at this temperature for 3 h. The EtOH was removed under reduced pressure and the reaction mixture was extracted with dichlorometane (3 × 50 mL). The organic solution was dried over Na2SO4, evaporated and chromatography (SiO2; ethyl acetate/petroleum ether, 3:1) to afford compounds 4ac-be.
4.2.1 (Z)-3-(amino(phenyl)methylene)-4-methyl-1-phenylpyrrolidine-2,5-dione 4ac
Yield (284 mg, 85%), white solid. M.p = 196–197 °C. IR (KBr) υmax/cm−1: 1732 (CO); 1735 (CO); 3330 (NH2).1H-NMR (DMSO)δ: 0.82 (d, J = 7.2 Hz, 3H), 3.74 (q, J = 7.2 Hz, 1H), 7.42 (br s, 1H), 7.47 to 7.65 (m, 10H), 8.35 (br s, 1H), 13C{1H}NMR (DMSO) δ: 16.6, 38.2, 93.3, 127.4, 127.8, 127.9, 129.0, 129.1, 130.2, 133.2, 136.2, 157.9, 170.6, 177.9. HRMS (M+H)+ requires 309.1233 found 309.1239. Elemental analysis: C18H16N2O2 requires C, 73.95; H, 5.52; N, 9.58; found C 73.85, H 5.66, N 9.61%.
4.2.2 (Z)-3-(amino(p-tolyl)methylene)-4-methyl-1-phenylpyrrolidine-2,5-dione 4ad
Yield (245 mg, 80%), white solid. M.p = 182–183 °C. IR (KBr) υmax/cm−1: 1730 (CO); 1736 (CO); 3330 (NH2).1H NMR (CDCl3)δ: 0.83 (d, J = 7.2 Hz, 3H), 1.65 (s, 3H), 3.57 (q, J = 7.2 Hz, 1H), 3.83 (s, 3H), 7.43 (br s, 1H), 6.94 to 7,55 (m, 8H), 8.35 (br s, 1H). 13C{1H}NMR (CDCl3) δ: 17.1, 39.0, 95.4, 114.6, 125.6, 127.3, 128.1, 128.9, 129.6, 155.2, 156.7, 159.3, 161.5, 171.8, 179.1. HRMS (M+H)+ requires 323.1390 found 323.1396. Elemental analysis: C19H18N2O2 requires C, C, 74.49; H, 5.92; N, 9.14; found C 75.63, H 5.87, N 9.09%.
4.2.3 (Z)-3-(amino(4-methoxyphenyl)methylene)-4-methyl-1-phenylpyrrolidine-2,5-dione 4ae
Yield (241 mg, 75%), white solid. M.p = 169–170 °C, IR (KBr) υmax/cm−1: 1735 (CO); 1740 (CO); 3330 (NH2), 1H NMR (DMSO)δ: 0.72 (d, J = 7.2 Hz, 3H), 2.50 (s, 3H), 3.61 (q, J = 7.2 Hz, 1H), 3.79 (s, 3H), 7.01 (J = 8.7 Hz, 2H), 7.21 (J = 8.7 Hz; 2H), 7.44 (br s, 1H), 7.53 to 7,56 (m, 5H), 8.19 (br s, 1H). 13C{1H}NMR (DMSO) δ: 16.5, 38.1, 55.7, 93.4, 114.2, 125.8, 127.8, 128.6, 129.1, 130.2, 136.2, 157.6, 158.8, 170.9, 178.1. HRMS (M+H)+ requires 339.1339 found 339.1342. Elemental analysis: C19H18N2O3 requires C, 70.79; H, 5.63; N, 8.69; found C 70.71, H 5.58, N 8.55%.
4.2.4 (Z)-3-(amino(phenyl)methylene)-1-(4-methoxyphenyl)-4-methylpyrrolidine-2,5-dione 4bc
Yield (306 mg, 95%), white solid. M.p = 174–175 °C. IR (KBr) υmax/cm−1: 1729 (CO); 1738 (CO); 3330 (NH2). 1H NMR (CDCl3)δ: 0.72 (d, J = 7.2 Hz, 3H), 3.62 (q, J = 7.2 Hz, 1H), 3.79 (s, 3H), 7.00 (J = 9.0 Hz, 2H), 7.21 (J = 9.0 Hz, 2H), 7.39 (br s, 1H), 7.51 to 7,59 (m, 5H), 8.22 (br s, 1H). 13C{1H}NMR (CDCl3) δ: 16.6, 38.1, 55.7, 93.4, 114.2, 125.8, 127.8, 128.6, 129.1, 130.1, 136.3, 157.6, 158.8, 170.9, 178.1. HRMS (M+H)+ requires 339.1339 found 339.1331. Elemental analysis: C19H18N2O3 requires C, 70.79; H, 5.63; N, 8.69; found C 70.82, H 5.59, N 8.75%.
4.2.5 (Z)-3-(amino(p-tolyl)methylene)-1-(4-methoxyphenyl)-4-methylpyrrolidine-2,5-dione 4bd
Yield (235 mg, 70%), white solid. M.p = 166–167 °C, IR (KBr) υmax/cm−1: 1737 (CO); 1737 (CO); 3330 (NH2). 1H NMR (CDCl3)δ: 0.84 (d, J = 7.2 Hz, 3H), 1.62 (s, 3H), 3.58 (q, J = 7.2 Hz, 1H), 3.83 (s, 3H), 4.75 (br s, 1H), 6.97 to 7,52 (m, 8H), 8.41 (br s, 1H). 13C{1H}NMR (CDCl3) δ: 17.0, 39.0, 55.8, 95.4, 114.7, 125.6, 127.2, 128.2, 128.8, 129.6, 155.1, 156.8, 159.4, 161.4, 171.9, 179.1. HRMS (M+H)+ requires 353.1495 found 353.1490. Elemental analysis: C20H20N2O3 requires C, 71.41; H, 5.99; N, 8.33; found C 71.53, H 5.89, N 8.37%.
4.2.6 (Z)-3-(amino(4-methoxyphenyl)methylene)-1-(4-methoxyphenyl)-4-methylpyrrolidine-2,5-dione 4be
Yield (264 mg, 75%), white solid. M.p = 199–200 °C. IR (KBr) υmax/cm−1: 1730 (CO); 1733 (CO); 3330 (NH2), 1H NMR (CDCl3)δ: 0.88 (d, J = 7.2 Hz, 3H), 3.49 (q, J = 7.2 Hz, 1H), 3.76 (s, 3H), 3.80 (s, 3H), 4.73 (br s, 1H), 6.90 (J = 8.7 Hz, 2H), 7.11 (J = 8.7 Hz; 2H), 7.23 (J = 9.0 Hz, 2H), 7.37 (J = 9.0 Hz, 2H), 8.39 (br s, 1H). 13C{1H}NMR (CDCl3) δ: 17.0, 39.0, 55.8, 55.9, 95.4, 114.7, 114.8, 125.6, 127.8, 128.2, 128.8, 156.7, 159.4, 161.4, 171.9, 179.0. HRMS (M+H)+ requires 369.1444 found 369.1440. Elemental analysis: C20H20N2O4 requires C, 68.17; H, 5.72; N, 7.95; found C 68.13, H 5.66, N 7.88%.