1 Introduction
Composition, aetiology and consequences of microcalcifications in kidney allografts are unclear. Widely different incidences of crystal deposits have been described, ranging from 4 to 78% [1–8]. First descriptions were in favour of calcium oxalate deposits as a consequence of delayed graft function and serious tubular necrosis [1,9]. More recently, studies have pointed out the responsibility of mineral disorder present after renal transplantation in the constitution of calcium phosphate deposits [4,10]. The impact of such deposits in renal outcome is conflicting. Inconsistencies in studies are in part explained by different biopsy protocols and histological evaluation. Staining procedures give different information about crystals in renal transplant biopsies. Pathologists take advantage of Von Kossa staining to highlight the calcium deposits [11]. Note that a recent investigation has underlined some drastic limitations regarding such staining procedures [12]. The Pizzolato staining is generally used for the demonstration of calcium oxalate crystals in paraffin [13]. Polarized light permits distinction between calcium phosphate and calcium oxalate crystals [6]. But none of these techniques are able to distinguish the chemical nature in different phases as spectrometry can. Infrared microspectroscopy (IR-MS) is now available to study accurately crystals in human tissue [14–16]. This technique permits to screen the presence of microcalcifications in a sample biopsy and to determine their chemical compounds [17]. The present study aims to illustrate the contribution of IR-MS in (a) the physicochemical characterisation of crystal deposits, (b) the understanding of the circumstances of such depositions, and (c) the outcomes of allografts with microcalcifications.
2 Materials and methods
2.1 Study population
We performed a retrospective single-centre observational study: 118 biopsy samples were retrospectively retrieved from a key-word search of the computed database of the Department of Pathology of the University Hospital of Lille. The selection terms consisted of “calcification”, “crystal” and “graft kidney”. The selection criteria included availability of the paraffin-embedded kidney allograft biopsy tissue. These 118 biopsies were performed in 72 allograft recipients. One hundred patients without crystals in their biopsy were randomised as controls. All patients consented to participate in the protocol. Patients were treated with double immunosuppressant of calcineurin inhibitor and mycophenolate mofetil, and prednisolone was added in case of sensitisation. This study was approved by our local ethics committee. Informed consent was obtained for biopsy and for the use of clinical data and secondary use of histological materials for research.
2.2 Biopsy analysis
2.2.1 Histopathological analysis
The study population consisted of protocol biopsies performed 3 months after transplantation and biopsies for renal dysfunction. Two tissue cylinders were obtained by percutaneous renal transplant biopsy using a biopsy gun with a 16-gauge needle. One cylinder was fixed in buffered formalin and embedded in paraffin for routine light microscopic examination. Slices of biopsy samples in paraffin were routinely stained by Massons' trichrome, HES, PAS, and Jones methods. The other tissue cylinder was frozen for immunofluorescence microscopy analysis. All biopsies were evaluated according to the updated BANFF classification. In case of visualisation of crystalloid deposits during microscopy examination, biopsies were submitted to IR-MS analysis.
2.2.2 IR-MS analysis
IR-MS was performed on an IN10MX microscope (Thermo Scientific, ZA Courtaboeuf, Les Ulis, France). Tissue samples were deposited on Low-e microscope slides (MirrIR, Kevley Technologies, Tienta Sciences, Indianapolis) and then chemically treated with xylene to remove paraffin in order to improve crystal detection. All spectra were collected in ultrafast mode using a 50 μm × 50 μm aperture. The spectra were collected in the 4000–800 cm−1 mid-IR range at a resolution of 16 cm−1 with one spectrum per pixel. Data analysis of IR spectra and chemical images was performed using OMNIC software. Infrared spectroscopy was also carried out on a PerkinElmer Spotlight 400 FTIR microspectrometer fitted with a liquid nitrogen cooled, 16-element linear array mercury cadmium telluride (MCT) detector. All IR spectra were collected in the mid-infrared from 4000 cm−1 to 650 cm−1 using 16 cm−1 spectra resolution and 64 accumulations for each collection by the array. The different compounds were identified by comparing them to reference spectra [18]. Results were classified in pure or mixed chemical deposits, with an intensity range of spatial distribution in the sample tissue from 1 to 4.
2.3 Sample collection and analyses
All relevant clinical and biochemical data of both donors and recipients were collected prospectively in a database. Serum and urine sample analyses were performed at the first ambulatory consultation (day 18) and at months 3, 6, 12 and 24 after transplantation. Serum concentrations of PTH were determined by third generation chemiluminescence assay. This assay detects full-length and not the N-terminal truncated fragments with a normal range of 15–68 pg/ml.
2.4 Statistics
SPSS statistical software package, version 22.0 (SPSS Inc, Chicago, Illinois, USA), was used for the statistical analyses. Results are expressed as mean ± SD. Numerical data were compared with the student t-test or the Mann–Witney U-test, depending on the results of analysis for a normal distribution using the Shapiro–Wilk test. Comparisons of categorical data between groups were performed with the Chi-square test or the Fischer exact-test as indicated. Graft survival Kaplan Meier-curves were compared with the log-rank test. Differences with p < 0.05 were considered as being statistically significant.
3 Results
3.1 IR-MS
118 biopsies from 72 patients were analysed by IR-MS (Fig. 1). Biopsies were performed in the first two years of transplantation in 88 (74.5%) cases, between 2 and 5 years in 19 (16.1%) cases and after 5 years in 11 (9.3%) cases. In 3 biopsies, deposits visualized in optic microscopy were not found in IR-MS. IR results are listed in Table 1. Microcalcifications had mixed constitution in 54%. Calcium phosphate deposits were present in 92% of all biopsies. Only 6 biopsies were calcium phosphate free: one with 2,8-dihydroxyadenine (DHA) crystals in an adenine phosphoribosyltransferase (APRT) deficiency transplanted recipient [19], 3 with Whewellite (WW), and 2 with undetermined deposits. The calcifications were considered moderate in 80% (score 1–2) and high in 20% (score 3–4).
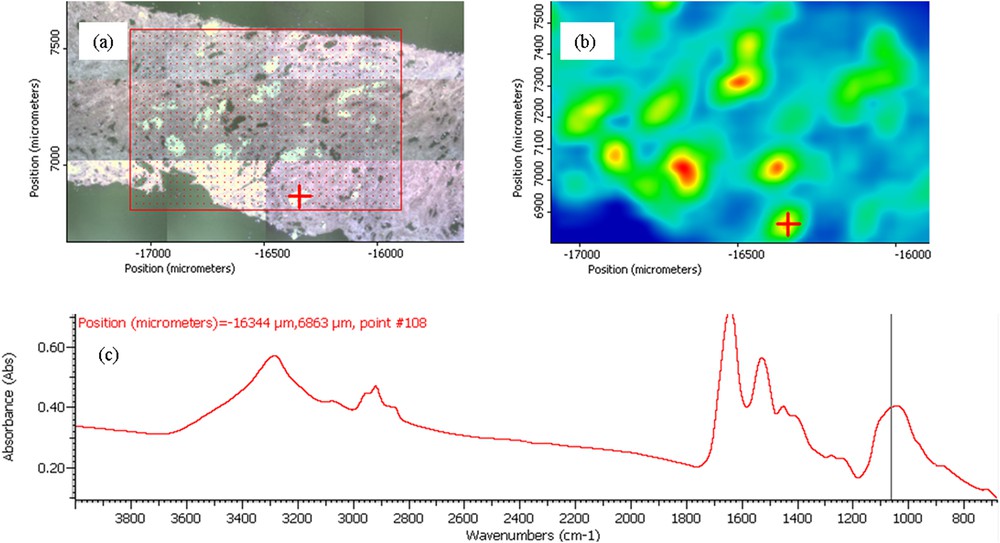
(a) Optical micrograph image of the allograft kidney biopsy. (b) Spatial distribution of crystals obtained from FTIR spectra. (c) The FTIR spectrum of a calcium phosphate deposit made of a mixture of amorphous carbonated calcium phosphate and carbapatite. The red cross in (a) and (b) indicates the position where the infrared spectrum shown in (c) was recorded.
Chemical composition of crystals as given by IR-MS. CA = carbonated calcium apatite; ACCP = amorphous carbonated calcium phosphate; WW = Whewellite; X = undetermined; DHA = Dihydroxy adenine; MPS = mucopolysacharides; OCP = Octacalcium phosphate.
Composition | Pure | Mixed | Total |
CA | 25 (21.7%) | 54 (46.9%) | 79 (68.7%) |
ACCP | 22 (19.3%) | 57 (49.5%) | 79 (68.7%) |
WW | 3 (2.6%) | 10 (8.7%) | 13 (11.3%) |
X | 2 (2.6%) | 3 (1.7%) | 5 (4.3%) |
DHA | 1 (0.8%) | 0 | 1 (0.8%) |
Calcite | 0 | 3 (2.6%) | 3 (2.6%) |
OCP | 0 | 1 (0.8%) | 1 (0.8%) |
MPS | 0 | 10 (8.7%) | 10 (8.7%) |
TOTAL | 53 (46%) | 62 (54%) | 115 (100%) |
3.2 Case-control study
Among the 72 patients with allograft calcification there were 3 children and 69 adults. Crystals were found in biopsies performed within the first 2 years of transplantation in 55 out of 69 adult patients. Consequently, we compared these 55 adult patients with microcalcifications (C+) to 100 patients without microcalcifications (C−) in order to identify the determinants of allograft crystal deposits during the 2 years following transplantation. Microcalcifications were calcium phosphates in 50 patients (CA 27 (49%), and ACCP 23 (42%)). In three patients (5.5%) calcifications were Whewellite and DHA in one (1.8%). The intensity score of crystal deposits was considered moderate (1–2) for 45 (82%) patients and high (3–4) for 10 (18%) patients.
3.2.1 Clinical values
No difference was found between the two populations for age, sex, body mass index, nephropathy, type of dialysis, parathyroid hormone concentration before transplantation, cold ischemia time and donor features. Immunosuppression was the same in the 2 groups mostly based on the association of Tacrolimus and Mycophenolat Mofetil with equivalent decreasing dosages during the follow up (data not shown). Compared to the control group, transplanted patients with calcification within grafts experienced similar delayed graft function (C+ 40%; C− 29% p = 0.2), pyelonephritis (C+ 9.3%; C− 11%), and acute rejection (C+ 11%; C− 13%).
3.2.2 Biopsy findings
Biopsies were performed at 5.2 ± 6 months and 6.9 ± 7 months after transplantation respectively in C− and C+ groups. Half of biopsies in group C− and 55% in group C+ were done for renal dysfunction. Using optical examination acute tubular necrosis was similar in group C+ and group C− (23.6% versus 20.3%, respectively). Tubular epithelial cell vacuolization was detected more often in case of microcalcifications (C+ 58% versus C− 37%; p = 0.004). We also found more thrombotic microangiopathy lesions in the group with microcalcifications (C+ 16% versus C− 5%; p = 0.004).
3.2.3 Biological values
Biological parameters and graft function were compared between patients C+ and C− at the first consultation (18±7 days) and at months 3, 6, 12 and 24. Prevalence of hypercalcemia (total Ca >105 mg/l) at the first consultation was more frequent in group C+ (C+ 14% versus C− 4%; p = 0.02) with higher serum PTH levels during the first year (Fig. 2). Serum phosphorus and 25-OH vitamin D did not differ between patients with or without calcification. The bone remodelling was also higher in the group with calcification: crosslaps were higher at the beginning of the transplantation (12,000 pmol/l) and until 3 months (11,000 pmol/l) than in the control group (9000 and 8000 pmol/l; p = 0.006 and 0.008). The osteocalcin rate was also higher in group C+ at 3 months (C+ 70 versus C− 50 ng/ml; p = 0.004) and 6 months (C+ 85 versus C− 54 ng/ml; p = 0.02). We did not observe any differences between the two groups for bone specific alkaline phosphatase. Serum creatinine and the estimated glomerular filtration rate (eGFR) did not differ between patients with and without calcification. The variation between eGFRs within the third month after transplantation and the one-year value was also calculated with identical results for the two groups. But there was a trend to a greater increase in eGFRs for patients without calcification (C− 3 ± 13 ml/min; C+ −1.6 ± 12.9 ml/min; p = 0.5), suggesting that calcification could have a negative effect on the outcome but only for some patients.
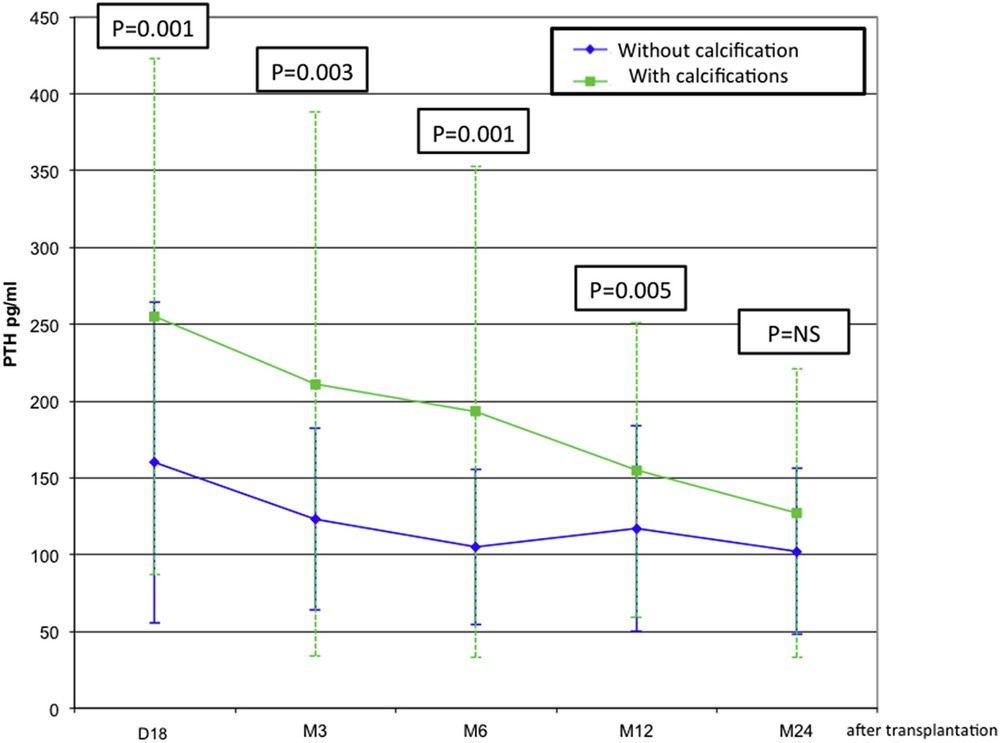
Comparison of serum PTH levels between patients with and without calcification at first consultation (day 18), 3, 6, 12 and 24 months after transplantation.
3.2.4 Allograft outcome
Using the Kaplan–Meier method, the 75-month graft survival (Fig. 3) rates were significantly lower in patients with marked calcifications (score 3–4) than in patients with moderate (score 1–2) or without calcifications (p = 0.04).
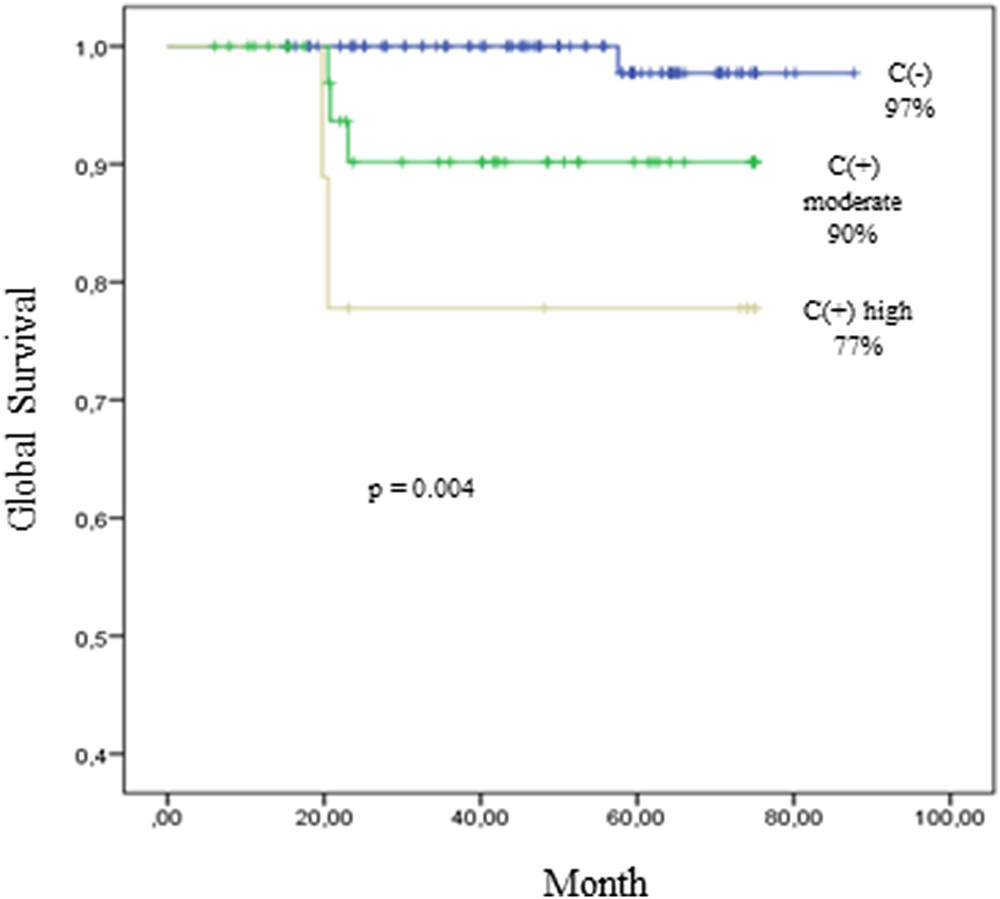
These Kaplan–Meier survival curves illustrate graft survival of 100 patients without calcification (C−) in kidney allograft biopsy. C+ with moderate score of calcification (n = 40 patients); C+ with an high score of calcification (n = 10 patients).
4 Discussion
Physicochemical characterisation of crystal deposits in kidney allograft biopsy samples by IR-MS gives important information to understand their pathogenesis. In exceptional cases, the FTIR spectra can show 2,8-dihydroxyadenine crystals and restore the diagnosis of APRT deficiency in transplanted recipients [20]. The minority of the calcium oxalate component in the crystals of our patients suggests that oxalate disorders, such as an excess of plasma oxalate at the time of transplantation [21] or enteric hyperoxaluria due to antibiotic use [22], have limited impact on crystal deposition. In contrast, the overwhelming presence of calcium phosphate crystal deposits in our biopsies (92%) underlines the key role of mineral metabolism disorder.
Hypercalcemia, hypophosphatemia and renal phosphate wasting are commonly encountered early after kidney transplantation. They are related to hyperparathyroidism and high levels of FGF-23 [23,24]. As a result of hypercalcemia and hyperphosphatoninism, calciuria and phosphaturia concentrations could increase along the nephron and the luminal calcium phosphate product could exceed the limit of solubility leading to crystal precipitation. Animal models strengthen this hypothesis [25] but clinical human data are more conflicting. In our case-control study, hypercalcemia with a higher serum PTH level is more frequent in group C+ than C−. Both Evenepoel and Gwinner found higher levels of calcemia, PTH and bone alkaline phosphatases in patients with than without graft calcification [2,4]. Moreover, the level of calciuria was reported to be higher in patients with calcifications compared to patients without calcifications, though urine calcium concentrations were lower than levels usually found in urine of stone formers [26]. Evenepoel et al. reported lower phosphate plasma levels with more frequent oral phosphate supplement therapy. But Gwinner failed to find an association between graft calcification and the serum phosphorus level. Daily phosphaturia (mg/d) was similar in the two groups with slight differences in urinary concentration (mg/l) and fractional excretion of phosphate, which were faintly higher in the group with calcifications [4]. These observations point the fact that other parameters than calciuria and phosphaturia are needed for crystal formation. Urinary pH and concentrations of inhibitors such as citrate, magnesium, pyrophosphate and urinary proteins could determine crystal formation. Note that hypocitraturia is common after kidney transplantation in part caused by acidosis or proximal tubular dysfunction by the calcineurin inhibitor [21]. However Evenepeol et al. reported low urinary magnesium and citrate concentrations in a similar range both in patients with and without calcification [2]. Kidney graft tubular damage could also promote crystal formation and deposits. Tubular dysfunction can facilitate acidosis, hypocitraturia, and a lack of local inhibitor production [27–30].
Acute tubular necrosis (ATN) may induce an up regulation of crystal binding molecules (such as osteopontin, hyaluronic acid⋯) [31,32] and membranous cellular debris in the nephron lumen may provide a matrix for crystal growth and aggregation [33]. Very little clinical data support this hypothesis. Boom et al. reported calcification one week after transplantation in 27% patients with and 11% patients without delayed graft function (DGF), suggesting that ATN would lead to calcification [9]. For Pinheiro et al., ATN is present in 47% of patients with microcalcification versus 24% without calcium deposits [1]. Nevertheless, in agreement with Evenepoel et al., we did not show difference in renal function between patients with and without calcifications, though microscopic analysis detect more often tubular epithelial cell vacuolization and thrombotic microangiopathy in case of microcalcification. These lesions could be related to renal toxicity of the calcineurin inhibitor. Moreover, Evenepoel et al. observed that acute graft rejection was associated to calcification occurrence. This was not supported by our result and Bagnasco's study [6]. Acute rejection could involve different parts of kidney parenchyma, not only tubules but also interstitium, capillary, glomeruli or vessels. Also rejection episode can occur without any tubular dysfunction. These particularities probably need to distinguish the patterns of rejection from other circumstances of microcalcification discovery.
It is difficult to establish a cause-and-effect relationship between tubular cells injuries and crystals deposits as they could be either a cause or a consequence. Besides obstructive nephron damage, crystals are directly implicated in tubular cells injury. They can promote tubular cells death by oxygen-free radicals-mediated necrosis or apoptosis [31,32]. Crystals indeed induce inflammation and a pro-fibrotic factor secretion and by this way may contribute to chronic allograft nephropathy [34].
To date clinical evidence between microcalcification and poor long-term allograft survival are lacking. Bagnasco et al. demonstrated a worst allograft outcome in the case of microcalcification at one year but not at 2 years of follow-up [6]. Pinheiro et al. reported a better 12-year survival allograft rate in the absence of microcalcification [1]. These two studies analyzed only biopsies performed for renal dysfunction. In a protocol biopsy program Schwarz et al. identified microcalcification detection at 12 weeks as an independent predictor for chronic allograft nephropathy at 26 weeks [35]. But Evenepoel et al. found a similar renal function after a mean follow-up of 33 months in patients with and without calcification during their protocol biopsy program. Explanations for these controversial results could be the different chemical nature and intensity of microcalcification deposits. IR-MS permits an accurate characterization of microcalcifications. Our work demonstrated an association between intensity of microcalcification deposits and graft outcome. Patients with marked calcifications have worsened outcome (77%) than patients with moderate (90%) or without calcifications (97%) at 75 months (p = 0.04). IR-MS allowed us to identify grafts with microcalcifications and worst outcome and consequently patients who need therapeutic intervention. Additional studies with larger numbers of patients and longer follow-up are required to settle this promising issue.
5 Conclusion
IR-MS is a high-performance tool for detection and identification of crystal deposits within allograft kidney biopsies. In our population, most microcalcifications (92%) contain calcium phosphate deposits. These deposits are associated with a higher post-graft parathyroid hormone concentration and increased tubular epithelial cell microvacuolisations, thus suggesting that hyperparathyroidism and epithelial cell damage are required for calcium phosphate crystal occurrence. Moreover, IR-MS enables a quantification of crystal deposits. The burden of crystal deposits within biopsies (quantified by IR-MS) seems to be correlated with the allograft outcome. Though our findings require validation by a large prospective study, IR-MS analysis represents an interesting tool to detect and identify crystals within kidney allograft biopsies in order to target patients requiring specific therapies acting on mineral homeostasis.