1 Introduction
Glycoside nucleoside lipids (or glycoside nucleolipids), also abbreviated as GNLs, are bioinspired molecules that belong to the large family of glycolipids. These compounds feature saccharides or oligosaccharides linked to a lipid moiety, either directly via the anomeric oxygen, or indirectly via a spacer unit such as glycerol (glycoglycerolipids), or sphingosine (glycosphingosines), for example. In the case of GNLs, a nucleoside is inserted between the lipid and the carbohydrate moiety (Fig. 1). Glycolipids are ubiquitous components of the living world in which they play important biological functions [1]. Recently, these compounds have been the object of increasing interest after the discovery of glycosphingolipids derived from a marine origin [2], which were able to activate natural killer T cells [3]. In this family, one of the most extensively studied glycoconjugate has been the α-GalCer – or KRN 7000 [4] – which is known for its antitumor effect on hepatic metastases [5]. Hence, many research groups are currently working on new KRN 7000 analogs [6]. From a biological point of view, carbohydrates and lipids are of primary importance: the former are involved in cell surface recognition while the latter constitute the cell membrane framework. Beside their biological properties, the combination of a polar carbohydrate moiety with a lipophilic part gives a natural amphiphilic character to the molecule. This characteristic, which induces the formation of self-assemblies, is also an important feature of GNLs. Glycolipids and glycosyl nucleolipids share partly the same structural features. Nevertheless, owning to the presence of the nucleoside, the glycosyl nucleolipids present additional H-bonding and π-stacking capabilities [7], which offer a new dimension for supramolecular chemistry. Indeed, these additional interactions allow the stabilization of new self-assembly morphologies, including nanofibers or nanotubes [19]. The aim of this article is to highlight some recent results obtained in our research group with the properties and biotechnological applications of GNLs, how they can be related to the other glycolipids, and how they may differ from them.
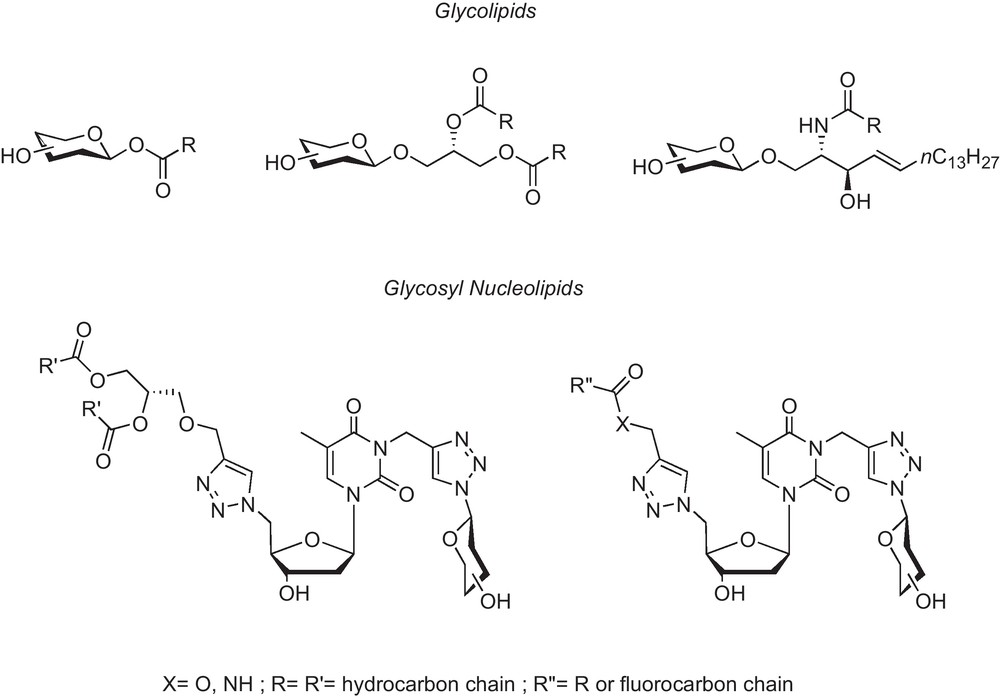
Glycolipids (from left to right: acyl, glycerol and sphingosine derived GLs) vs. Glycosyl-nucleoside-lipids (GNLs).
2 From natural nucleoside-based amphiphiles to synthetic GNLs
When the polar moiety is either a nucleobase, a nucleoside, a nucleotide or an oligonucleotide, then the resulting conjugate is called a nucleolipid. Several nucleolipids can be found in natural sources [8]. Among others, cytidine diphosphate diacylglycerol 1, for example (Fig. 2), present in mammalian cells [9], is involved in the formation of membrane cellular components, whereas the uracil nucleoside 2, produced by Streptomyces griseosporeus is an antibiotic [10].
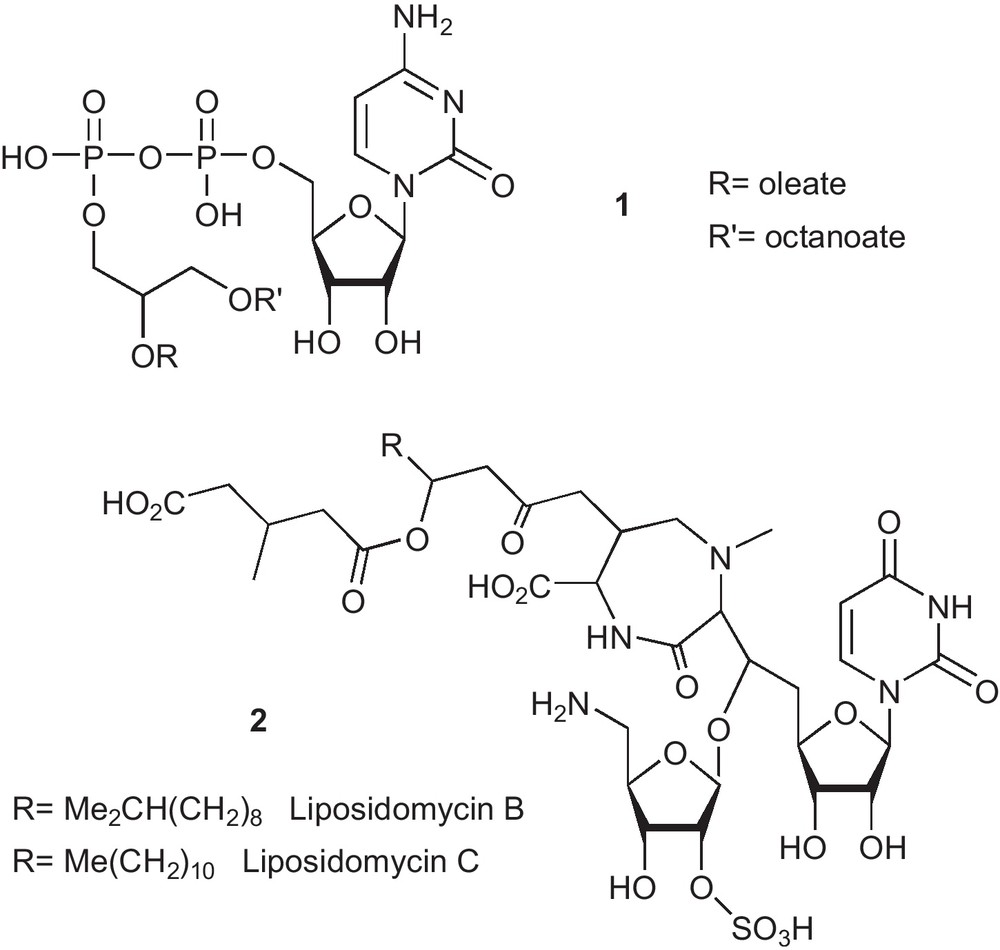
Example of some known natural nucleolipids.
Therefore, nucleolipid analogs have been of course investigated for therapeutic purposes. This can be illustrated by the synthesis of DOTAU and O-Et-DOUPC in our group as transfecting agents (Fig. 3) [11]. The latter compound, for example, proved its efficacy in CHO cells using a reporter β-gal gene assay.
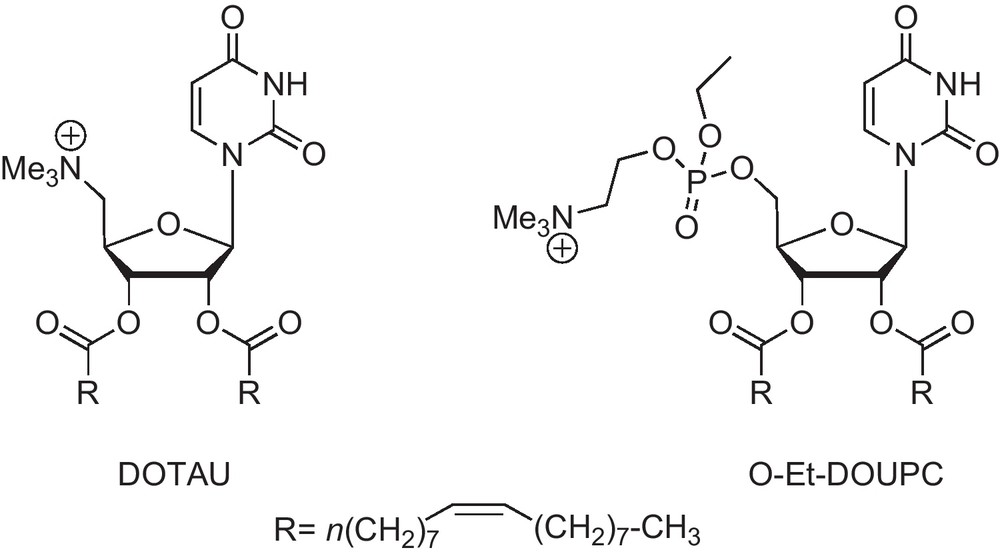
Uridine lipids used as transfecting agents.
Another recent example is the synthesis of squalene-conjugated nucleoside analogues SQddC-MP and SQdFdC-MP [12] (Fig. 4). Dideoxycitidine monophosphate and gemcitabine monophosphate once linked to squalene (a natural lipid) self-assemble into nanoparticules in water, showing significant anti-HIV and anticancer activity on leukemia cells respectively.

Structures of 4-(N)-trisnor-squalenoyldideoxycitidine monophosphate (SQddC-MP) and 4-(N)-trisnor-squalenoylgemcitabine monophosphate (SQdFdC-MP).
In nature, the delivery of nucleic acids into cells is clearly the most interesting application for nucleolipids. Interaction with cellular DNA usually relies on electrostatic interactions between the polyanionic DNA and cationic lipids, as shown by DOTAU, O-Et-DOUPC for example. Nevertheless, neutral nucleolipids can serve this purpose too, as illustrated with the compound in Fig. 5, which compensates for its lack of neat negative charge by an additional polar moiety, namely a glycoside moiety [13] and this is basically the first example of glycosyl nucleolipid synthesized in our group.
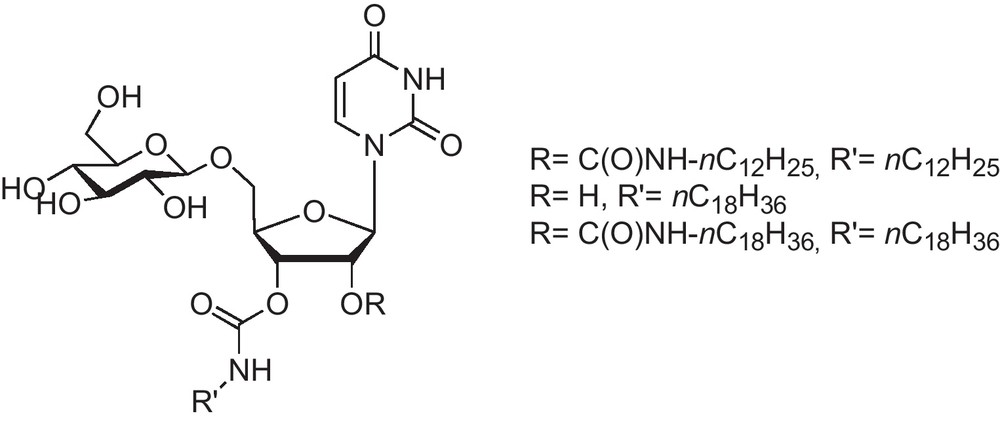
First generation of GNL. Glycosyl moiety is attached to the 5′ position of d-ribose.
However, assembling three biomolecular moieties into a single one needs several cumbersome protection and deprotection steps and we wanted to develop a more straightforward synthesis of such neutral nucleolipids featuring a carbohydrate moiety. This was made possible by using the efficient methodology provided by click-chemistry [14]. One of the most popular click reaction is the Huisgen copper catalyzed 1,3-dipolar addition of an azide and a terminal alkyne to create a triazole linkage. It provides numerous advantages over more traditionnal synthesis in the present case. To mention a few:
- • alkyne and azide starting materials are easily obtained;
- • the reaction is irreversible;
- • the reaction can take place in water, therefore masking all protic functional groups. This leads to a very high selectivity and avoids needing to protect the carbohydrate moiety, for example;
- • triazole is almost isosteric with the ubiquitous amide moiety found in nature, but it is also much more chemically resistant. Additionally, triazole may give rise to intermolecular π–π stacking interactions [19].
More particularly, in order to easily connect the three natural building blocks, we used a “double-click” chemistry approach (Scheme 1): a first click reaction connects the azido nucleic acid to an acetylenic lipid, then a second one connects an azido sugar to the propargylated nucleobase.
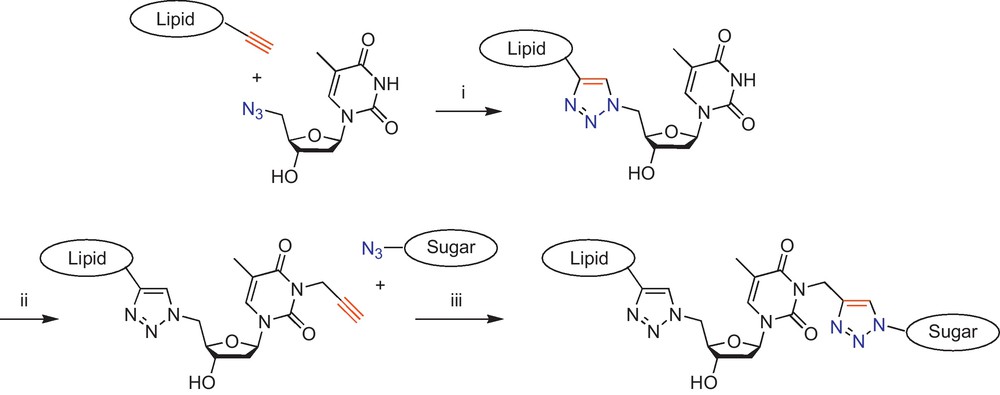
(i) First click reaction; (ii) 1 equiv of propargyl bromide, 1.5 equiv of K2CO3, DMF, rt, 12 h; (iii) Second click reaction [19].
So far, we have synthesized numerous thymidine GNLs with various lipidic and carbohydrate moieties (Table 1).
Detailed lipid and carbohydrate structures used in thymidine GNL synthesis.
Lipidic moieties |
Carbohydrate moieties |
1-Azido-1-deoxy-β-d-glucopyranoside |
1-Azido-1-deoxy-β-d-galactopyranoside |
1-Azido-1-deoxy lactose |
As an example, the detailed synthesis of two glycerol derived GNLs is depicted in Scheme 2.

(i). NaH/toluene, propargyl bromide, 80 °C; (ii) Dowex 50 X2, MeOH r.t.; (iii) stearic or oleic acid, DCC, DMAP/CH2Cl2 r.t.; (iv) 5’-azido-5’-deoxythymidine, sodium ascorbate, CuSO4, THF/H2O 60 °C; (v) K2CO3, propargyl bromide/DMF r.t.; (vi) 1-azido-1-deoxy-β-d-glucopyranoside, sodium ascorbate, CuSO4, THF/H2O 60 °C [22].
Commercial sn-protected glycerol was propargylated using propargyl bromide and sodium hydride in toluene to give the acetylenic protected glycerol, which was further deprotected using a Dowex acidic resin. The crude material was reacted with stearic or oleic acid in the presence of DCC/DMAP. The alkyne lipidic moiety was then subjected to a first click reaction with 5′-azido-5′-deoxythymidine [15] to give the triazolyl nucleoside-lipid intermediate. The heterocycle was propargylated on its N-3 position [16] and the resulting compound was subjected to the second click reaction with commercial 1-azido-1-deoxy-β-d-glucopyranoside to give the desired GNLs.
3 GNLs’ supramolecular assemblies
Amphiphilic molecules share similar structural features like the combination of a hydrophobic tail and a hydrophilic head group, giving rise to their well-known surfactant properties and also their ability to self-assemble into a wide variety of nanostructures [17].
Compared to common amphiphilic structures such as for example phospholipids or glycerophospholipids, nucleolipids possess a highly informative polar head, thanks to the nucleobase, allowing further intermolecular interactions. Finally, glycosyl nucleolipids with their added polar carbohydrate and triazolyl moieties give rise to three types of interactions (Fig. 6).
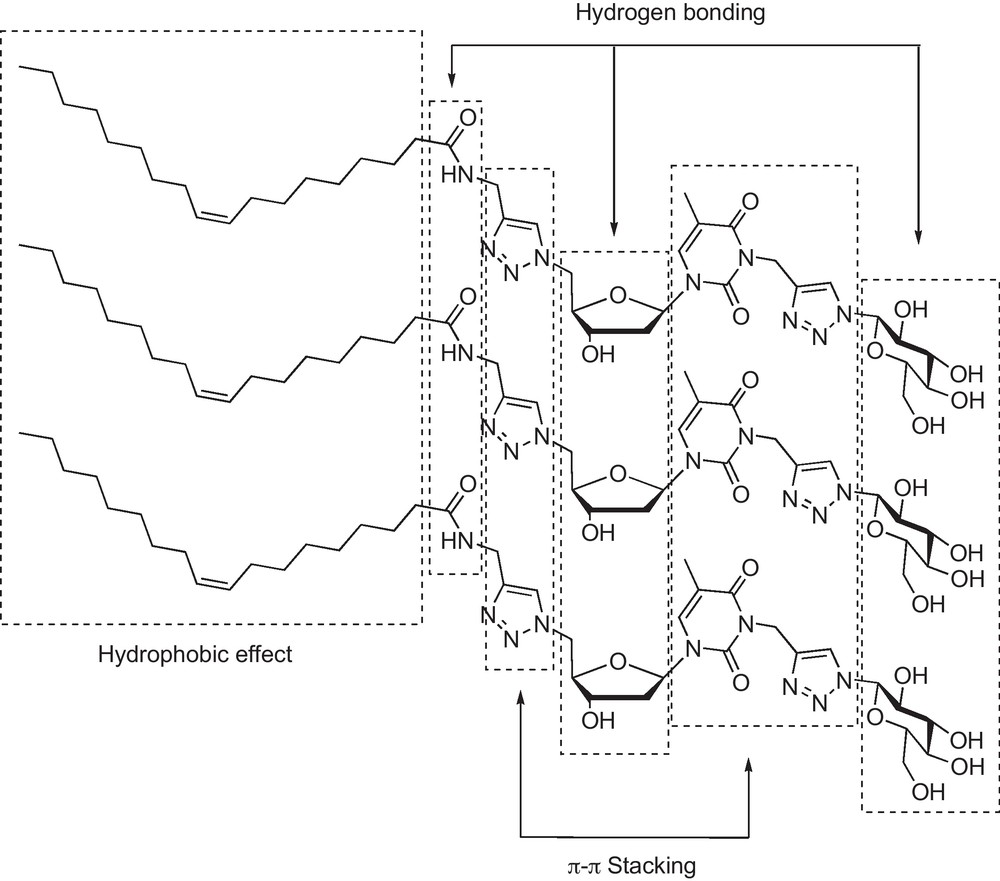
The various types of intermolecular interactions exhibited by GNLs.
Indeed, hydrophobic effects between the hydrocarbon chains were evidenced by pyrene inclusion, hydrogen bonding from polar groups by IR studies and π-π stacking by UV–vis experiments [18]. Therefore, as other amphiphiles, GNLs are expected to form structured self-assembly materials, and we were pleased to observe for most of them the formation of organo- and hydrogels at very low concentrations (up to 0.1% w/w in water) and with very diverse nanostructures (nanofibers, circularly organized fibers, and semitubular objects were observed through transmission electronic microscopy) [18,19]. As previously underlined [18], the three moieties (namely lipid, nucleobase and carbohydrate) are necessary to form a gel, but this prerequisite is not a sufficient condition, since both stearyl glycerol and oleyl glycerol derived GNLs did not stabilize a hydrogel (within a 0.1 to 6% w/w concentration range) and were found totally soluble, on the other hand, in most of the organic solvents tested.
4 GNLs’ cytotoxicity
Previous results have demonstrated the relative absence of toxicity of a fluorinated GNL (with a nC8F17(CH2)2C(O)NH chain) compared to its hydrocarbon counterpart after a 5-day incubation of growing human hepatocarcinoma (Huh) -7 cell line [19]. This prompted us to assess the cytotoxicity of the hydrogel formed by this fluorinated GNL, with the objective in the near future to use our hydrogels as scaffolds for stem cell culture and delivery systems [20]. Adipose derived stem cells (ADSCs) were used for this purpose with a hydrogel formulation of 1.5%. Both MTT and neutral red assays did not reveal any significant cytotoxicity after 72 hours (Fig. 7).
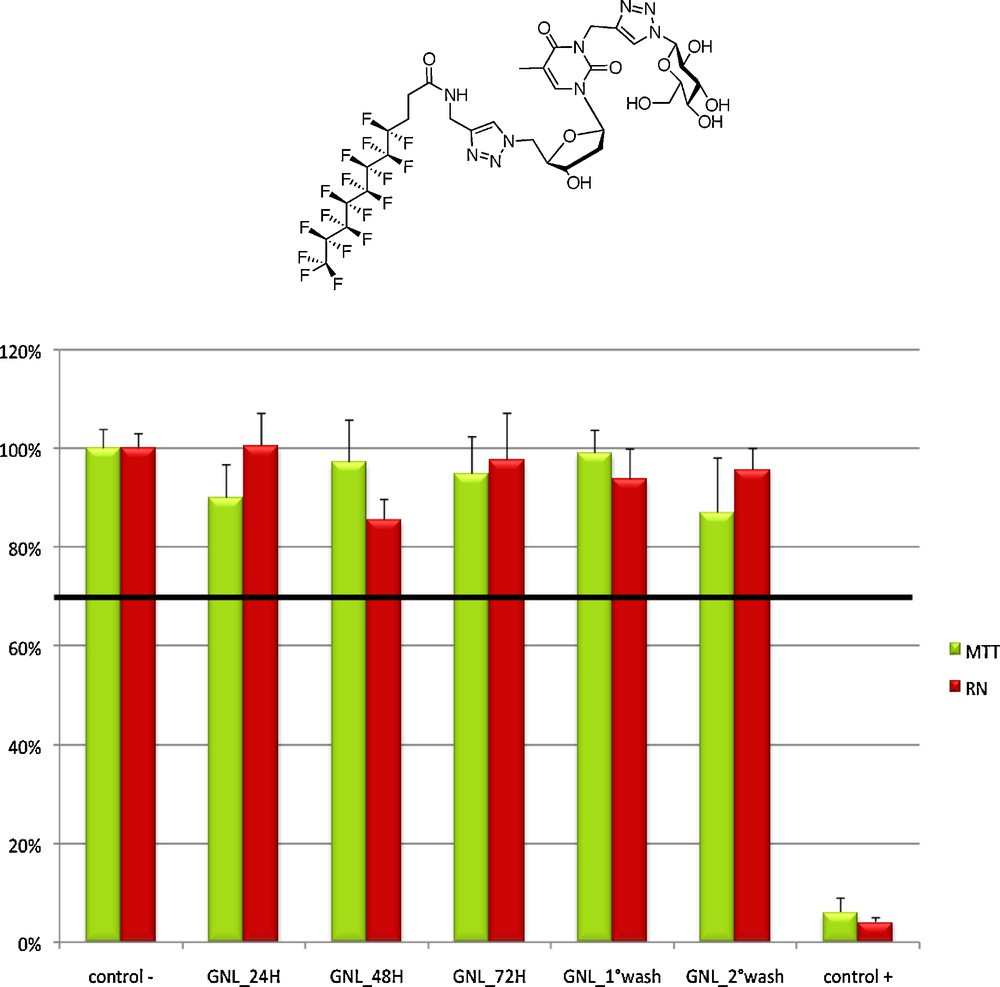
F-GNL cytotoxicity assay. Cell viability remains far above the 70% threshold even after 72 h.
ADSCs were cultivated for 2 weeks on a polystyrene culture dish (which acted as a control) and at the surface of F-GNL at 1 and 0.5% (2D experiment, Fig. 8). On polystyrene, isolated cells spread and colonized the whole culture dish as expected. On the contrary, cells cultivated on the hydrogel exhibited an unusual round shape since D1, and after 9 days, cell mortality became very important. Furthermore, cells alive on the 0.5% F-GNL gel exhibited a very different morphology at D14.

2D cell culture on polystyrene and on F-GNL hydrogels at 1 and 0.5%. Cells on the F-GNL show a different morphology after 14 days.
In another and even more differentiating assessment of the viability of cell culture with F—GNL, cells were mixed during the hydrogel formulation and then dispersed throughout the hydrogel volume, a process we called a three-dimensional culture, but almost all isolated cells were found dead at D14. It was assumed that cell death resulted from lack of cell adhesion through integrin receptors. As an alternative, cells were allowed to self assemble on ultra low attachment plastic dishes. In these conditions, spheroids containing around 2000 cells were obtained. These spheroids had an excellent viability in the hydrogels (Fig. 9).

3D spheroids cell culture. Live-dead assay confirmed the spheroids viability after 2 weeks.
5 GNLs based liposomes
For years, liposomes have found numerous applications ranging from fundamental research, to novel drug delivery systems, and more recently to gene delivery [21]. Owing to the amphiphilic nature of GNLs, we wanted to examine their ability to be incorporated in colloidal formulations. Hence, stearyl glycerol derived GNL was used either alone or mixed to various amounts (0, 10, 20 and 100%) of soya lecithin. The crude vesicles were extruded at 200 nm to give objects ranging from 74 to 150 nm in average size for liposomes made of pure GNLs and pure phospholipids respectively, as evidenced by DLS measurements and TEM images. Encouraging results were subsequently obtained when liposomes were incubated with adipose derived stem cells (ADSCs) since we observed that only the GNL-containing liposomes (L2, L2 and L4) were able to interact with cells (Fig. 10) [22].
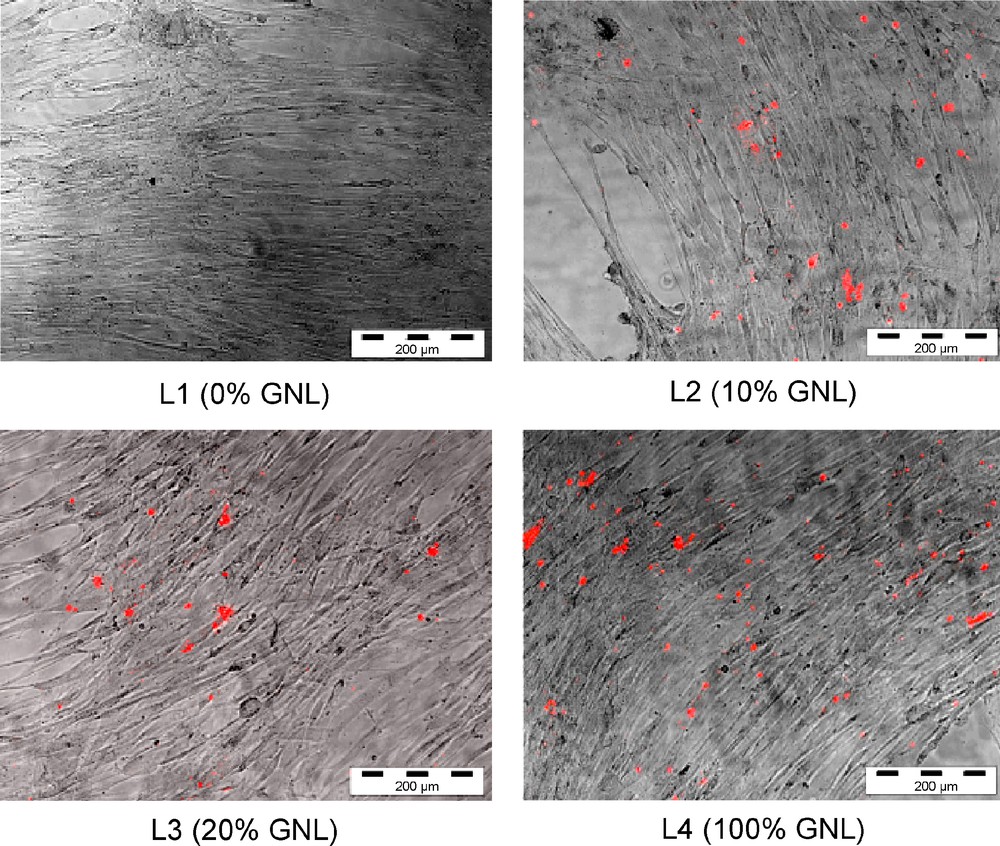
Different liposomes formulations (L1 to L4) incubated with ADSCs for 48 h. A red lipophilic carbocyanine dye was used for each liposome formulation to reveal cell fixation.
6 Conclusion
While some nucleolipids (NLs) and many glycolipids (GLs) are naturally occuring molecules, GNLs are bioinspired artificial conjugates, which differ from both NLs and GLs in their molecular arrangement. Similar physicochemical characteristics may be expected from all these amphiphilic compounds, but hydro- and organogel formation at very low concentration seems to be a unique feature exhibited by GNLs. As both nucleo- and glycolipids are currently assessed for biomedical applications, transfection capability, etc., all remains to be done in this domain with GNLs. Obviously, their biological interest is closely related to their gelling properties with potential applications in tissue engineering and local drug delivery. Nevertheless, our recent works have demonstrated that GNLs can be easily synthesized using an original double-click chemistry approach. Importantly, these GNLs possess unique gelation properties and supramolecular capabilities. In fine, GNLs are non-cytotoxic bioinspired compounds, which are currently evaluated as transfecting agents in their liposome form and as scaffolds for stem cell culture.
Acknowledgements
We acknowledge financial support from the US Army Research Office, the Région d’Aquitaine and the Agence nationale pour la recherche (GelCells, ANR-10-BLANC-0728).