1 Introduction
Nowadays, considerable attention has been paid to the design of new, efficient and environmentally benign synthetic strategies to reduce the amount of toxic waste and by-products in chemical manufacture [1]. One of the factors that contribute significantly to the environmental pollution is the use of large quantities of toxic solvents for the extraction and chromatographic purification of the target molecules. Hence, optimization of the reaction conditions to afford pure products by eliminating solvent extraction and purification steps is a major challenge in organic synthesis [2].
In recent years, the construction of a xanthene scaffold has attracted great interest due to its wide range of applications in the field of medicinal and materials chemistry [3]. In particular, 9-substituted-2,3,4,9-tetrahydro-1H-xanthen-1-one derivatives have been identified as an orally active neuropeptide Y Y5 receptor antagonist [4]. However, methods remain limited for the synthesis of this class of xanthene analogues. According to the literature, they can be prepared by reacting 1,3-cyclohexanediones either with substituted salicylaldehydes in the presence of AcOH–H2O [4], TEBA [5], CeCl3·7H2O [6], cellulose sulphuric acid [7], cyanuric chloride [8] and heteropolyacids [9] as catalysts, or with 3-substituted-2-(trimethylsilyl)phenyltrifluoromethane sulfonates in DMF containing catalytic amounts of tetra-n-butylammonium fluoride [10]. In addition, the catalyst-free synthesis of this heterocyclic nucleus has also been described by using water [11], glycerol [12] and DMF [13] as solvents. Unfortunately, some of these protocols suffer from severe drawbacks, including high catalyst load, prolonged reaction times, elevated temperatures, poor yields, tedious workup procedure or required extraction and chromatographic isolation techniques. Consequently, the development of an efficient, operationally simple, eco-friendly and practical method for the synthesis of 9-substituted-2,3,4,9-tetrahydro-1H-xanthen-1-one derivatives under mild reaction conditions is in high demand.
Recently, l-proline has emerged as an efficient, cheap, versatile, environmentally benign and metal-free organocatalyst for the formation of carboncarbon bonds [14]. It has been found very effective in accelerating the reaction rate by forming either the iminium ion or enaminone as intermediates [15]. To this end, it was expected that l-proline might catalyse the reaction of cyclic-1,3-diones with salicylaldehydes by forming enaminone and iminium ion intermediates. Therefore, we envisaged to use the catalytic amount of l-proline for the synthesis of 9-substituted-2,3,4,9-tetrahydro-1H-xanthen-1-ones in high yields.
As a consequence of our interest in developing new efficient synthetic methodologies [16] for the synthesis of bioactive molecules and owing to the biological significance of xanthene derivatives, we wish to report herein a l-proline-accelerated, mild, operationally simple, economical and environmentally benign protocol for the synthesis of various 9-substituted-2,3,4,9-tetrahydro-1H-xanthen-1-one derivatives at 60 °C in ethanol as a solvent (Scheme 1). It is important to note that the title compounds were obtained in pure form just by filtration of the reaction mixture, avoiding the solvent extraction or tedious column chromatographic purification steps. This simple workup procedure magnifies the attractiveness of the present protocol and affords the target xanthene compounds in good to excellent yields.
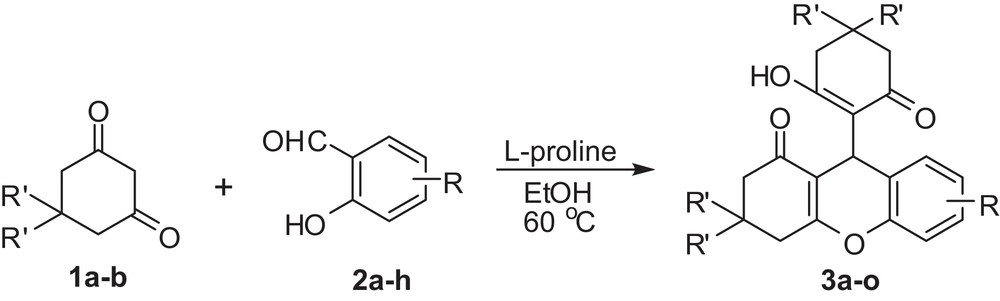
l-proline accelerated synthesis of 9-substituted-2,3,4,9-tetrahydro-1H-xanthen-1-ones under mild conditions.
2 Results and discussion
Recently, one of the research groups has reported the formation of 9-(2-hydroxy-4,4-dimethyl-6-oxocyclohex-1-enyl)-3,3-dimethyl-2,3,4,9-tetrahydro-1H-xanthen-1-one as a by-product during the synthesis of 4H-chromenes by a three-component reaction of salicylaldehydes, 1,3-cyclohexanediones and a sulphur, carbon, or nitrogen-based nucleophile in the presence of 10 mol% of l-proline at 80 °C [17]. In addition, various xanthen-1,8-diones were synthesized by reacting aldehydes with dimedone in the presence of Zn[(l)proline]2 in water at a reflux temperature [18]. However, our synthetic protocol has proven efficient in the synthesis of a series of 9-substituted-2,3,4,9-tetrahydro-1H-xanthen-1-one derivatives by using only 5 mol% of l-proline as an organocatalyst under mild conditions.
We chose dimedone and salicylaldehyde as model substrates for the optimization of the reaction conditions. Initially, the reaction of model compounds was carried out in the presence of 10 mol% of l-proline at 80 °C under solvent-free conditions (Table 1, entry 1). Gratifyingly, the desired product, 9-(2-hydroxy-4,4-dimethyl-6-oxocyclohex-1-enyl)-3,3-dimethyl-2,3,4,9-tetrahydro-1H-xanthen-1-one (3a), was isolated as a white solid in 99% yield within 30 min. Performing the same reaction at lower temperatures, i.e. 70 °C and 60 °C, did not affect the yield of the product (Table 1, entries 2 and 3). In contrast, the reaction carried out at 50 °C and 25 °C furnished the lower yields of the desired product (3a) after 30 min and 18 h, respectively (Table 1, entries 4 and 5). Hence, 60 °C was chosen as the optimum temperature for carrying out the further reactions.
Optimization of the reaction conditions for the synthesis of 9-(2-hydroxy-4,4-dimethyl-6-oxocyclohex-1-enyl)-3,3-dimethyl-2,3,4,9-tetrahydro-1H-xanthen-1-one (3a)a.
Entry | Catalyst load (mol%) | Solvents | Temp (°C) | Time (h) | Yields (%) |
1 | 10 | – | 80 | 0.5 | 99 |
2 | 10 | – | 70 | 0.5 | 99 |
3 | 10 | – | 60 | 0.5 | 99 |
4 | 10 | – | 50 | 0.5 | 75 |
5 | 10 | – | 25 | 18 | 65 |
6 | 5 | – | 60 | 0.5 | 98 |
7 | – | – | 60 | 7 | 10 |
8 | 5 | CH3CN | 60 | 7 | 35 |
9 | 5 | PEG-400 | 60 | 7 | 40 |
10 | 5 | t-butanol | 60 | 7 | 97 |
11 | 5 | EtOH | 60 | 0.5 | 98 |
a Dimedone (2 equiv) and salicylaldehyde (1.2 equiv).
The effect of the catalyst load on the yield of the product was next examined. It was observed that the use of either 5 or 10 mol% catalyst under the same reaction conditions produced the desired compound (3a) in almost similar yields (Table 1, entries 3 and 6) and thus, 5 mol% was used as the optimum catalyst load. It should be noted that the reaction performed at 60 °C under solvent-free conditions in the absence of the catalyst resulted in only a 10% yield of the desired product after 7 h (Table 1, entry 7), demonstrating the catalytic role of l-proline in the synthesis of xanthene (3a). Further, the effect of various solvents on the yield of the product (3a) was also investigated. The solvents such as acetonitrile and PEG-400 adversely affected the rate of reaction and generated the corresponding product (3a) after 7 h in 35% and 40% yields, respectively (Table 1, entries 8 and 9). On the other hand, the reaction proceeded well by using t-butanol as a solvent medium, but it was found sluggish as compared to the reaction performed in ethanol and produced the desired product (3a) in 97% yield after 7 h (Table 1, entries 10 and 11). Notably, the reaction performed either under solvent-free conditions or in ethanol as a solvent medium afforded the desired product (3a) in 98% yield within 30 min (Table 1, entries 6 and 11). However, ethanol was selected as a solvent medium to perform the reactions as, in most of the cases, the reacting substrates were solid, making the reaction mixture heterogeneous under solvent-free conditions that adversely affect the yields of the desired products. Moreover, the reaction performed under solvent-free conditions produced mostly impure products that need to be purified either by recrystallization or column chromatography techniques. In contrast, reactions carried out in ethanol provided the desired products in pure form by direct filtration of the reaction mixture. Therefore, 5 mol% of l-proline at 60 °C temperature in ethanol was chosen as the optimum reaction conditions.
With the standardized conditions in hand, the scope and limitations of this condensation-cyclization reaction was investigated by synthesizing a series of 9-substituted-2,3,4,9-tetrahydro-1H-xanthen-1-one derivatives from the reaction of dimedone or 1,3-cyclohexanedione and various substituted salicylaldehydes in the presence of 5 mol% of l-proline at 60 °C in ethanol as a solvent medium (Table 2).
l-proline accelerated synthesis of 9-substituted-2,3,4,9-tetrahydro-1H-xanthen-1-one derivatives (3a–o)a.
Entry | R′ | R | Products | Time (h) | Yields (%) |
1 | CH3 | H | 3a | 0.5 | 98 |
2 | CH3 | 7-Cl | 3b | 0.5 | 99 |
3 | CH3 | 7-NO2 | 3c | 0.5 | 99 |
4 | CH3 | 7-OMe | 3d | 3 | 97 |
5 | CH3 | 5-OMe-7-Br | 3e | 0.5 | 98 |
6 | CH3 | 5,7-Cl2 | 3f | 1 | 94 |
7 | CH3 | 5-OH | 3g | 3.5 | 93 |
8 | CH3 | 6-OH | 3h | 5 | 85 |
9 | H | H | 3i | 2 | 95 |
10 | H | 7-Cl | 3j | 3 | 94 |
11 | H | 7-NO2 | 3k | 1 | 95 |
12 | H | 7-OMe | 3l | 4 | 91 |
13 | H | 5-OMe-7-Br | 3m | 4 | 94 |
14 | H | 5-OH | 3n | 4 | 95 |
15 | H | 6-OH | 3o | 5 | 80 |
a Reaction conditions: dimedone or 1,3-cyclohexanedione (2 equiv), substituted salicylaldehydes (1.2 equiv), l-proline (5 mol%), ethanol (3 mL), 60 °C.
A variety of salicylaldehydes carrying either electron-donating or electron-withdrawing groups such as OH, OMe, Me, Cl, NO2, Br etc. were employed to synthesize the corresponding products (3b–h and 3j–o) in good to excellent yields. Interestingly, dimedone reacted faster as compared to 1,3-cyclohexanedione and afforded the desired products comparatively in higher yields (Table 2, entries 1 and 9, 2 and 10, 3 and 11, 4 and 12). The 4-hydroxysalicylaldehyde reacted sluggishly and provided the corresponding products (3h and 3o) in good yields (Table 2, entries 8 and 15), while excellent yields were achieved either with 3-hydroxysalicylaldehyde or with substituted salicylaldehydes having chloro, nitro, methoxy and methyl substituents on the aromatic ring (Table 2, entries 2–7 and 10–14).
The structures of all synthesized compounds (3a–o) have been ascertained on the basis of IR, 1H NMR, 13C NMR and mass data. In addition, the structure of 9-(2-hydroxy-4,4-dimethyl-6-oxocyclohex-1-enyl)-3,3-dimethyl-2,3,4,9-tetrahydro-1H-xanthen-1-one (3a) was further confirmed by single-crystal X-ray analysis1 (Fig. 1).

X-ray structure of compound 3a.
3 Conclusion
In conclusion, we have developed a simple, eco-friendly and practical protocol for the synthesis of various 9-substituted-2,3,4,9-tetrahydro-1H-xanthen-1-one derivatives by reacting dimedone or 1,3-cyclohexanedione with substituted salicylaldehydes under mild reaction conditions. The present methodology afforded the desired products in good to excellent yields and offers several notable advantages over the existing methods, including the fact there is no need of solvent extraction and column chromatography steps for the isolation of the desired products.
4 Experimental
4.1 Typical experimental procedure for the synthesis of compound (3a)
A mixture of salicylaldehyde (898 μL, 8.56 mmol), dimedone (2 g, 14.26 mmol), and l-proline (41 mg, 0.356 mmol) in ethanol (3 mL) was stirred at 60 °C for 30 min. After completion of the reaction as indicated by TLC, the reaction mixture was cooled to 10 °C for 10 min. The solid obtained was filtered, washed with cold ethanol and dried under vacuum to afford the pure product (3a).
Similarly, other compounds (3b–o) were prepared and characterized. The physical and spectral data of known compounds (3a–d, 3f, 3h–k and 3o) were found in agreement with the reported data [4,5,10], while the characterization data of products (3e, 3g and 3l–n) are given below.
4.2 Characterization data of selected products
4.2.1 7-Bromo-9-(2-hydroxy-4,4-dimethyl-6-oxocyclohex-1-enyl)-5-methoxy-3,3-dimethyl-2,3,4,9-tetrahydro-1H-xanthen-1-one (3e)
White solid; mp 262 °C. IR (CHCl3) νmax: 3195, 2958, 1628, 1464, 1371, 1311, 1257, 1228, 1153, 1131, 1102, 1073, 1027, 1012, 860, 816, 831, 759, 695 cm−1; 1H NMR (400 MHz, CDCl3) δH: 10.33 (s, 1H, OH), 6.83 (d, J = 2.20 Hz, 1H, ArH), 6.70 (d, J = 2.20 Hz, 1H, ArH), 4.56 (s, 1H, CH), 3.84 (s, 3H, OCH3), 2.62 (d, J = 17.57 Hz, 1H, CH2), 2.51 (d, J = 17.57 Hz, 1H, CH2), 2.39 (d, J = 17.57 Hz, 1H, CH2), 2.32 (d, J = 16.84 Hz, 1H, CH2), 2.30 (s, 2H, CH2), 1.96 (s, 2H, CH2), 1.09 (s, 3H, CH3), 0.99 (s, 6H, 2CH3), 0.97 (s, 3H, CH3) ppm; 13C NMR (100 MHz, CDCl3) δC: 200.72, 196.64, 171.09, 168.35, 147.68, 139.79, 126.77, 122.34, 117.60, 116.43, 113.70, 110.63, 56.21, 50.57, 49.85, 43.13, 41.35, 32.24, 31.01, 29.66, 29.10, 27.62, 27.02, 26.56 ppm; HRMS (ESI) calcd for C24H28BrO5: 475.1120 [M+H]+, found: 475.1124.
4.2.2 5-Hydroxy-9-(2-hydroxy-4,4-dimethyl-6-oxocyclohex-1-enyl)-3,3-dimethyl-2,3,4,9-tetrahydro-1H-xanthen-1-one (3g)
White solid; mp 251 °C. IR (Nujol) νmax: 3630, 1618, 1571, 1463, 1377, 1310, 1281, 1233, 1187, 1148, 1125, 1062, 1027, 1013, 721 cm−1; 1H NMR (400 MHz, CDCl3) δH: 10.31 (s, 1H, OH), 6.86 (t, J = 7.32 Hz, 1H, ArH), 6.74 (d, J = 7.32 Hz, 1H, ArH), 6.51 (d, J = 6.59 Hz, 1H, ArH), 5.71 (s, 1H, OH), 4.63 (s, 1H, CH), 2.60 (d, J = 17.57 Hz, 1H, CH2), 2.48 (d, J = 17.57 Hz, 1H, CH2), 2.35 (d, J = 2.93 Hz, 2H, CH2), 2.32 (s, 2H, CH2), 1.98 (d, J = 16.11 Hz, 1H, CH2), 1.92 (d, J = 16.84 Hz, 1H, CH2), 1.11 (s, 3H, CH3), 1.01 (s, 6H, 2CH3), 0.97 (s, 3H, CH3) ppm; 13C NMR (100 MHz, CDCl3) δC: 200.97, 196.75, 170.77, 167.88, 143.17, 139.00, 124.69, 119.19, 117.83, 114.27, 111.59, 50.59, 49.89, 43.12, 41.38, 32.34, 30.91, 29.86, 29.16, 27.66, 27.14, 26.39 ppm.
4.2.3 9-(2-Hydroxy-6-oxocyclohex-1-enyl)-7-methoxy-2,3,4,9-tetrahydro-1H-xanthen-1-one (3l)
White solid; mp 186 °C. IR (CHCl3) νmax: 3420, 3007, 2949, 1614, 1495, 1425, 1382, 1289, 1228, 1200, 1138, 1037, 1003, 810, 755, 665 cm−1; 1H NMR (400 MHz, CDCl3) δH: 10.88 (s, 1H, OH), 6.93 (d, J = 8.79 Hz, 1H, ArH), 6.66 (dd, J1 = 8.79 Hz, J2 = 2.93 Hz, 1H, ArH), 6.49 (d, J = 2.93 Hz, 1H, ArH), 4.59 (s, 1H, CH), 3.70 (s, 3H, OCH3), 2.75–2.39 (m, 6H, 3CH2), 2.16–1.73 (m, 6H, 3CH2) ppm; 13C NMR (100 MHz, CDCl3) δC: 201.20, 196.91, 172.83, 171.17, 156.20, 144.95, 125.48, 119.54, 116.04, 112.66, 112.56, 111.41, 55.41, 36.85, 35.85, 29.61, 28.29, 27.89, 19.78, 19.51 ppm; HRMS (ESI) calcd for C20H21O5: 341.1389 [M+H]+, found: 341.1380.
4.2.4 7-Bromo-9-(2-hydroxy-6-oxocyclohex-1-enyl)-5-methoxy-2,3,4,9-tetrahydro-1H-xanthen-1-one (3m)
White solid; mp 231 °C. IR (CHCl3) νmax: 3198, 2946, 1606, 1574, 1483, 1416, 1376, 1278, 1228, 1192, 1139, 1106, 1082, 980, 750 cm−1; 1H NMR (400 MHz, DMSO-d6) δH: 10.61 (bs, 1H, OH), 6.99 (d, J = 2.20 Hz, 1H, ArH), 6.65 (d, J = 2.20 Hz, 1H, ArH), 5.03 (s, 1H, CH), 3.85 (s, 3H, OCH3), 2.32–2.22 (m, 6H, 3CH2), 1.96–1.69 (m, 6H, 3CH2) ppm; 13C NMR (100 MHz, DMSO-d6) δC: 195.89, 166.19, 147.71, 138.48, 128.20, 121.82, 115.25, 112.83, 111.56, 100.78, 56.04, 36.62, 27.16, 25.32, 20.38, 20.26 ppm; HRMS (ESI) calcd for C20H20BrO5: 419.0494 [M+H]+, found: 419.0468.
4.2.5 5-Hydroxy-9-(2-hydroxy-6-oxocyclohex-1-enyl)-2,3,4,9-tetrahydro-1H-xanthen-1-one (3n)
White solid; mp 242 °C. IR (Nujol) νmax: 3503, 3359, 2945, 1609, 1570, 1377, 1296, 1279, 1255, 1235, 1190, 1139, 1070, 1037 cm−1; 1H NMR (400 MHz, DMSO-d6) δH: 10.40 (bs, 1H, OH), 9.37 (s, 1H, OH), 6.72 (t, J = 8.05 Hz, 1H, ArH), 6.59 (dd, J1 = 8.05 Hz, J2 = 1.46 Hz, 1H, ArH), 6.39 (d, J = 7.32 Hz, 1H, ArH), 5.01 (s, 1H, CH), 2.49–2.48 (m, 2H, CH2), 2.28–2.12 (m, 6H, 3CH2), 1.94–1.66 (m, 4H, 2CH2) ppm; 13C NMR (100 MHz, DMSO-d6) δC: 196.24, 166.77, 144.51, 138.48, 126.67, 123.91, 118.27, 113.67, 111.84, 100.67, 36.81, 27.42, 25.51, 20.48 ppm; HRMS (ESI) calcd for C19H19O5: 327.1232 [M+H]+, found: 327.1259.
Acknowledgements
This work is supported by University of Delhi, India under the scheme to strengthen R&D Doctoral Research Programme. We are thankful to the Central Instrumentation Facility, University of Delhi and SAIF, CDRI, Lucknow, India for providing NMR and mass data, respectively. D. P. and A. P. are grateful to CSIR, New Delhi, India for providing fellowship.
1 CCDC 871881 contains the supplementary crystallographic data for the deposited structure. These data can be obtained free of charge from the Cambridge Crystallographic Data Centre via www.ccdc.cam.ac.uk/data_request/cif.