1 Introduction
Proton transfer reactions between proton donors and acceptors are of crucial importance for biological energy conversion in many biological systems [1–3]. The preparation and characterization of proton transfer compounds have been considered by chemists in recent years [4]. The successful synthesis of these systems depends on the choice of the appropriate carboxylic acid, as proton donor, and amine, as proton acceptor, which interact with each other [5]. This is because functionalized carboxylic acids and amines can enhance the intermolecular forces between the obtained cationic and anionic fragments. In the complexes thus obtained, the ion pairs act as ligand or counterion, partially or totally. The anionic fragment of the ion pair is usually, not always, coordinated with the metallic ion and lies in the ligand domain, and the counterion remains in the periphery domain [6]. A review of the literature showed that dicarboxylic-containing fragments in the resulting proton transfer compounds can function as suitable ligands in the synthesis of metal-organo complexes [7,8].
Among dicarboxylic acids, 2,6-pyridinedicarboxylic acid (dipicolinic acid, dipicH2) has attracted, as a very important carboxylate derivative, much interest in coordination chemistry [9–13]. In recent years, syntheses and crystal structures of a large number of proton transfer compounds and of their complexes with dipicolinic acid and some amino compounds have been reported [14].
Phenylenediamine (PDA) is found in ortho, meta and para isomeric forms and is used in syntheses of commercial hair dyes, polyamides, maleimides, and agricultural pesticides. The ortho form (OPD) of phenylenediamine (PDA) has two amino groups at 1 and 2 positions of the benzene ring. Thus, it could act as a good proton accepter in the proton transfer reactions. In this regard, a large number of proton transfer compounds have been constructed with o-phenylenediamine (OPD) acting as the proton acceptor with different acids such as chloroacetic acid [15], benzoic acid [16], malonic acid [17], phosphorous acid [18], and picric acid [19] as the proton donors. OPD can coordinate with the metal center as a unidentate in a cationic form [20], as a chelate in a neutral form [21–24], as well as a bridge between two metal atoms through amine groups [25], and it can be also found in some complexes as a discrete cation [26].
Complex anion [Co(dipic)2]2− is rather frequent in the literature [27–34]. It has pH-dependent labiality and in this regard is most similar to [VO2dipic]−. It is found that [Co(dipic)2]2− is effective in reducing the hyperlipidemia of diabetes using oral administration in drinking water in rats with STZ-induced diabetes [35]. According to the Cambridge Structural Database, organic ligands found as proton acceptor and cations in complexes with [Co(dipic)2]2− are imidazolium [29], 2,6-diaminopyridinium [30], propane-1,3-diaminium [31], l-histidine and l-ornithine [32], thiamine [33], and benzene-1,3-diammonium [34].
Here, we present the preparation and characterization of a proton transfer compound from dipicolinic acid and o-phenylenediamine and of its complex with cobalt, in which OPDH+ acts as a cation in complex anion [Co(dipic)2]2−.
2 Experimental
2.1 General methods and materials
OPD, dipicH2 and CoCl2·6H2O were purchased from Merck. Elemental analyses were performed by using a Leco CHNS-932 elemental analyzer. Fourier transform infrared spectra were recorded with a FTIR JASCO 680-PLUS spectrometer in the region from 4000 to 400 cm−1 using KBr pellets. Melting points were determined with an Electrothermal IA-900 apparatus. 1H NMR and 13C NMR spectra were obtained on a Bruker 250 (250 and 62.5 MHz, respectively) spectrometer. Chemical shifts were reported on the δ scale relative to TMS. Electronic spectra were obtained using a UV-JASCO-570 spectrometer.
2.2 Synthesis of proton transfer compound (OPDH)2(dipic)
Solutions of OPD (216 mg, 2 mmol) in 20 mL of water and 2,6-pyridinedicarboxylic acid (167 mg, 1 mmol) in H2O (10 mL) were added to each other in a 2:1 molar ratio, and the reaction mixture was heated at about 90 °C for 30 min. Pale brown crystals of the title compound in 80% yield were obtained from the solution after 2 days at room temperature. m.p.: 180–182 °C. 1H NMR (DMSO-d6) δ (ppm): 8.09–8.23 (m, 3H, dipic2−), 6.83–6.90 (m, 2H, OPDH+), 6.71–6.78 (m, 2H, OPDH+). 13C NMR (DMSO-d6) δ (ppm): 118.5, 121.4, 126.7, 132.2, 139.7, 150.4, 168.0. FTIR (KBr) cm−1: 3436, 3357, 3242, 3082, 2947, 1623, 1380, 1079, 757, 698. UV (aqueous solution) λ (nm): 224, 277.
2.3 Synthesis of [OPDH]2[Co(dipic)2]·H2O
A solution of CoCl2·6H2O (103 mg, 0.5 mmol) in 5 mL of water was added to 30 mL of a stirred aqueous solution containing 383 mg (1 mmol) of (OPDH)2(dipic). The reaction mixture was stirred at about 90 °C for 30 min. After 1 week, dark pink crystals of the title compound suitable for X-ray analysis were obtained (yield: 78%). Anal. calcd. for C26H26CoN6O9: C, 49.93; H, 4.19; N, 13.44; O 23.02. Found: C, 49.48; H, 4.14; N, 13.48; O, 23.09%; FTIR (KBr) cm−1: 3435, 3361, 3249, 2923, 1615, 1372, 585, 527, 453, 434, 420. UV–vis (aqueous solution) λ (nm): 224, 268, 456, 581.
2.4 X-ray crystallographic study
The diffraction data from a selected single crystal of [OPDH]2[Co(dipic)2]·H2O were collected at room temperature on an Oxford Diffraction Xcalibur Gemini S diffractometer using Mo Kα radiation (λ = 0.7107 Å). A total of 13,065 reflections (−10 ≤ h ≤ 10, −18 ≤ k ≤ 17, −19 ≤ l ≤ 21) were collected with 7509 independent reflections. The data were processed with CrysAlis software and Empirical absorption correction using spherical harmonics were implemented in SCALE3 ABSPACK scaling algorithm [36]. The crystallographic data, the data collection parameters, and the refinement parameters for (OPDH)2[Co(dipic)2]·H2O are summarized in Table 1. The crystal structure was solved by direct methods using Sir 2008 [37] and refined by full-matrix least-squares calculations against F2 using SHELXL [38]. All non-hydrogen atoms were refined with anisotropic displacement parameters. All aromatic CH atoms were included in their calculated positions and treated as riding atoms: CH = 0.93 Å for aromatic CH with Uiso(H) = 1.2 × Ueq(C). The figures were produced using ORTEP-3 [39], MERCURY [40]. The software used for the preparation of the materials for publication: WinGX [41], PLATON [42], PARST [43].
Crystal data, data collection and structure refinement details for [OPDH]2[Co(dipic)2]·H2O.
Empirical formula | C26H26CoN6O9 |
Formula weight | 625.46 |
Color, shape | Dark pink |
Temperature (K) | 293 |
Crystal size (mm) | 0.185 × 0.100 × 0.038 |
Crystal system | Triclinic |
Space group | |
a (Å) | 7.1740(17) |
b (Å) | 12.9960(2) |
c (Å) | 15.5240(2) |
α (°) | 67.463(13) |
β (°) | 81.981(15) |
γ (°) | 87.820(15) |
Volume (Å3) | 1323.6(4) |
Z | 2 |
λ(Å) | 0.7107 |
Calculated density, ρ (g·cm−3) | 1.569 |
θ range for data collection (°) | 3.2113–31.1769 |
Absorption coefficient (mm−1) | 5.671 |
F(000) | 646 |
Reflections collected | 13065 |
Independent reflections | 6060 [R(int) = 0.0638] |
Parameters | 427 |
Goodness-of-fit | 1.090 |
R1 [I > 2σ(I)] | 0.1262 |
wR2 (all data) | 0.1443 |
Largest ΔF peak and hole (e·Å−3) | 0.584 and −0.373 |
2.5 Potentiometric equilibrium measurements
A Model 794 Metrohm Basic Titrino was attached to an extension combined glass-calomel electrode mounted in an air-protected, sealed, thermostated jacketed cell maintained at 25.0 ± 0.1 °C by circulating water, from a constant-temperature bath Fisherbr and model FBH604, LAUDA, Germany, equipped with a stirrer and a 10,000-mL-capacity Metrohm piston burette. The pH-meter electrode system was calibrated to read–log [H+]. The jacketed cell containing the test solution was equipped with a magnetic stirrer and a tightly fitting cap, through which the electrode system and the 10-mL capacity Metrohm piston burette were inserted and sealed with clamps and O-rings. The atmospheric CO2 was excluded from the titration cell with a purging steam of purified nitrogen gas. Known amounts of dipic, OPD and dipic/OPD (1:2 molar ratio) aqueous solutions (2 × 10−3 and 4 × 10−3 M), respectively, were titrated in the absence and the presence of 1 × 10−3 M CoII ion, at 25 °C. A standard carbonate-free NaOH solution (0.11075 M) was used in all titrations. The ionic strength was adjusted to 0.1 M with NaNO3. Sufficient time was allowed to elapse before measuring the experimental point (pH), to establish the equilibrium protonation constants of the ligands and the stability constants of proton transfer, and their metal complexes were evaluated using the Hyperquad 2008 program as a new version of the older one, which permits the determination of formation constants even when multiple equilibria are involved [44]. Distribution diagrams were drawn using the Hyss 2009 program as a new version of an older one [45]. The value of the autoprotolysis constant for the aqueous system, Kw = [H+]·[OH−], was chosen according to the literature [46].
3 Results and discussion
3.1 Synthesis and characterization
The reaction between dipicH2 and OPD at a 1:2 molar ratio was accomplished to prepare the pale brown compound (OPDH)2(dipic) (Scheme 1). The melting point of (OPDH)2(dipic) compound is quite different from that of the starting materials. The IR and NMR spectroscopic studies were conducted to characterize the chemical structure of (OPDH)2(dipic).
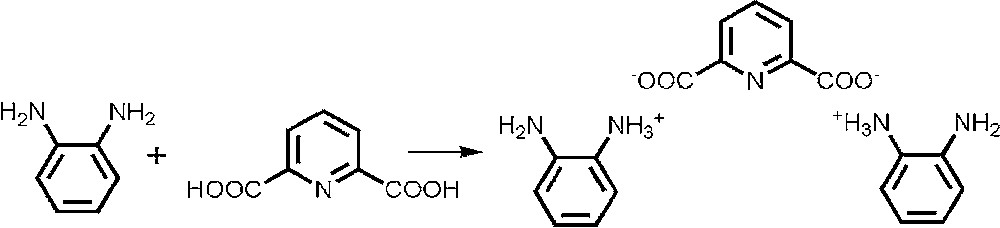
Synthetic pathway of compound (OPDH)2(dipic).
The IR spectroscopy results obtained for compound (OPDH)2(dipic) shows stretching frequencies at 3357, 3243 cm−1 corresponding to the NH group of OPD. The band observed in the 2900–3080 cm−1 region is the characteristic νCH band of the aromatic ring vibrations. The most distinct feature in the IR spectra of the proton transfer compound is the presence of strong peaks at 1380 and 1623 cm−1 due to the resonance of (COO)−. In fact, ν(CO) and ν(CO) bands are transformed into the symmetric and asymmetric stretching vibrations of the carboxylate anion [47].
The UV spectrum of aqueous solution of (OPDH)2(dipic) is illustrated in Fig. 1c. The absorption with a maximum at 277 nm can be assigned to the n → π* transition of carboxyl CO of (dipic)2−group. Moreover, this absorption, which appears in the spectra of dipicH2 at 271.5, is shifted to a larger wavelength. The observed large redshift toward larger wavelengths is due to a proton transfer from the diacid to the amine molecule and the consequent interaction of the resulting oppositely charged species [48].
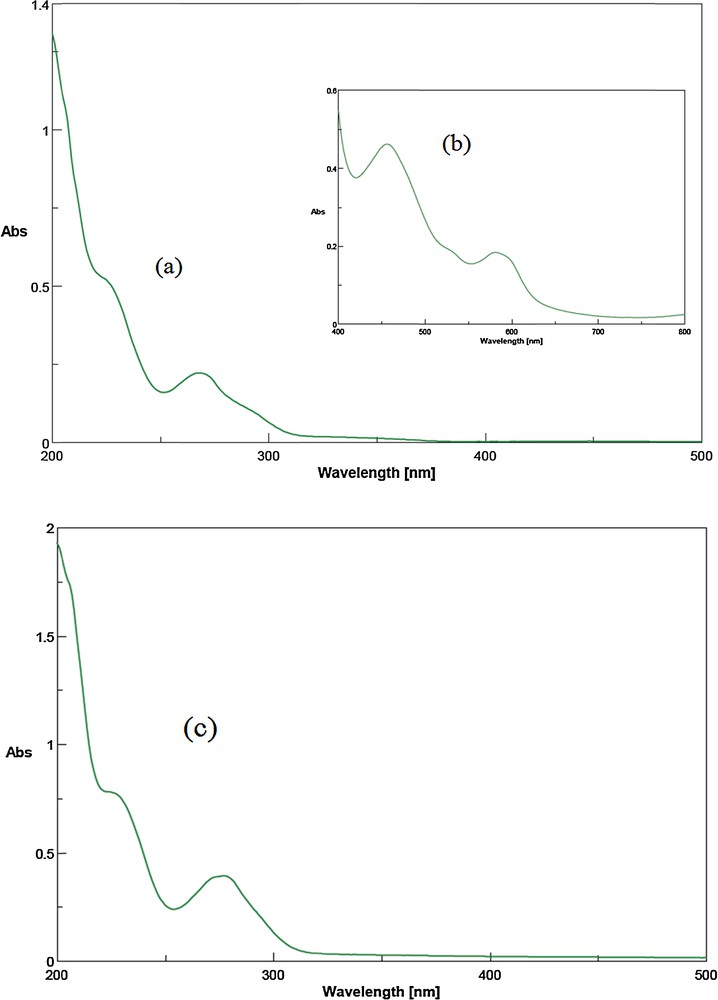
Electronic spectra of [OPDH]2[Co(dipic)2]·H2O (a: 1.96 × 10−5 M; b: 2.0 × 10−3 M) and of the proton transfer compound (OPDH)2(dipic); (c: 2.7 × 10−5 M) in aqueous solution.
Considering the 1H and 13C NMR data of (OPDH)2(dipic), in DMSO-d6, one clearly realizes that both dipic2− and OPDH+ are present in (OPDH)2(dipic). The 1H NMR shows three resonances, out of which the one at 8.09–8.23 ppm is assigned to dipic2− and the remaining two correspond to OPDH+. In the 13C NMR spectrum, four signals at 126.7, 139.7, 150.4 and 168.0 ppm out of the total of seven could definitely be assigned to dipic2−, and another three peaks can be assigned to the carbon atoms of OPDH+. From the number of resonances in both spectra and the integration of the 1H resonances, it can be concluded that (OPDH)2(dipic) consists of both OPDH+ and dipic2− fragments, respectively.
The reaction of (OPDH)2(dipic) with CoCl2·6H2O in 2:1 molar ratio in water was carried out at 90 °C and the dark pink crystals were obtained by slow evaporation of the solvent at room temperature. The elemental analysis of the complex is entirely consistent with its composition determined by X-ray crystallography (C26H26CoN6O9).
The IR spectrum of the complex shows a strong, broad and branched peak at 3435–2647 cm−1 attributed to ν(OH) of water molecules, to the aromatic CHs of phenyl and pyridine rings, as well as to the NH stretching vibrations, which are well broadened and are seen in this range. The strong absorption bands at 1615 and 1372 cm−1 correspond to the asymmetric and symmetric stretching of carboxyl groups, respectively. The value of Δ[vas–vs] is 243 cm−1, comparatively larger than 200 cm−1, indicating a monochelation of the carboxylic group to the metal ion [49]. The absorption bands at 585 and 527 cm−1 correspond to CoO vibrations, and the bonds observed at 453, 434 and 420 cm−1 are attributed to the CoN vibrations of the complex.
The electronic absorption spectrum of [OPDH]2[Co(dipic)2]·H2O in aqueous solution was recorded in the range from 200 to 800 nm (Fig. 1a and b). In the UV region, the complex exhibits two absorption bands. The comparison of the absorption bands with the absorption spectrum of the proton transfer compound (Fig. 1c) denotes that the band centered at 268 nm can be assigned to an n → π* transition of carboxyl CO of (dipic)2− group. However, in [OPDH]2[Co(dipic)2]·H2O, where (dipic)2− is coordinated with the cobalt(II) ion, due to the presence of non-bonding carboxyl O atoms in coordination with the metal ion, the energy band of non-bonding species is getting stable and therefore the energy transfer to π* is shifted toward higher energies (lower wavelengths, 268 nm). Furthermore, the complex shows two broad bands, centered at 456 and 581 nm, which are due to spin-allowed d–d transitions. These two d–d bands are commonly assigned to 4T1g(F) → 4T2g (F) and 4T1g(F) → 4T1g(P) transitions, respectively, of the octahedral high-spin d7 CoII ion. The third spin-allowed d–d band, which corresponds to the 4T1g(F) → 4A2g(F) transition, being essentially a two-electron transition from (t2g)5(eg)2 to (t2g)3(eg)4 configurations, is expected to be weak and is probably not observed [50,51].
3.2 X-ray crystal structure of complex [OPDH]2[Co(dipic)2]·H2O
The asymmetric unit of [OPDH]2[Co(dipic)2]·H2O is composed of one complex anion [Co(dipic)]−2, of two o-phenylenediamine cations, which are formed through the transfer of one proton from an acid group to an amine group, and of one crystallization water molecule (Fig. 2).
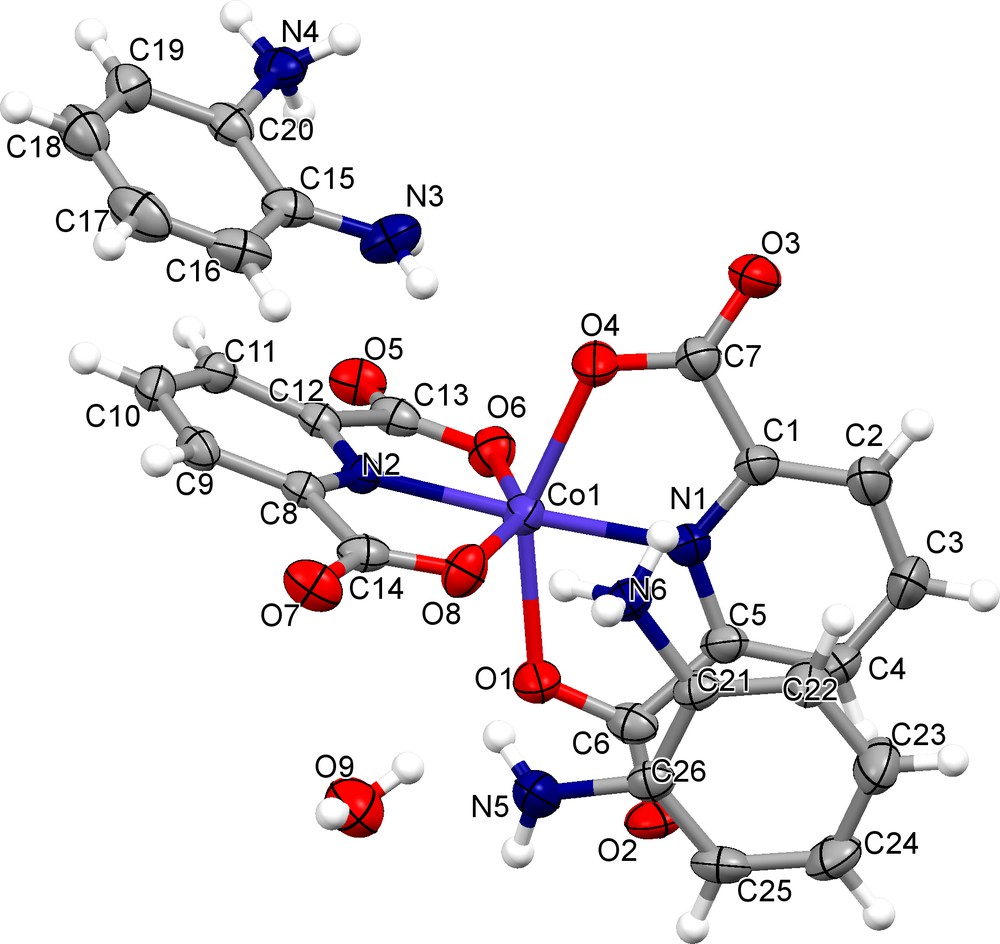
(Color online.) Asymmetric unit of [OPDH]2[Co(dipic)2]·H2O, showing 50%-probability displacement ellipsoids.
The two dipic ligands are deprotonated in the complex, both are coordinated to one cobalt atom in a tridentate manner, resulting a distorted octahedral coordination environment formed by four oxygen atoms and two nitrogen atoms. This type of complex had previously been observed in, e.g., the structure of bis(imidazolium 2,6-pyridinedicarboxylate) cobalt(II) dihydrate [29]. The values of CoN bond distances are 2.035(3) and 2.039(3) Å, and the Co(1)O bond distances range from 2.118 (3) to 2.220 (3) Å (average 2.157 Å). All bond lengths are in agreement with those common to the related structures (Cambridge Structural Database CSD.2013) [52] (Table 2).
Selected bond lengths (Å) and bond angles (°) for the complex [OPDH]2[Co(dipic)2]·H2O.
N(1)-Co(1) | 2.039(3) | O(4)-Co(1) | 2.142(3) |
N(2)-Co(1) | 2.032(3) | O(8)-Co(1) | 2.217(3) |
O(1)-Co(1) | 2.121(3) | O(6)-Co(1) | 2.161(3) |
O(1)-Co(1)-C(8) | 88.52(11) | O(4)–Co(1)–O(6) | 93.73(11) |
O(1)-Co(1)-O(4) | 151.39(10) | O(6)–Co(1)–N(1) | 104.17(11) |
O(1)-Co(1)-N(1) | 75.87(12) | O(6)–Co(1)–N(2) | 76.90(10) |
O(1)-Co(1)-N(2) | 108.13(11) | O(8)–Co(1)–N(1) | 105.06(11) |
O(1)-Co(1)-O(6) | 95.56(11) | O(8)–Co(1)–N(2) | 74.16(10) |
O(4)-Co(1)-O(8) | 96.47(11) | O(6)–Co(1)–O(8) | 150.59(9) |
O(4)-Co(1)-N(1) | 75.87(12) | N(1)–Co(1)–N(2) | 176.06(14) |
O(4)-Co(1)-N(2) | 100.31(11) |
In the crystal structure, two different types of strong intermolecular hydrogen bonds (OH···O, and NH···O) involving carboxylate groups, amine groups, as well as water molecules are forming a three-dimensional network (Table 3 and Fig. 3).
Hydrogen bonds for [OPDH]2[Co(dipic)2]·H2O.
D–H···A | D–H (Å) | H···A (Å) | D···A (Å) | D–H···A (°) |
N3–H(3a)···O5(ii) | 0.90(5) | 2.25(7) | 3.117(6) | 160(6) |
N3–H(3b)···O9(iii) | 0.79(4) | 2.51(6) | 3.264(8) | 167(5) |
N4–H(4a)···O5 (iv) | 0.88(2) | 1.97(5) | 2.853(6) | 171(5) |
N4–H(4b)···O3 (i) | 0.89(2) | 1.84(4) | 2.735(7) | 166(5) |
N4–H(4c)···O5(ii) | 0.90(4) | 2.06(6) | 2.838(6) | 145(5) |
N5–H(5a)···O8 (v) | 0.86(2) | 2.10(6) | 2.927(5) | 155(5) |
N5–H(5b)···O7(vi) | 0.89(2) | 2.23(7) | 3.102(6) | 167(2) |
N6–H(6a)···O2(vii) | 0.88(2) | 1.97(5) | 2.852(6) | 169(5) |
N6–H(6b)···O7(viii) | 0.88(2) | 1.93(5) | 2.787(5) | 164(5) |
N6–H(6c)···O8 (v) | 0.97(4) | 1.76(5) | 2.773(5) | 162(4) |
O9–H(9a)···O1(v) | 0.82(2) | 1.89(6) | 2.703(6) | 163(6) |
O9–H(9b)···N5(ix) | 0.84(7) | 2.18(2) | 3.010(7) | 175(1) |
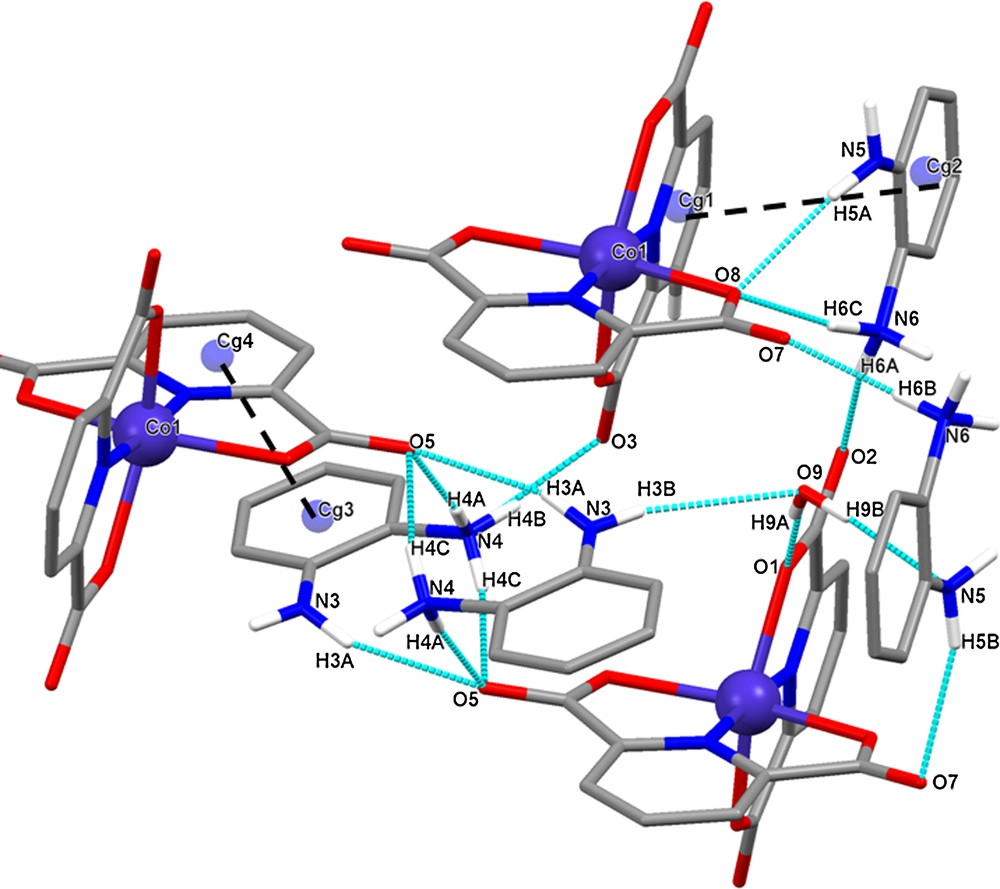
(Color online.) Representation of the Hbonds in the crystal packing of [OPDH]2[Co(dipic)2]·H2O.
Additionally, in this crystal structure, the N-containing rings (OPDH+) and the phenyl rings of ligand (dipic2−) are involved in π–π stacking interactions [Cg(1)–Cg(2) dπ–π 3.965 (3) Å, dihedral angle between stacked ring planes α: 9.0(2)°, slipping angle β 14.51 and for Cg(3)–Cg(4) dπ–π 4.176(3) Å, α: 9.4(2)°, slipping angles β: 30.31°]; even if these interaction are weaker than the strong hydrogen bonds, they give an additional stability to the crystal structure (Fig. 3).
3.3 Solution studies
In preliminary experiments, the fully protonated forms of dipic (L) and OPD (Q) were titrated with a standard NaOH solution in order to obtain some information about their protonation constants as the building blocks of the dipic-OPD proton transfer. The protonation constants of dipic and OPD were calculated by fitting the volume-pH data (Figs. 4a and 5a). The potentiometric data were processed using Hyperquad 2008 program. The results are summarized in Table 4.

(Color online.) Potentiometric titration curves of dipic (L) in the absence and presence of the CoII ion with NaOH 0.11075 M in aqueous solution at 25 ± 0.1 °C and μ = 0.1 M NaNO3, (a) and the corresponding distribution diagrams (b, c).

(Color online.) Potentiometric titration curves of o-phenylenediamine (OPD) (Q), (a) in the absence and in the presence of the CoII ion with NaOH 0.11075 M in aqueous solution at 25 ± 0.1 °C and μ = 0.1 M NaNO3, and distribution diagram of OPD (Q), (b).
Overall stability and stepwise protonation constants of dipic and OPD and recognition constants for the interaction between them in aqueous solution at 25 ± 0.1 °C and μ = 0.1 M NaNO3.
Stoichiometry | log β | Equilibrium quotient K | log K | Max % | At pH | ||
Dipic | OPD | H | |||||
1 | 0 | 1 | 4.96 ± 0.05 | ---------------------- | 4.96 ± 0.05 | 91.7 | 3.6 |
1 | 0 | 2 | 7.23 ± 0.06 | ---------------------- | 2.27 ± 0.08 | 65.25 | 2.0 |
0 | 1 | 1 | 4.90 ± 0.05 | ---------------------- | 4.90 ± 0.05 | 96.22 | 3.2 |
0 | 1 | 2 | 6.38 ± 0.10 | ---------------------- | 1.48 ± 0.12 | 23.29 | 2.0 |
1 | 1 | 0 | 4.23 ± 0.29 | [dipicOPD]/[dipic][OPD] | 4.23 ± 0.29 | 48.62 | > 7.8 |
1 | 1 | 2 | 13.68 ± 0.29 | [dipicOPDH2]/[dipicH][OPDH] | 3.82 ± 0.30 | 35.02 | 3.6 |
1 | 2 | 2 | 16.98 ± 0.34 | [dipic(OPD)2H2]/[dipicH][OPDH][OPD] | 7.12 ± 0.35 | 17.62 | 4.6 |
[dipic(OPD)2H2]/[dipic][OPDH]2 | 7.18 ± 0.35 | ||||||
1 | 2 | 4 | 24.16 ± 0.35 | [dipic(OPD)2H4]/[dipicH2][OPDH]2 | 7.12 ± 0.36 | 32.12 | 2.0 |
[dipic(OPD)2H4]/[dipicH][OPD H2][OPDH] | 7.91 ± 37 |
The protonation constants of dipic and OPD are in good agreement with those reported in the literature [53–56]. As one can see, for each ligand, two protonation constants were obtained; for dipic, the nitrogen in the pyridine ring was protonated firstly and then one of the carboxylate groups; for OPD, two protonation constants related to two amine groups. Distribution diagrams for dipic and OPD are shown on Figs. 4b and 5b, respectively.
The evaluation of the equilibrium constants for the interaction between dipic and OPD, in different protonation forms, was accomplished through the comparison of the calculated and experimental pH profiles [57–59], obtained with both dipic and OPD present. The results are shown in Table 4. The corresponding species distribution diagram is shown in Fig. 6. As it is obvious, the most abundant proton transfer species present in the pH range 7.8–12 (48.62%), 3.6 (35.02%), 4.6 (17.62%) and 2 (32.12%) are [dipicOPD] (log K = 4.23 ± 0.29), [dipicHOPDH] (log K = 3.82 ± 0.30), {[dipicHOPDHOPD] (log K = 7.12 ± 0.35), [dipicOPDHOPDH] (log K = 7.18 ± 0.35)} and {[dipicH2OPDHOPDH] (log K = 7.12 ± 0.36), [dipicHOPDHOPDH2] (log K = 7.91 ± 0.37)}, respectively. It is interesting to note that the LQ2H2 species in aqueous solution with the abundant (17.62%) are the same as that observed in the solid state. The stability of proton transfer species depends on some factors such as the nature of the solvent, the power and the number of sites on acid and base as building structure of proton transfer for Hbonding formation, the attractive forces of opposite charges and the repulsive forces of similar charges on acid and base, the topology and the geometry of the acid and the base.
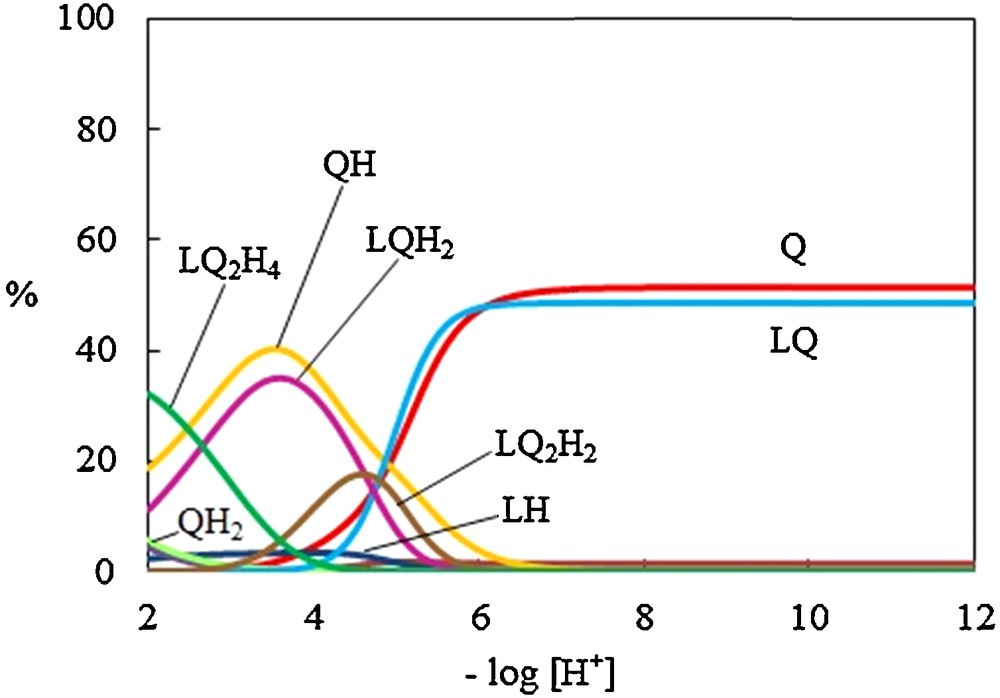
(Color online.) Distribution diagram of the proton transfer interaction between dipic (L) and o-phenylenediamine (Q).
In order to evaluate the stoichiometry and stability of CoII ion complexes with the dipic-OPD proton transfer system in aqueous solution, the equilibrium potentiometric pH titration profiles of dipic, OPD and their 1:2 mixture were obtained in the absence and in the presence of the CoII ion. The resulting pH profiles are shown on Figs. 4a, 5a and 7a. It is seen from Fig. 5a that the titration of OPD in the presence of the CoII ion was stopped when the precipitate was observed. It was found that OPD with the CoII ion has no interaction before the precipitate is observed. The potentiometric titration curves of dipic and dipic-OPD mixtures are depressed in a relatively considerable fashion in the presence of the CoII ion.
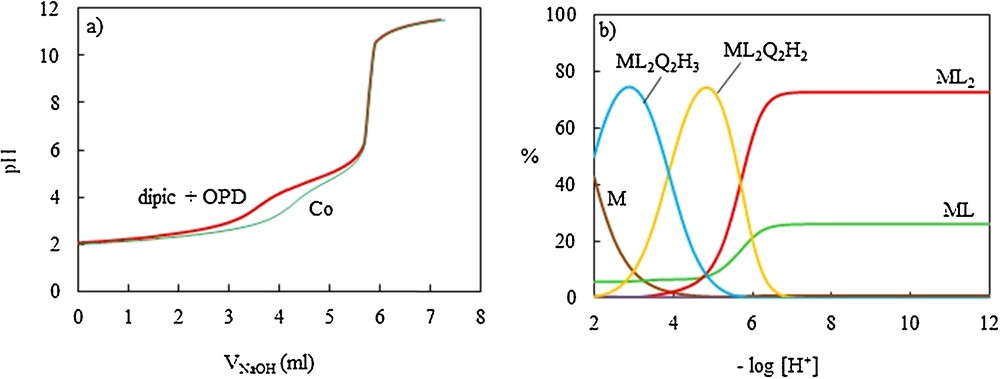
(Color online.) Potentiometric titration curves of dipic + o-phenylenediamine (OPD) in the absence and the presence of the CoII ion with NaOH 0.11075 M in aqueous solution at 25 ± 0.1 °C and μ = 0.1 M NaNO3, (a) and distribution diagram of dipic (L)/OPD (Q)/CoII ternary systems (b).
The cumulative stability constants of MmLlQqHh, βmlqh, are defined in our previous publications [60,61], where M, L, Q, and H are metal ion, dipic, OPD and proton, respectively, and m, l, q, and h are the respective stoichiometric coefficients.
The cumulative stability constants were evaluated by fitting the corresponding pH–volume data to the Hyperquad 2008 program and the resulting values for the most likely complex species in aqueous solution are also included in Table 5. The corresponding distribution diagrams are shown in Figs. 4c and 7b. These results revealed that the CoII ion has no interaction with OPD, while it forms relative stable complexes with dipic and dipic-OPD systems. In the dipic–Co binary system, the most likely species are: ML (at pH = 2.2), ML2 (at pH > 8.5), ML2H (at pH = 2.3).
Overall stability constants of dipic/OPD/CoII (l/q/m) binary and ternary systems in aqueous solution at 25 ± 0.1 °C and μ = 0.1 M NaNO3.
System | m | l | q | h | log β | Max % | At pH |
Co-dipic | 1 | 1 | 0 | 0 | 6.82 ± 0.65 | 34.61 | 2.2 |
1 | 2 | 0 | 0 | 12.55 ± 0.11 | 96.09 | > 8 | |
1 | 2 | 0 | 1 | 15.32 ± 0.29 | 42.34 | 2.3 | |
Co–dipic-OPD | 1 | 2 | 2 | 2 | 29.12 ± 0.22 | 74.24 | 4.8 |
1 | 2 | 2 | 3 | 33.00 ± 0.15 | 74.49 | 2.9 |
Fig. 7b and Table 5 revealed the formation of a variety of ternary complexes between the CoII ion and the proton transfer system in different pH ranges. The predominant species are: ML2Q2H2 (at pH = 4.8) and ML2Q2H3 (at pH = 2.9). It is worth mentioning that the stoichiometry of one of the most abundant ternary complexes existing in aqueous solution, ML2Q2H2, is the same as that reported for the corresponding isolated complexes in the solid state.
4 Conclusions
We have evidenced that the reaction between the proton transfer compound from 2,6-pyridinedicarboxylic acid and o-phenylenediamine with cobalt leads to the formation of complex [OPDH]2[Co(dipic)2]·H2O. Different non-covalent interactions such as π–π stacking and hydrogen bonding play important roles in the construction of three-dimensional networks in the crystal system. The potentiometric studies made in this solution provided additional evidences of the interaction between dipic-OPD proton transfer compound and the CoII ion, which supports the results obtained from solid-state studies. The stoichiometry of the complex species in solution was the same as that of the crystalline metal ion complex.
Acknowledgement
We gratefully acknowledge the support of this work by the Iranian Government and the University of Sistan and Baluchestan (Grant, No. 90g10). Financial support from Spanish Ministerio de Ciencia e Innovación (MAT2010-15094 and ‘Factoría de Cristalización’ Consolider Ingenio 2010, CSD2006-015) and ERDF funding is acknowledged.