[Comportement thermique d’un Matériau Composite (nano-MCP/mousse d’aluminium) utilisé pour des applications de stockage d’énergie thermique (TES)]
Les systèmes de stockage de l’énergie thermique (TESS) utilisant des matériaux à changement de phase (MCP) ont suscité l’intérêt dans divers domaines de la science et de la technologie. Cependant, l’intérêt de ces systèmes est limité par la conductivité thermique relativement faible des MCP et leur fuite à l’état fondu. Pour surmonter ces inconvénients, le présent document propose une nouvelle alternative pour l’amélioration des MCP en incorporant des matériaux hautement conducteurs, tels que mousse métallique et/ou nanoparticules. Une simulation numérique directe (DNS) a été réalisée pour étudier le processus de fusion de la cire de paraffine comme MCP amélioré par des nanoparticules d’alumine (c-à-d un nano-MCP) incorporées dans de la mousse d’aluminium à température constante. Une structure tridimensionnelle (3D) régulière de la mousse a été conçue. Les effets de la porosité de la mousse d’aluminium et de la fraction volumique des nanoparticules sur le comportement thermique des MCP composites ont été étudiés. Le modèle à deux températures basé sur l’hypothèse d’un non-équilibre thermique local a été appliqué en raison de la grande différence de conductivité thermique entre le nano-MCP et la mousse d’aluminium.
Les résultats ont montré que la mousse d’aluminium et les nanoparticules d’alumine (AlO) accélèrent toutes deux de manière significative le processus de fusion. En outre, la conductivité thermique effective du MCP composite (nano-MCP/mousse d’aluminium) a augmenté de manière drastique. Les résultats de la DNS ont montré une différence de température importante entre le MCP et le ligament métallique, assurant la viabilité du non-équilibre thermique local.
Thermal energy storage systems (TESS) using phase change materials (PCM) have attracted interest in various fields of science and technology. However, the interest of these systems is limited by the relatively low thermal conductivity of PCMs and their leakage in the melted state. To overcome these drawbacks, the present paper suggests a new alternative for the enhancement of the PCMs by incorporating highly conductive materials, such as metal foam and/or nanoparticles. A Direct Numerical Simulation (DNS) was carried out to investigate the melting process of paraffin wax as PCM enhanced with alumina nanoparticles (i.e. nanoPCM) embedded in aluminum foam under constant temperature. A three-dimensional (3D) foam regular structure was designed. The effects of aluminum foam porosity and nanoparticles’ volume fraction on the thermal behavior of composite PCMs were investigated. The two-temperature model based on the assumption of local thermal non equilibrium was applied due to the great difference of thermal conductivity between nanoPCM and aluminum foam.
The results showed that both aluminum foam and alumina nanoparticles (AlO) significantly accelerate the melting process. Furthermore, the effective thermal conductivity of composite PCM (nanoPCM/aluminum foam) drastically increased. The DNS results showed important temperature difference between PCM and metallic ligament, assuring the viability of local thermal non-equilibrium.
Révisé le :
Accepté le :
Première publication :
Publié le :
Mot clés : Nanoparticules d’alumine, Mousse d’aluminium, Matériau à changement de phase, Stockage d’énergie thermique, Simulation numérique directe
H. Mhiri 1 ; A. Jemni 1 ; H. Sammouda 1
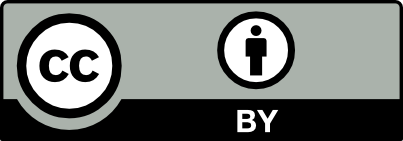
@article{CRPHYS_2020__21_3_233_0, author = {H. Mhiri and A. Jemni and H. Sammouda}, title = {Thermal behavior of {Composite} {Material} {(nanoPCM/aluminum} foam) used for thermal energy storage {(TES)} applications}, journal = {Comptes Rendus. Physique}, pages = {233--252}, publisher = {Acad\'emie des sciences, Paris}, volume = {21}, number = {3}, year = {2020}, doi = {10.5802/crphys.2}, language = {en}, }
TY - JOUR AU - H. Mhiri AU - A. Jemni AU - H. Sammouda TI - Thermal behavior of Composite Material (nanoPCM/aluminum foam) used for thermal energy storage (TES) applications JO - Comptes Rendus. Physique PY - 2020 SP - 233 EP - 252 VL - 21 IS - 3 PB - Académie des sciences, Paris DO - 10.5802/crphys.2 LA - en ID - CRPHYS_2020__21_3_233_0 ER -
%0 Journal Article %A H. Mhiri %A A. Jemni %A H. Sammouda %T Thermal behavior of Composite Material (nanoPCM/aluminum foam) used for thermal energy storage (TES) applications %J Comptes Rendus. Physique %D 2020 %P 233-252 %V 21 %N 3 %I Académie des sciences, Paris %R 10.5802/crphys.2 %G en %F CRPHYS_2020__21_3_233_0
H. Mhiri; A. Jemni; H. Sammouda. Thermal behavior of Composite Material (nanoPCM/aluminum foam) used for thermal energy storage (TES) applications. Comptes Rendus. Physique, Volume 21 (2020) no. 3, pp. 233-252. doi : 10.5802/crphys.2. https://comptes-rendus.academie-sciences.fr/physique/articles/10.5802/crphys.2/
[1] Electron tunnelling and hopping effects on the temperature coefficient of resistance of carbon nanotube/polymer nanocomposites, Phys. Chem. Chem. Phys., Volume 19 (2017), pp. 5113-5120 | DOI
[2] A work procedure of utilising PCMs as thermal storage systems based on air-TES systems, Energy Convers. Manag. (2014), pp. 608-627 | DOI
[3] A review on phase change material (PCM) for sustainable passive cooling in building envelopes, Renewable and Sustainable Energy Reviews, Volume 60 (2016), pp. 1470 -1497 | DOI
[4] Form-stable phase change materials for thermal energy storage, Renew. Sustain. Energy Rev., Volume 16 (2012), pp. 1999-2040 | DOI
[5] Review on thermal performances and applications of thermal energy storage systems with inorganic phase change materials, Energy (2018), pp. 685-708 | DOI
[6] An experimental investigation of discharge/solidification cycle of paraffin in novel shell and tube with longitudinal fins based latent heat storage system, Energy Convers. Manag., Volume 154 (2017), pp. 157-167 | DOI
[7] Thermal performance of copper foam/paraffin composite phase change material, Energy Convers. Manag., Volume 157 (2018), pp. 372-381 | DOI
[8] Synthesis of antistatic hybrid nanocomposite coatings using surface modified indium tin oxide (ITO) nanoparticles, J. Coatings Technol. Res. (2014), pp. 587-593 | DOI
[9] Microencapsulation of butyl stearate as a phase change material by interfacial polycondensation in a polyurea system, Energy Convers. Manag. (2009), pp. 723-729 | DOI
[10] Melting of nano-PCM inside a cylindrical thermal energy storage system: numerical study with experimental verification, Energy Convers. Manag. (2018), pp. 241-259 | DOI
[11] Polymer nanocomposites, printable and flexible technology for electronic packaging, Proc. - 2009 Int. Symp. Microelectron. IMAPS 2009 (2009), p. 1
[12] Paper based, expanded graphite/polypyrrole nanocomposite supercapacitors free from binders and current collectors, J. Electrochem. Soc., Volume 165 (2018), p. A283-A290 | DOI
[13] Fabrication and electrical characterizations of graphene nanocomposite thin film based heterojunction diode, Phys. B Condens. Matter. (2017), pp. 97-103 | DOI
[14] Next-generation polymer nanocomposite-based electrochemical sensors and biosensors: a review, TrAC - Trends Anal. Chem., Volume 82 (2016), pp. 55-67 | DOI
[15] Synthesis of antistatic hybrid nanocomposite coatings using surface modified indium tin oxide (ITO) nanoparticles, J. Coatings Technol. Res. (2014), pp. 587-593 | DOI
[16] A multi-component nanocomposite screen-printed ink with non-linear touch sensitive electrical conductivity, Nanotechnology (2013), 23535342 | DOI
[17] Thermal conductivity enhancement by using nano-material in phase change material for latent heat thermal energy storage systems, Saussurea (2015), pp. 48-55
[18] A facile microencapsulation of phase change materials within silicone-based shells by using glass capillary devices, Colloids Surfaces A Physicochem. Eng. Asp. (2019), pp. 297-303 | DOI
[19] Poly dimethylsiloxane/carbon nanofiber nanocomposites: fabrication and characterization of electrical and thermal properties, Int. J. Smart Nano Mater., Volume 7 (2016), pp. 236-247 | DOI
[20] Review on polymer/graphite nanoplatelet nanocomposites, J. Mater. Sci., Volume 46 (2011), pp. 5595-5614 | DOI
[21]
(“Electrical percolation in metal nanowire networks for bulk polymer nanocomposites and transparent conductors, and resistive switching in metal/polymer nano-gap devices”, ProQuest Diss. Theses, 2013)[22] Thermal characteristics of expanded perlite/paraffin composite phase change material with enhanced thermal conductivity using carbon nanotubes, Energy Convers. Manag., Volume 134 (2017), pp. 373-381 | DOI
[23] A review of materials, heat transfer and phase change problem formulation for latent heat thermal energy storage systems (LHTESS), Renew. Sustain. Energy Rev. (2010), pp. 615-628 | DOI
[24] A nano-graphite/paraffin phase change material with high thermal conductivity, Appl. Energy, Volume 106 (2013), pp. 25-30 | DOI
[25] Thermal conductivity of an organic phase change material/expanded graphite composite across the phase change temperature range and a novel thermal conductivity model, Energy Convers. Manag., Volume 102 (2015), pp. 202-208 | DOI
[26] Experimental investigation of the effects of using nano/phase change materials (NPCM) as coolant of electronic chipsets, under free and forced convection, Appl. Therm. Eng., Volume 111 (2017), pp. 271-279 | DOI
[27] Experimental study on solidification process of a phase change material containing TiO2 nanoparticles for thermal energy storage, Energy Convers. Manag. (2017), pp. 162-170 | DOI
[28] Heat transfer study of phase change materials with graphene nano particle for thermal energy storage, Sol. Energy, Volume 146 (2017), pp. 453-463 | DOI
[29] Numerical study on melting of paraffin wax with AlO in a square enclosure, Int. Commun. Heat Mass Transf. (2012), pp. 8-16 | DOI
[30] Preparation and thermal performance of paraffin/Nano-SiO nanocomposite for passive thermal protection of electronic devices, Appl. Therm. Eng., Volume 96 (2016), pp. 699-707 | DOI
[31] Paraffin nanocomposites for heat management of lithium-ion batteries: a computational investigation, J. Nanomater. (2016), 2131946 | DOI
[32] Experimental study of a phase change thermal energy storage with copper foam, Appl. Therm. Eng. (2016), pp. 247-261 | DOI
[33] Open-cell aluminum foams filled with phase change materials as compact heat sinks, Scr. Mater. (2006), pp. 887-890 | DOI
[34] Effective thermal conductivity of open-cell metal foams impregnated with pure paraffin for latent heat storage, Int. J. Therm. Sci. (2014), pp. 94-105 | DOI
[35] Enhanced thermal management with microencapsulated phase change material particles infiltrated in cellular metal foam, Energy (2017), pp. 671-679 | DOI
[36] Experimental and numerical study on melting of phase change materials in metal foams at pore scale, Int. J. Heat Mass Transf. (2014), pp. 646-655 | DOI
[37] Lattice Boltzmann simulation on phase change heat transfer in metal foams/paraffin composite phase change material, Appl. Therm. Eng., Volume 93 (2016), pp. 476-485 | DOI
[38] Experimental and numerical studies on melting phase change heat transfer in open-cell metallic foams filled with paraffin, Appl. Therm. Eng., Volume 37 (2012), pp. 1-9 | DOI
[39] Energy storage system based on nanoparticle-enhanced phase change material inside porous medium, Int. J. Therm. Sci. (2015), pp. 49-58 | DOI
[40] Enthalpy-porosity technique for modeling convection-diffusion phase change: application to the melting of a pure metal, Numer. Heat Transf., Volume 13 (1988), pp. 297-318 | DOI
[41] Experimental and numerical investigation of melting of NePCM inside an annular container under a constant heat flux including the effect of eccentricity, Int. J. Heat Mass Transf., Volume 67 (2013), pp. 455-468 | DOI
[42] Phase-change materials to improve solar panel’s performance, Energy Build., Volume 62 (2013), pp. 59-67 | DOI
Cité par Sources :
Commentaires - Politique