Ces dernières années, la thématique des métasurfaces est devenue un sujet de recherche en pleine expansion dans plusieurs domaines de l’ingénierie et de la physique appliquée, en raison de leur capacité à manipuler à la fois la phase et l’amplitude des champs électromagnétiques. Ces matériaux artificiels bi-dimensionnels, généralement composés d’éléments métalliques imprimés sur des substrats diélectriques, ont l’avantage d’être de très faible épaisseur, légers et faciles à fabriquer et à intégrer avec les circuits imprimés. Cet article passe en revue les dernières avancées dans la conception d’antennes à métasurface, où les métasurfaces sont utilisées pour minimiser l’épaisseur, augmenter la bande passante et contrôler le diagramme de rayonnement en champ proche et en champ lointain.
In recent years, metasurfaces have become a rapidly growing domain of research in several fields of engineering and applied physics due to their ability to manipulate both phase and amplitude of electromagnetic fields. These artificial 2D-materials, usually composed of metallic elements printed on dielectric substrates, have the advantages of being low profile, lightweight as well as easy to fabricate and integrate with standard circuit technologies. In this context, this paper reviews the latest progress in metasurface antenna design, where metasurfaces are used to miniaturize the profile, increase the bandwidth, and control the radiation pattern in the near- and far-field regions.
Publié le :
Mot clés : Métasurface, Antennes à métasurface, Surfaces artificielles, Surface d’impédance, Cavité de Fabry–Pérot, Antennes à fuite, Symétrie de glissement
Massimiliano Casaletti 1, 2 ; Guido Valerio 1, 2 ; Oscar Quevedo-Teruel 3 ; Paolo Burghignoli 4
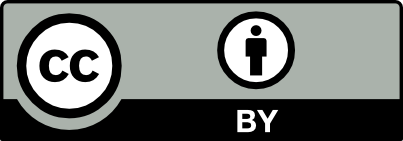
@article{CRPHYS_2020__21_7-8_659_0, author = {Massimiliano Casaletti and Guido Valerio and Oscar Quevedo-Teruel and Paolo Burghignoli}, title = {An overview of metasurfaces for thin antenna applications}, journal = {Comptes Rendus. Physique}, pages = {659--676}, publisher = {Acad\'emie des sciences, Paris}, volume = {21}, number = {7-8}, year = {2020}, doi = {10.5802/crphys.20}, language = {en}, }
TY - JOUR AU - Massimiliano Casaletti AU - Guido Valerio AU - Oscar Quevedo-Teruel AU - Paolo Burghignoli TI - An overview of metasurfaces for thin antenna applications JO - Comptes Rendus. Physique PY - 2020 SP - 659 EP - 676 VL - 21 IS - 7-8 PB - Académie des sciences, Paris DO - 10.5802/crphys.20 LA - en ID - CRPHYS_2020__21_7-8_659_0 ER -
%0 Journal Article %A Massimiliano Casaletti %A Guido Valerio %A Oscar Quevedo-Teruel %A Paolo Burghignoli %T An overview of metasurfaces for thin antenna applications %J Comptes Rendus. Physique %D 2020 %P 659-676 %V 21 %N 7-8 %I Académie des sciences, Paris %R 10.5802/crphys.20 %G en %F CRPHYS_2020__21_7-8_659_0
Massimiliano Casaletti; Guido Valerio; Oscar Quevedo-Teruel; Paolo Burghignoli. An overview of metasurfaces for thin antenna applications. Comptes Rendus. Physique, Volume 21 (2020) no. 7-8, pp. 659-676. doi : 10.5802/crphys.20. https://comptes-rendus.academie-sciences.fr/physique/articles/10.5802/crphys.20/
[1] Roadmap on metasurfaces, J. Opt., Volume 21 (2019) no. 7, 073002
[2] Frequency Selective Surfaces Theory and Design, Wiley Interscience, New York, 1995
[3] Electromagntic Metamaterials: Physics and Engineering Aspects, IEE-Wiley, New York, 2006
[4] Effective surface impedance of a printed-circuit tensor impedance surface (pctis), IEEE Trans. Microw. Theory Tech., Volume 61 (2013) no. 4, pp. 1403-1413
[5] An overview of the theory and applications of metasurfaces: the two-dimensional equivalents metamaterials, IEEE Trans. Antennas Propag., Volume 54 (2012) no. 2, pp. 10-35
[6] Metasurfaces and their applications, Nanophotonics, Volume 7 (2018) no. 6, pp. 989-1011
[7] Fundamentals and applications of metasurfaces, Small Methods, Volume 1 (2017) no. 4, 1600064
[8] Analysis and Design of Reflectarrays/Transmitarrays Antennas, Lambert Academic Publishing, Saarbrücken, 2014
[9] High-impedance electromagnetic surfaces with a forbidden frequency band, IEEE Trans. Microw. Theory Tech., Volume 47 (1999) no. 11, pp. 2059-2074
[10] A wideband low-profile tightly coupled antenna array with a very high figure of merit, IEEE Trans. Antennas Propag., Volume 67 (2019) no. 4, pp. 2332-2343
[11] TE surface wave resonances on high-impedance surface based antennas: analysis and modeling, IEEE Trans. Antennas Propag., Volume 59 (2011) no. 10, pp. 3588-3596
[12] On the bandwidth of high-impedance frequency selective surfaces, IEEE Antennas Wirel. Propag. Lett., Volume 8 (2009), pp. 1341-1344
[13] Fundamental properties of surface waves in lossless stratified structures, Proc. R. Soc. A, Volume 466 (2010) no. 2120, pp. 2447-2469
[14] Design of a broadband dipole in close proximity to an EBG ground plane, IEEE Antennas Propag. Mag., Volume 50 (2008) no. 6, pp. 52-64
[15] Optimal design of dipole antennas backed by a finite high-impedance screen, Prog. Electromagn. Res. C, Volume 18 (2011), pp. 137-151
[16] Antenna miniaturization and bandwidth enhancement using a reactive impedance substrate, IEEE Trans. Antennas Propag., Volume 52 (2004) no. 9, pp. 2403-2414
[17] Reflection phase characterizations of the EBG ground plane for low profile wire antenna applications, IEEE Trans. Antennas Propag., Volume 51 (2003) no. 10, pp. 2691-2703
[18] Aperture-coupled patch antenna on UC-PBG substrate, IEEE Trans. Microw. Theory Tech., Volume 47 (1999) no. 11, pp. 2123-2130
[19] Microstrip antennas integrated with electromagnetic band-gap (EBG) structures: a low mutual coupling design for array applications, IEEE Trans. Antennas Propag., Volume 51 (2003) no. 10, pp. 2936-2946
[20] An application of high-impedance ground planes to phased array antennas, IEEE Trans. Antennas Propag., Volume 53 (2005) no. 4, pp. 1377-1381
[21] Investigation of using high impedance surfaces for wide-angle scanning arrays, IEEE Trans. Antennas Propag., Volume 63 (2015) no. 7, pp. 2895-2901
[22] Planar soft surfaces and their application to mutual coupling reduction, IEEE Trans. Antennas Propag., Volume 57 (2009) no. 12, pp. 3852-3859
[23] Electromagnetic Band Gap Structures in Antenna Engineering, Cambridge University Press, Cambridge, UK, 2009
[24] A novel compact spiral electromagnetic band-gap (EBG) structure, IEEE Trans. Antennas Propag., Volume 56 (2008) no. 6, pp. 1656-1660
[25] Wideband smaller unit-cell planar EBG structures and their application, IEEE Trans. Antennas Propag., Volume 56 (2008) no. 3, pp. 903-908
[26] Low profile fully planar folded dipole antenna on a high impedance surface, IEEE Trans. Antennas Propag., Volume 60 (2012) no. 1, pp. 51-62
[27] Innovation and intellectual property rights, Metamaterials: Physics and Engineering Explorations, Whiley, New York, USA, 2006, pp. 377-402
[28] Thickness reduction and performance enhancement of steerable square loop antenna using hybrid high impedance surface, IEEE Trans. Antennas Propag., Volume 58 (2010) no. 5, pp. 1477-1485
[29] Optimum high impedance surface configuration for mutual coupling reduction in small antenna arrays, Prog. Electromagn. Res. B, Volume 32 (2011), pp. 283-297
[30] Circular high-impedance surfaces characterization, IEEE Antennas Wirel. Propag. Lett., Volume 11 (2012), pp. 260-263
[31] Analysis, design and measurements of circularly symmetric high-impedance surfaces for loop antenna applications, IEEE Trans. Antennas Propag., Volume 64 (2016) no. 2, pp. 618-629
[32] Gain and bandwidth enhancement of a spiral antenna using a circularly symmetric HIS, IEEE Antennas Wirel. Propag. Lett., Volume 16 (2017), pp. 1080-1083
[33] Metasurfaces with interleaved conductors: phenomenology and applications to frequency selective and high impedance surfaces, IEEE Trans. Antennas Propag., Volume 64 (2016) no. 2, pp. 599-608
[34] Circuit model of a double-layer artificial magnetic conductor, IEEE Antennas Wirel. Propag. Lett., Volume 15 (2016), pp. 1061-1064
[35] The design synthesis of multiband artificial magnetic conductors using high impedance frequency selective surfaces, IEEE Trans. Antennas Propag., Volume 53 (2005) no. 1, pp. 8-17
[36] A novel multi-band electromagnetic band-gap structure, Prog. Electromagn. Res. Lett., Volume 9 (2009), pp. 67-74
[37] Dual-band artificial magnetic conductor, Appl. Phys. A, Volume 109 (2012), pp. 1075-1080
[38] Study of a low-profile 2.4-GHz planar dipole antenna using a high-impedance surface with 1-D varactor tuning, IEEE Trans. Antennas Propag., Volume 61 (2013) no. 2, pp. 506-515
[39] Partially reflecting sheet arrays, IRE Trans. Antennas Propag., Volume 4 (1956) no. 4, pp. 666-671
[40] High gain planar antenna using optimised partially reflective surfaces, IEE Proc.-Microw. Antennas Propag., Volume 148 (2001) no. 6, pp. 345-350
[41] Directivity of an antenna embedded inside a Fabry–Perot cavity: analysis and design, Microw. Opt. Technol. Lett., Volume 48 (2006) no. 1, pp. 12-17
[42] Investigation into the effects of the patch-type FSS superstrate on the high-gain cavity resonance antenna design, IEEE Trans. Antennas Propag., Volume 58 (2010) no. 2, pp. 258-270
[43] Gain enhancement methods for printed circuit antennas, IEEE Trans. Antennas Propag., Volume 33 (1985) no. 9, pp. 976-987
[44] A leaky-wave analysis of the high-gain printed antenna configuration, IEEE Trans. Antennas Propag., Volume 36 (1988) no. 7, pp. 905-910
[45] 2-d periodic leaky-wave antennas—Part I: metal patch design, IEEE Trans. Antennas Propag., Volume 53 (2005) no. 11, pp. 3505-3514
[46] 2-d periodic leaky-wave antennas—Part II: slot design, IEEE Trans. Antennas Propag., Volume 53 (2005) no. 11, pp. 3515-3524
[47] General formulas for 2-D leaky-wave antennas, IEEE Trans. Antennas Propag., Volume 53 (2005) no. 11, pp. 3525-3533
[48] Fundamental properties and optimization of broadside radiation from uniform leaky-wave antennas, IEEE Trans. Antennas Propag., Volume 54 (2006) no. 5, pp. 1442-1452
[49] Radiation from cylindrical leaky waves, IEEE Trans. Antennas Propag., Volume 38 (1990) no. 4, pp. 482-488
[50] Low-profile resonant cavity antenna with artificial magnetic conductor ground plane, Electron. Lett., Volume 40 (2004) no. 7, pp. 405-406
[51] Artificial magnetic conductor surfaces and their application to low-profile high-gain planar antennas, IEEE Trans. Antennas Propag., Volume 53 (2005) no. 1, pp. 209-215
[52] High-gain subwavelength resonant cavity antennas based on metamaterial ground planes, IEE Proc.-Microw. Antennas Propag., Volume 153 (2006) no. 1, pp. 1-6
[53] Directive emissions from subwavelength metamaterial-based cavities, Appl. Phys. Lett., Volume 86 (2005) no. 10, 101101
[54] All-metamaterial-based subwavelength cavities (60) for ultrathin directive antennas, Appl. Phys. Lett., Volume 88 (2006) no. 8, 084103
[55] A broadband high-gain resonant cavity antenna with single feed, 2006 First European Conference on Antennas and Propagation, IEEE, 2006, pp. 1-5
[56] Bandwidth enhancement of 2-D leaky-wave antennas with double-layer periodic surfaces, IEEE Trans. Antennas Propag., Volume 62 (2014) no. 2, pp. 586-593
[57] Multilayer partially reflective surfaces for broadband Fabry–Perot cavity antennas, IEEE Trans. Antennas Propag., Volume 62 (2014) no. 7, pp. 3474-3481
[58] Broadband high gain compact resonator antennas using combined FSS, 2008 IEEE Antennas Propagation Society International Symposium, IEEE, San Diego, CA, USA, 2008, pp. 1-4
[59] The use of simple thin partially reflective surfaces with positive reflection phase gradients to design, wideband, low-profile EBG resonator antennas, IEEE Trans. Antennas Propag., Volume 60 (2012) no. 2, pp. 743-750
[60] EBG structure with wide defect band for broadband cavity antenna applications, IEEE Antennas Wirel. Propag. Lett., Volume 7 (2008), pp. 693-696
[61] Wideband high-gain EBG resonator antennas with small footprints and all-dielectric superstructures, IEEE Trans. Antennas Propag., Volume 62 (2014) no. 6, pp. 2970-2977
[62] Compact high-gain antenna with simple all-dielectric partially reflecting surface, IEEE Trans. Antennas Propag., Volume 66 (2018) no. 8, pp. 4343-4348
[63] Leaky-wave explanation of gain-bandwidth-enhanced Fabry–Perot cavity antennas formed by a thick multilayer partially-reflective surface, 2015 IEEE International Symposium on Antennas Propagation & USNC/URSI National Radio Science Meeting, IEEE, Vancouver, BC, Canada, 2015, pp. 1090-1091
[64] Leaky-wave analysis of wideband planar Fabry–Perot cavity antennas formed by a thick PRS, IEEE Trans. Antennas Propag., Volume 67 (2019) no. 8, pp. 5163-5175
[65] Highly polarized directive radiation from a Fabry–Pérot cavity leaky-wave antenna based on a metal strip grating, IEEE Trans. Antennas Propag., Volume 58 (2010) no. 12, pp. 3873-3883
[66] Omnidirectional 2-D leaky-wave antennas with reconfigurable polarization, IEEE Antennas Wirel. Propag. Lett., Volume 16 (2017), pp. 2354-2357
[67] A steerable leaky-wave antenna using a tunable impedance ground plane, IEEE Antennas Wirel. Propag. Lett., Volume 1 (2002), pp. 179-182
[68] Forward and backward leaky wave radiation with large effective aperture from an electronically tunable textured surface, IEEE Trans. Antennas Propag., Volume 53 (2005) no. 1, pp. 236-247
[69] Electronically reconfigurable metamaterial for compact directive cavity antennas, Electron. Lett., Volume 43 (2007) no. 13, pp. 698-700
[70] An active high-impedance surface for low-profile tunable and steerable antennas, IEEE Antennas Wirel. Propag. Lett., Volume 7 (2008), pp. 676-680
[71] Design of subwavelength tunable and steerable Fabry–Perot/leaky wave antennas, Prog. Electromagn. Res., Volume 111 (2011), pp. 467-481
[72] A Fabry–Pérot antenna with two-dimensional electronic beam scanning, IEEE Trans. Antennas Propag., Volume 64 (2016) no. 4, pp. 1536-1541
[73] A reconfigurable high-gain partially reflecting surface antenna, IEEE Trans. Antennas Propag., Volume 56 (2008) no. 11, pp. 3382-3390
[74] Graphene Fabry–Pérot cavity leaky-wave antennas: plasmonic versus nonplasmonic solutions, IEEE Trans. Antennas Propag., Volume 65 (2017) no. 4, pp. 1651-1660
[75] A tunable ferroelectric antenna for fixed-frequency scanning applications, IEEE Antennas Wirel. Propag. Lett., Volume 5 (2006), pp. 353-356
[76] Tunable Fabry–Pérot cavity THz antenna based on leaky-wave propagation in nematic liquid crystals, IEEE Antennas Wirel. Propag. Lett., Volume 16 (2017), pp. 2046-2049
[77] Reconfigurable terahertz leaky-wave antenna using graphene-based high-impedance surface, IEEE Trans. Nanotechnol., Volume 14 (2015) no. 1, pp. 62-69
[78] Complex mode spectra of graphene-based planar structures for THz applications, J. Infrared Millimeter Terahertz Waves, Volume 36 (2015) no. 8, pp. 720-733
[79] A reconfigurable substrate–superstrate graphene-based leaky-wave THz antenna, IEEE Antennas Wirel. Propag. Lett., Volume 15 (2016), pp. 1545-1548
[80] Generation of propagating Bessel beams using leaky-wave modes, IEEE Trans. Antennas Propag., Volume 60 (2012) no. 8, pp. 3605-3613
[81] Higher-order leaky-mode Bessel-beam launcher, IEEE Trans. Antennas Propag., Volume 64 (2016) no. 3, pp. 904-913
[82] A new approach to Bessel beams, J. Modern Opt., Volume 46 (1999) no. 6, pp. 923-930
[83] Generation of non-diffractive Bessel beams by inward cylindrical traveling wave aperture distributions, Opt. Express, Volume 22 (2014) no. 15, pp. 18354-18364
[84] Large depth of field pseudo–Bessel beam generation with a RLSA antenna, IEEE Trans. Antennas Propag., Volume 62 (2014) no. 8, pp. 3911-3919
[85] Experimental validation of Bessel beam generation using an inward Hankel aperture distribution, IEEE Trans. Antennas Propag., Volume 63 (2015) no. 6, pp. 2539-2544
[86] Transverse circular-polarized bessel beam generation by inward cylindrical aperture distribution, Opt. Express, Volume 24 (2016) no. 10, pp. 11103-11111
[87] Generation of spatial Bessel beams using holographic metasurface, Opt. Express, Volume 23 (2015) no. 6, pp. 7593-7601
[88] Radially periodic leaky-wave antenna for Bessel beam generation over a wide-frequency range, IEEE Trans. Antennas Propag., Volume 66 (2018) no. 6, pp. 2828-2843
[89] Focusing leaky waves: a class of electromagnetic localized waves with complex spectra, Phys. Rev. A, Volume 9 (2018) no. 5, 054005
[90] Holographic surface leaky-wave lenses with circularly-polarized focused near-fields—Part I: concept, design and analysis theory, IEEE Trans. Antennas Propag., Volume 61 (2013) no. 7, pp. 3475-3485
[91] Holographic surface leaky-wave lenses with circularly-polarized focused near-fields—Part II: experiments and description of frequency steering of focal length, IEEE Trans. Antennas Propag., Volume 61 (2013) no. 7, pp. 3486-3494
[92] A novel printed leaky-wave ‘bull-eye’ antenna with suppressed surface-wave excitation, IEEE Antennas Propagation Society Symposium, Volume 1, IEEE, Monterey, CA, USA, 2004, pp. 1078-1081
[93] Planar antenna for continuous beam scanning and broadside radiation by selective surface wave suppression, Electron. Lett., Volume 46 (2010) no. 9, pp. 613-614
[94] Analysis and design of annular microstrip-based planar periodic leaky-wave antennas, IEEE Trans. Antennas Propag., Volume 62 (2014) no. 6, pp. 2978-2991
[95] 77-GHz high-gain bull’s-eye antenna with sinusoidal profile, IEEE Antennas Wirel. Propag. Lett., Volume 14 (2015), pp. 205-208
[96] Terahertz corrugated and bull’s-eye antennas, IEEE Trans. Terahertz Science and Technol., Volume 3 (2013) no. 6, pp. 740-747
[97] Scalar and tensor holographic artificial impedance surfaces, IEEE Trans. Antennas Propag., Volume 58 (2010) no. 10, pp. 3212-3221
[98] Spiral leaky-wave antennas based on modulated surface impedance, IEEE Trans. Antennas Propag., Volume 59 (2011) no. 12, pp. 4436-4444
[99] A printed leaky-wave antenna based on a sinusoidally-modulated reactance surface, IEEE Trans. Antennas Propag., Volume 59 (2011) no. 6, pp. 2087-2096
[100] Polarized beams using scalar metasurfaces, IEEE Trans. Antennas Propag., Volume 64 (2016) no. 8, pp. 3391-3400
[101] The effects of spatial dispersion on power flow along a printed-circuit tensor impedance surface, IEEE Trans. Antennas Propag., Volume 62 (2014) no. 3, pp. 1464-1469
[102] Flat optics for surface waves, IEEE Trans. Antennas Propag., Volume 64 (2016) no. 1, pp. 155-166
[103] Synthesis of modulated-metasurface antennas with amplitude phase and polarization control, IEEE Trans. Antennas Propag., Volume 64 (2016) no. 9, pp. 3907-3919
[104] Implementation of radiating aperture field distribution using tensorial metasurfaces, IEEE Trans. Antennas Propag., Volume 65 (2017) no. 11, pp. 5895-5907
[105] Flat optics for leaky-waves on modulated metasurfaces: adiabatic floquet-wave analysis, IEEE Trans. Antennas Propag., Volume 64 (2016) no. 9, pp. 3896-3906
[106] Efficiency of metasurface antennas, IEEE Trans. Antennas Propag., Volume 65 (2017) no. 4, pp. 1532-1541
[107] Bandwidth of gain in metasurface antennas, IEEE Trans. Antennas Propag., Volume 65 (2017) no. 6, pp. 2836-2842
[108] Guided waves on scalar and tensorial reactance surfaces modulated by periodic functions: a circuital approach, IEEE Access, Volume 7 (2019), pp. 68823-68836
[109] Design of scalar impedance holographic metasurfaces for antenna beam formation with desired polarization, IEEE Trans. Antennas Propag., Volume 63 (2015) no. 7, pp. 3016-3024
[110] Multiwavelength multiplexing hologram designed using impedance metasurfaces, IEEE Trans. Antennas Propag., Volume 66 (2018) no. 11, pp. 6408-6413
[111] Polarization-insensitive holographic surfaces with broadside radiation, IEEE Trans. Antennas Propag., Volume 64 (2016) no. 12, pp. 5272-5280
[112] Experimental validation of a Ku-band dual-circularly polarized metasurface antenna, IEEE Trans. Antennas Propag., Volume 66 (2018) no. 3, pp. 1153-1159
[113] Design of a LP, RHCP and LHCP polarization-reconfigurable holographic antenna, IEEE Access, Volume 7 (2019), pp. 82776-82784
[114] Polarization-diverse holographic metasurfaces, IEEE Antennas Wirel. Propag. Lett., Volume 18 (2019) no. 2, pp. 264-268
[115] A quasi-direct method for the surface impedance design of modulated metasurface antennas, IEEE Trans. Antennas Propag., Volume 67 (2019) no. 1, pp. 24-36
[116] Realization and measurement of broadside beam modulated metasurface antennas, IEEE Antennas Wirel. Propag. Lett., Volume 15 (2016), pp. 610-613
[117] Tensorial metasurface antennas radiating polarized beams based on aperture field implementation, Int. J. Microw. Wirel. Technol., Volume 10 (2018) no. 2, pp. 161-168
[118] A circularly-polarized isoflux antenna based on anisotropic metasurface, IEEE Trans. Antennas Propag., Volume 60 (2012) no. 11, pp. 4998-5009
[119] Multibeam by metasurface antennas, IEEE Trans. Antennas Propag., Volume 65 (2017) no. 6, pp. 2923-2930
[120] Experimental validation of tensorial metasurfaces for the implementation of radiating aperture field distributions, IEEE Trans. Antennas Propag., Volume 67 (2019) no. 7, pp. 4901-4906
[121] Near-field multi-beam generation by tensorial metasurfaces, IEEE Trans. Antennas Propag., Volume 67 (2019) no. 9, pp. 6068-6075
[122] Metasurfing: addressing waves on impenetrable metasurfaces, IEEE Antennas Wirel. Propag. Lett., Volume 10 (2011), pp. 1499-1502
[123] A two-dimensional microwave Luneberg lens, Trans. IRE Professional Group on Antennas Propag., Volume 1 (1953) no. 1, pp. 12-23
[124] A plate Luneberg lens with the permittivity distribution controlled by hole density, Electron. Commun. Jpn. (Part I: Communications), Volume 85 (2002) no. 9, pp. 1-12
[125] 24 ghz automotive radar planar Luneburg lens, IET Microw. Antennas Propag., Volume 1 (2007) no. 3, pp. 624-628
[126] Lens antennas for 5G communications systems, IEEE Commun. Mag., Volume 56 (2018) no. 7, pp. 36-41
[127] A solution of the problem of rapid scanning for radar antennae, J. Appl. Phys., Volume 19 (1948) no. 9, pp. 860-862
[128] Electromagnetic characterization of textured surfaces formed by metallic pins, IEEE Trans. Antennas Propag., Volume 56 (2008) no. 2, pp. 405-415
[129] Angular independency of a parallel-plate luneburg lens with hexagonal lattice and circular metal posts, IEEE Antennas Wirel. Propag. Lett., Volume 1 (2002), pp. 128-130
[130] Surface-wave Luneberg lens antennas, IRE Trans. Antennas Propag., Volume 8 (1960) no. 5, pp. 508-515
[131] Ultrawideband metasurface lenses based on off-shifted opposite layers, IEEE Antennas Wirel. Propag. Lett., Volume 15 (2016), pp. 484-487
[132] Simple and accurate analytical model of planar grids and high-impedance surfaces comprising metal strips or patches, IEEE Trans. Antennas Propag., Volume 56 (2008) no. 6, pp. 1624-1632
[133] Printed holey plate Luneburg lens, Microw. Opt. Technol. Lett., Volume 50 (2008) no. 2, pp. 378-380
[134] Parallel-plate-waveguide Luneburg lens through a holey plate metasurface, 2015 9th European Conference on Antennas Propagation (EuCAP), IEEE, 2015, pp. 1-2
[135] Metasurfing: addressing waves on impenetrable metasurfaces, IEEE Antennas Wirel. Propag. Lett., Volume 10 (2011), pp. 1499-1502
[136] Implementation of transformed lenses in bed of nails reducing refractive index maximum value and sub-unity regions, Opt. Lett., Volume 40 (2015) no. 6, pp. 926-929
[137] Multi-beam multi-layer leaky-wave SIW pillbox antenna for millimeter-wave applications, IEEE Trans. Antennas Propag., Volume 59 (2011) no. 4, pp. 1093-1100
[138] broadband Luneburg lens antenna, IEEE Trans. Antennas Propag., Volume 58 (2010) no. 9, pp. 3055-3059
[139] Low-dispersive leaky-wave antenna integrated in groove gap waveguide technology, IEEE Trans. Antennas Propag., Volume 66 (2018) no. 11, pp. 5727-5736
[140] Non-uniform metasurface Luneburg lens antenna design, IEEE Trans. Antennas Propag., Volume 60 (2012) no. 9, pp. 4065-4073
[141] Glide-symmetric fully metallic Luneburg lens for 5G communications at Ka-band, IEEE Antennas Wirel. Propag. Lett., Volume 17 (2018) no. 9, pp. 1588-1592
[142] Cost-effective gap waveguide technology based on glide-symmetric holey EBG structures, IEEE Trans. Microw. Theory Tech., Volume 66 (2018) no. 2, pp. 927-934
[143] Using glide-symmetric holes to reduce leakage between waveguide flanges, IEEE Microw. Wirel. Components Lett., Volume 28 (2018) no. 6, pp. 473-475
[144] Holey glide-symmetric filters for 5G at millimeter-wave frequencies, IEEE Microw. Wirel. Components Lett., Volume 30 (2020) no. 1, pp. 31-34
[145] Glide-symmetric all-metal holey metasurfaces for low-dispersive artificial materials: modeling and properties, IEEE Trans. Microw. Theory Tech., Volume 66 (2018) no. 7, pp. 3210-3223
[146] Analyzing glide-symmetric holey metasurfaces using a generalized Floquet theorem, IEEE Access, Volume 6 (2018), pp. 71743-71750
[147] Mimicking glide symmetry dispersion with coupled slot metasurfaces, Appl. Phys. Lett., Volume 111 (2017) no. 12, 121603
[148] Ultrawideband anisotropic glide-symmetric metasurfaces, IEEE Antennas Wirel. Propag. Lett., Volume 18 (2019) no. 8, pp. 1547-1551
[149] Wide-angle impedance matching using glide-symmetric metasurfaces, IEEE Microw. Wirel. Compon. Lett., Volume 30 (2020) no. 1, pp. 8-11
Cité par Sources :
Commentaires - Politique