1 Introduction
Desulfurization of fuel oil has attracted much attention in recent years because of the stringent demand of environmental regulations. Among the desulfurization techniques, oxidative desulfurization (ODS) is considered as one of the most promising processes to remedy the shortages of industrial hydrodesulfurization technique, that is, difficult to remove aromatic sulfur-containing compounds such as dibenzothiophene (DBT) and 4,6-dimethyldibenzothiophene (4,6-DMDBT) in fuel oil. Moreover, the ODS process can be carried out at atmospheric pressure and low temperatures without costly hydrogen consumption [1–3]. Thus, there has been a great interest in developing ODS catalysts with high desulfurization efficiency.
Currently, various types of catalysts have been attempted for ODS of fuel oil. There into, homogeneous catalysts such as organic acids or heteropolyacid salts have been demonstrated to be active in ODS reactions [4]. For example, Otsuki et al. [5] reported that formic acid could promote the oxidation of DBT into corresponding sulfone with a yield of 40% at 50 °C in 3 h. Al-Shahrani et al. [6] showed that DBT or 4,6-DMDBT could be efficiently removed at 70 °C in 1 h in the presence of Na2WO4 and CH3COOH. In addition, heterogeneous catalysts like Ti-containing porous materials have also been widely studied [7]. For instances, microporous titanosilicates involving TS-1 (titanosilicate-1) with MFI topology [8], Ti-MWW with MWW topology [9], and Ti-Beta with BEA topology [10] displayed good catalytic performance for the oxidation of thiophene. However, they almost had no catalytic reactivity in the ODS reactions of sulfur compounds with relatively large molecular size like DBT or 4,6-DMDBT mainly because of the inaccessibility of active sites located in micropores. Recently, many efforts have been devoted to preparing Ti-containing mesoporous silica and hierarchical zeolites. Because of the introduction of mesopores, the ability of mass transfer could be improved. As a result, these materials exhibited superior catalytic performance in the ODS reactions of DBT and 4,6-DMDBT [11–13]. Nevertheless, such good ODS performance was generally based on the reaction temperature in the range of 40–80 °C. It is still rare that the catalysts can exhibit highly efficient ODS activity at room temperature.
In this study, we present that a series of catalysts (tetra-alkyl orthotitanates, TAOTs) displayed highly active ODS performance for the removal of DBT at room temperature and ambient pressure. Meanwhile, the formed sulfones (DBTO2) can be easily separated from model oil without extraction process. The effects of some factors such as alkyl groups in TAOTs, oxidant/sulfur (O/S) molar ratio, and water content in model oil on the catalytic performance were investigated in detail and a possible reaction mechanism was proposed.
2 Experimental section
2.1 Materials
Tetra-butyl orthotitanate (TBOT), tetra-ethyl orthotitanate, tetra-isopropyl orthotitanate (TPOT), n-octane, hydrogen peroxide (30% aqueous solution), tert-butyl hydroperoxide (TBHP), and cumene hydroperoxide (CHP) were purchased from Sinopharm. Benzothiophene (BT) and DBT were purchased from Acros Organics. All of the chemicals were used as obtained without purification.
2.2 Catalytic test
A model oil with 1000 ppmw S was prepared by dissolving 84 mg BT or 115 mg DBT in 20 g n-octane. The reaction was performed in a 100 mL three-neck glass flask equipped with a condenser under vigorous stirring (1000 rpm). In a typical run, 20 g of the model oil and a certain amount of CHP were first added to the reactor. Then, the mixture was stirred for 10 min at room temperature and ambient pressure before 50 μL TAOT was added. This moment of adding TAOT was regarded as the initial reaction time. After the reaction, the model oil was analyzed by gas chromatography (GC) on an Agilent 7890A gas chromatograph with an flame ionization detector (FID) detector using a 30 m packed HP5 column. The products were also identified by GC–mass spectrometry (MS) (7890A-5975C) analysis. The conversion of BT or DBT is defined based on the amount of BT or DBT removed from the model oil. The value of conversion was calculated according to the equation R = (C0 − Ct)/C0 × 100%. C0 and Ct stand for the initial and residual concentration of BT or DBT in n-octane, respectively.
2.3 Characterization
Infrared (IR) spectroscopy was measured using a Thermo Fisher Nicolet iS5 ATR-IR instrument. Raman spectroscopy was recorded using an Xplora PLUS Raman microscope (Horiba Company) with a 532 nm laser. The acquisition time was 10 s and accumulated for five cycles.
3 Results and discussion
3.1 Catalytic performance
In the beginning, TBOT with low cost was chosen as a representative of TAOT catalysts to evaluate its catalytic performance in the ODS reaction of DBT. As seen in Table 1, DBT can be efficiently removed within 10 min at room temperature. After the reaction, a solid product was formed in the model oil, which may be facilely separated by centrifugation. The calculated turnover frequency (TOF) value over TBOT reached 25.5 h−1. To the best of our knowledge, such ODS performance is highly active as compared with the reported homogeneous or heterogeneous ODS catalysts. For example, the typical homogeneous catalysts like formic acid and acetic acid need relatively high reaction temperature (50 °C) and long reaction time (more than 120 min) to complete the oxidation of DBT [1,5]. The TOF values were far less than 0.01 h−1. The well-known heterogeneous catalysts such as Ti–MCM-41, meso–TS-1, and Ti–SBA-2 exhibited superior ODS performance. However, the reaction temperature was generally greater than 40 °C [11–13], and their TOF values were still lower than 20 h−1. These results indicate that it is difficult for one catalyst to obtain highly efficient ODS performance at room temperature and TBOT is indeed a very active ODS catalyst.
Comparison of catalytic performance over some representative catalysts in the ODS reaction of DBT.
Catalysts | Initial sulfur content (ppmw) | Temperature (°C) | Reaction time (min) | Conversion (%) | O/S (molar ratio) | TOFa (h−1) | Reference |
Acetic acid | 500 | 50 | 120 | 100 | 895 | 6.5 × 10−4 | [1] |
Formic acid | 227 | 50 | 180 | 40 | 160 | 5.1 × 10−4 | [5] |
Ti-Beta | 584 | 70 | 300 | 91 | 20 | 5.0b | [10] |
Ti–MCM-41 | 1740 | 80 | 100 | 100 | 6 | 18.2b | [11] |
meso–TS-1 | 1000 | 60 | 60 | 100 | 2 | 3.7b | [12] |
Ti–SBA-2 | 87 | 40 | 20 | >99 | 3 | 6.4b | [13] |
Amorphous TiO2 | 500 | 40 | 90 | >99 | 3 | 0.6 | [25] |
TBOT | 1000 | 25 | <10 | >99 | 6 | 25.5 | This work |
a TOF was calculated based on mole number of S converted by unit mole Ti per hour.
b Ti content was calculated according to the molar ratio of Si/Ti.
To determine the composition of the model oil before and after reaction, the GC–MS technique was used (Fig. 1 and supplementary Figs. S1 and S2). Before reaction, only one GC peak corresponding to DBT was observed. After reaction, the peak almost disappeared. Strangely, the GC peak assigned to DBTO2 was not detected in the oil phase. This result suggested that the formed DBTO2 might be transferred into the separated solid. To verify this point, the solid was washed with acetonitrile, and then the acetonitrile solution was analyzed by GC–MS. As seen in Fig. 1, an apparent GC signal belonging to DBTO2 was observed. This result demonstrated that the formed DBTO2 together with the solid product could be separated from the oil phase by centrifugation or filtration. This means that the step to remove DBTO2 generally by extraction process after reaction was no longer needed when TBOT was used as a catalyst.

GC analysis of model oil before (0 min) and after reaction (10 min) and acetonitrile solution after washing the separated solid.
3.2 Effect of alkyl groups in TAOTs
To test the catalytic reactivity of TBOT homologues, the ODS reaction of DBT over TAOTs with various alkyl groups was conducted. As shown in Fig. 2, both tetra-ethyl orthotitanate and TPOT also exhibited highly efficient ODS performance. The removal content of DBT over both catalysts can reach more than 98% within 10 min at room temperature, revealing that TAOTs should be a series of highly active catalysts in the ODS reaction of DBT.
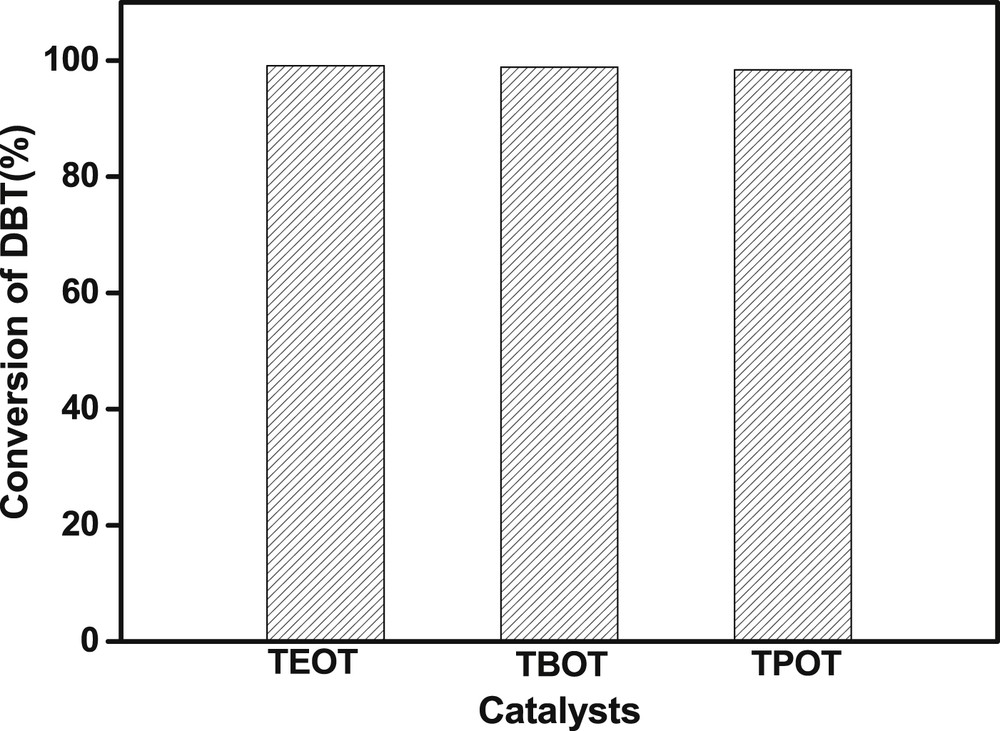
Catalytic reactivity of TAOTs with various alkyl groups. Reaction conditions: S content of 1000 ppmw, model oil of 20 g, O/S = 6, time = 10 min, room temperature. TEOT, tetra-ethyl orthotitanate.
3.3 Effect of various oxidants
The effect of various oxidants on catalytic performance was investigated. When using H2O2 and TBHP as oxidants, the conversions of DBT were 1% and 17% (Fig. 3), respectively. These values were much lower than that of using CHP as the oxidant. Such a considerable difference might result from the different water contents in various oxidants. Among these three oxidants, the water contents in H2O2 and TBHP reagents are 70% and 30%, respectively. Comparatively, the water content in CHP reagent is less than 1%. In the presence of water, TBOT tends to hydrolyze into amorphous titania. This process would destroy the pristine coordination state of TBOT and thus decrease its reactivity. This is the possible reason why the catalytic performance of TBOT by CHP as the oxidant was much better than that by H2O2 or TBHP as the oxidant. Moreover, the aqueous solution of oxidant is immiscible with the oil phase, which would lead to a biphasic reaction system and increase the obstacle of mass transfer between different phases, thus decreasing the reaction rate.
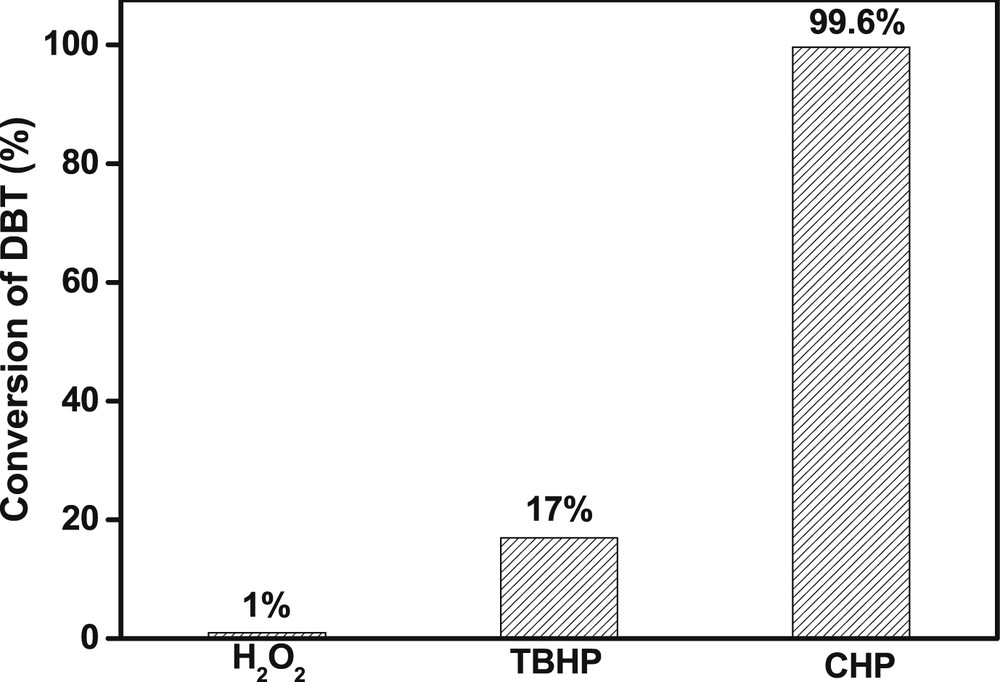
Effect of oxidants on the catalytic performance of TBOT. Reaction conditions: S content of 1000 ppmw, model oil of 20 g, O/S = 6, time = 10 min, room temperature.
3.4 Effect of O/S molar ratio
In ODS reactions, the O/S molar ratio is an influencing factor of ODS activity. Hence, the effect of different O/S molar ratios on the catalytic performance of TBOT was studied at room temperature (Fig. 4a). The results showed that the desulfurization efficiency of TBOT was improved with the increase in the O/S molar ratio. When the O/S molar ratios were 2:1 and 4:1, the removal contents of DBT were about 70% and 90%, respectively. With further increase in the O/S molar ratio to 6:1, the desulfurization efficiency reached 99%. These results indicated that low O/S molar ratio had a negative effect on ODS performance and the O/S molar ratio of 6:1 could be suitable for the current reaction system.

Effect of O/S molar ratio (a) and the water content (b) on the catalytic performance of TBOT Reaction conditions: S content of 1000 ppmw, model oil of 20 g, time = 10 min, room temperature.
3.5 Effect of water content
As known, a small amount of water exists in commercial fuel oil, which may weaken the ODS reactivity of catalysts by covering the active sites. Therefore, the catalytic reactivity of TBOT was also evaluated under the condition of adding a small amount of water into the model oil. The water content was adjusted from 0.03% to 0.07% (v/v). It can be seen from Fig. 4b that the removal content of DBT decreased with the increase in water content. When the water content was 0.03%, the removal content of DBT was 90%. With the increase in water content to 0.07%, the removal content of DBT was decreased to 80%. These results indicated that the presence of water in fuel oil should not be beneficial for the removal of DBT under this reaction system. However, it was noted that TBOT could still work in the presence of a small amount of water.
3.6 Effect of sulfur compound
To investigate the effect of sulfur compound on the catalytic performance of TBOT, the ODS reaction of BT was carried out (Fig. 5). Under similar reaction conditions, the conversion of BT reached 41%, which was much lower than that of DBT. This result indicated that the oxidation of BT was relatively difficult possibly because the electron density of sulfur atom in BT was lower than that in DBT. This result is in agreement with the reported results [5].
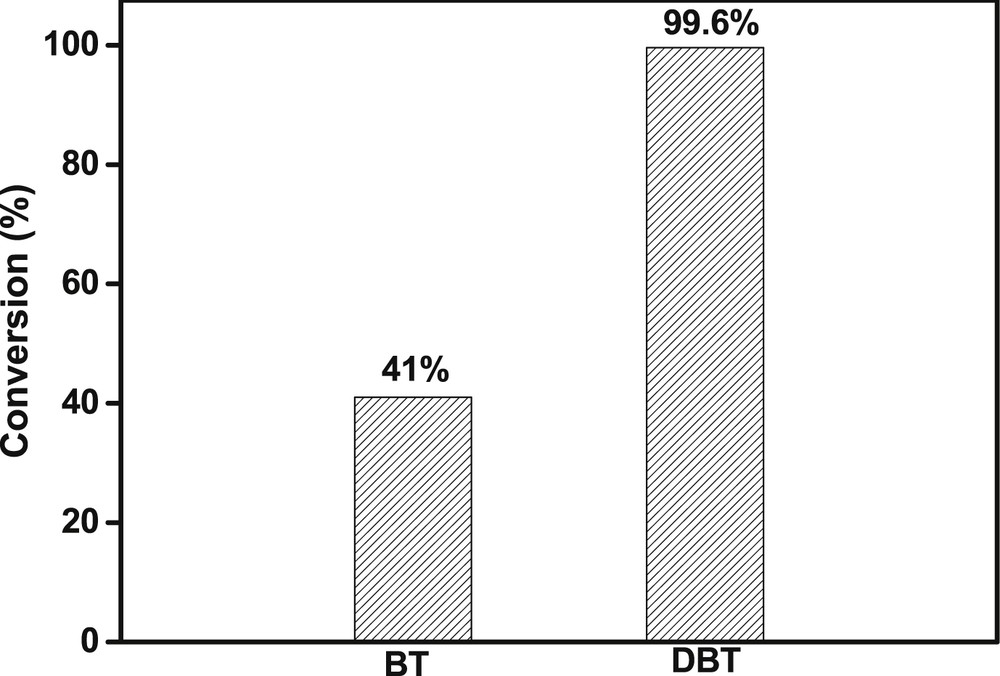
Effect of sulfur compound on the catalytic performance of TBOT. Reaction conditions: S content of 1000 ppmw, model oil of 20 g, O/S = 6, time = 10 min, room temperature.
3.7 Proposed reaction mechanism
It is well known that transition metal Ti sites can form peroxotitanium complexes in the presence of hydroperoxides [14,15]. During the ODS reactions, it has been demonstrated that peroxotitanium intermediates usually play an essential role in catalysis [16–18]. On the basis of these facts, it was supposed that TAOT first interacted with CHP to form peroxotitanium complexes that could oxidize DBT into the corresponding sulfones (Scheme 1). The structure of peroxotitanium species has been proposed by Dewkar et al. [19] who synthesized titanium superoxide by the reaction of TBOT/TPOT and H2O2. The peroxotitanium complexes might be converted into titanium dinuclear (Ti2O5)2+ species during the oxidation reactions [20,21]. It is different from the reported tetra-coordinated titanium catalysts like TS-1 in which the Ti species are generally bound by the formation of TiOAl or TiOSi bond. The Ti species in TAOTs seem to be free so that they are easy to form peroxotitanium complexes that could be catalytically active.

Proposed reaction route of DBT in the model oil by CHP as oxidant over TAOT.
In addition, it was noted from the photograph of model oil that the color of the solution was changed from colorless to yellowish after TBOT was added, which was in accordance with the color of peroxotitanium ester. Then, this solution became muddy as the reaction proceeded. Finally, the solution with a yellow-white solid was formed. After the solid was separated from the liquid by centrifugation, the solution became clear and colorless. According to the earlier report, it is known that the solution color of peroxotitanium complexes is yellow [19]. Most possibly, this solid should be composed of peroxotitanium complexes and then form titanium dinuclear (Ti2O5)2+ species. Ma et al. [22] reported that DBTO2 had high negative electrostatic potential located on the two oxygen atoms. Thus, the formed sulfone DBTO2 could be easily absorbed on the surface of (Ti2O5)2+ species by electrostatic interaction. This is the possible reason why the GC peak corresponding to DBTO2 in the oil phase after reaction was not detected in this work (Fig. 1). After washing the solid product with acetonitrile, DBTO2 partially dissolved in acetonitrile phase according to “like dissolves like” principle.
Further characterization results can partially support this supposition. As seen in Fig. 6a, the IR spectrum of the solid product displayed characteristic absorption peaks assigned to that of DBTO2. Moreover, three new absorption bands were observed. The absorption band at 1026 cm−1 should correspond to the vibration of OO bond [19]. The two absorption bands at 819 and 776 cm−1 revealed the existence of TiOTi bond [23]. Furthermore, the Raman spectrum of the solid product gave a new peak at 996 cm−1 (Fig. 6b), further demonstrating the formation of TiO bond [24].
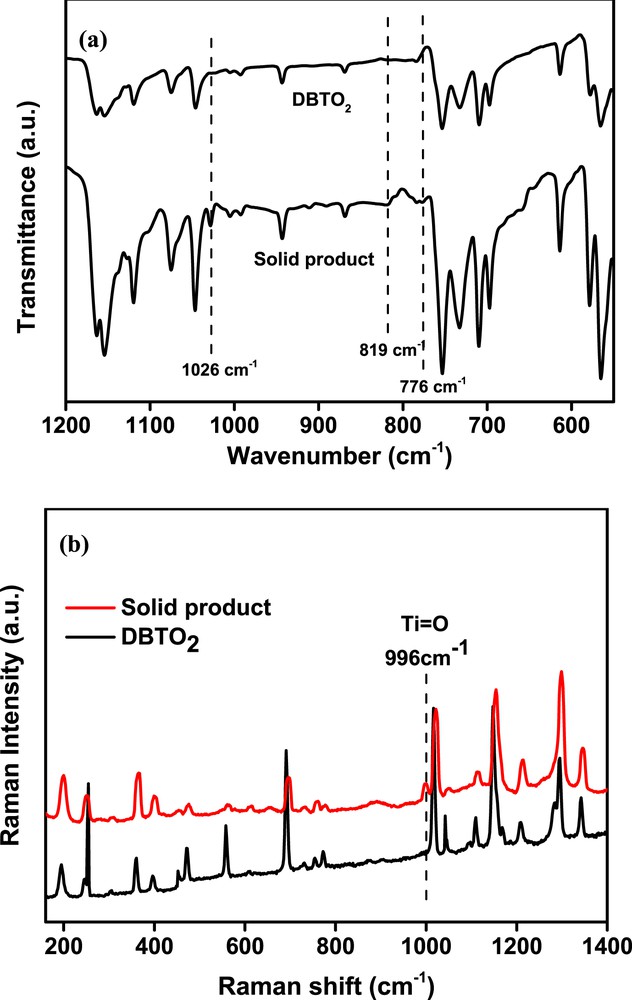
IR spectra (a) and Raman spectra (b) of DBTO2 and the solid product after reaction.
4 Conclusion
TAOTs exhibited highly efficient catalytic performance in the oxidative desulfurization of DBT. The sulfur content of 1000 ppmw in model oil over this kind of catalysts can be reduced to less than 10 ppmw in 10 min at room temperature and ambient pressure. Furthermore, the formed DBTO2 can be easily separated from the oil phase without extraction process perhaps because of the electrostatic interaction between DBTO2 and (Ti2O5)2+ species. This work will provide a basis for the design of novel ODS catalysts with high desulfurization efficiency.
Acknowledgments
We thank the financial support from the Fundamental Research Funds for the Central Universities (Grant No. HIT. NSRIF. 2015046), China; open funding from the State Key Laboratory of Inorganic Synthesis and Preparative Chemistry, Jilin University, China; the Key Laboratory of Functional Inorganic Material Chemistry (Heilongjiang University), Ministry of Education, China.